and Alessandra Iannelli1
(1)
Health Physics Department, “IRCCS Casa Sollievo della Sofferenza”, Viale Cappuccini, 71013 San Giovanni Rotondo, (Fg), Italy
High-field magnetic resonance (MR), originally developed in the framework of spectroscopy and functional neuroradiology, has become to our days an important diagnostic tool not only in research but also in advanced clinical practice.
High magnetic fields afford a better signal/noise ratio (SNR) and consequently better spatial resolution in a shorter time of acquisition, even though the diagnostic outcome is then subject to the dependence on the magnetic field of other factors that variously contribute to image quality.
The rationale for the utilization of high magnetic fields in MR diagnostic imaging is obvious: the distribution of the population into two spin levels is statistically determined.

An increase in B o values results in an inversion of the states of the two populations and therefore in a stronger MR signal.
In fact, the signal intensity is proportional to the square of the static magnetic field, since
S ≅ (N spin ⋅ V spin) where the number of N spin and V spin and the voltage induced by each spin both depend linearly on B o [1, 2]. However, while the signal is proportional to the square of the static field B o , the noise is proportional to B o , and therefore from 1.5 T to 3.0 T, the SNR doubles. This allows to obtain an acceptable image quality even with increased spatial resolution or reduced time of acquisition.
The overall MR diagnostic outcome is always a compromise between field strength and other parameter choices such as gradients used for slice selection, radio frequency, and acquisition timing.
For instance, achieving greater spatial resolution while minimizing undesired partial volume effects requires increasing gradient steepness G z and reducing the frequency bandwidth Δω in the decoding readout phase, since slice thickness is defined as
[2].

In any case, shimming requires a very strong field homogeneity. For instance, nowadays 3 Tesla machines used routinely achieve usually field homogeneity of less than 1 1.5 ppm in a spherical volume of 45 cm and of less than 0.005 ppm in a spherical volume of 10 cm, with a peak gradient ramp of 30÷50 mT/m and slew rates of about 150÷200 T/m/s.
Another key principle is that the resonance frequency ω = γ × B o depends on the static magnetic field. It therefore is 43 MHz at 1.0 T and 128 MHz at 3.0 T, resulting in greater radio frequency (RF) absorption by biological tissues, whose conductivity increases with frequency. This poses problems when designing coils suitable for the greater power applied as well as for patient safety, as increased tissue temperature is one of the risks associated with RF electromagnetic fields [3, 4].
Another important note is about susceptibility. Increased susceptibility of tissues exposed to higher magnetic field can result in local inhomogeneities that cannot be corrected and eventually present as artifacts. This effect has been exploited in functional MR BOLD studies, which are based on the changes in blood oxygenation generated by magnetic susceptibility inhomogeneities [2, 4, 5].
Notwithstanding technical and cost problems, high-field MR offers very significant advantages, and several human imaging studies at 340 MHz have demonstrated that safety margins exist above 3 and 4 Tesla [6]. More recent studies exploring biological effects on vital signs and cognitive ability suggest no risk even up to 9.4 Tesla➔2010 [7].
The growing interest in 3 T and higher magnetic fields has brought the safety issues back to the forefront.
When planning the installation of a high-field MR unit, the strength of the static magnetic field is one of the major problems to be addressed by those responsible for safety and technicians alike. In fact, this is but one, albeit the most apparent, element to be considered when assessing the associated risks and benefits. Patients in the tunnel of a high-strength imager are exposed to a magnetic field many thousands of times greater than the earth’s, even though no special patient or operator safety precautions are required compared with low or medium magnetic fields. However, during scanning, patients are also exposed to gradient switch-on and to RF impulses for signal decoding and spin excitation, both of which are related to the intensity of the static magnetic field and carry different though acceptable patient risk given the obviously beneficial result.
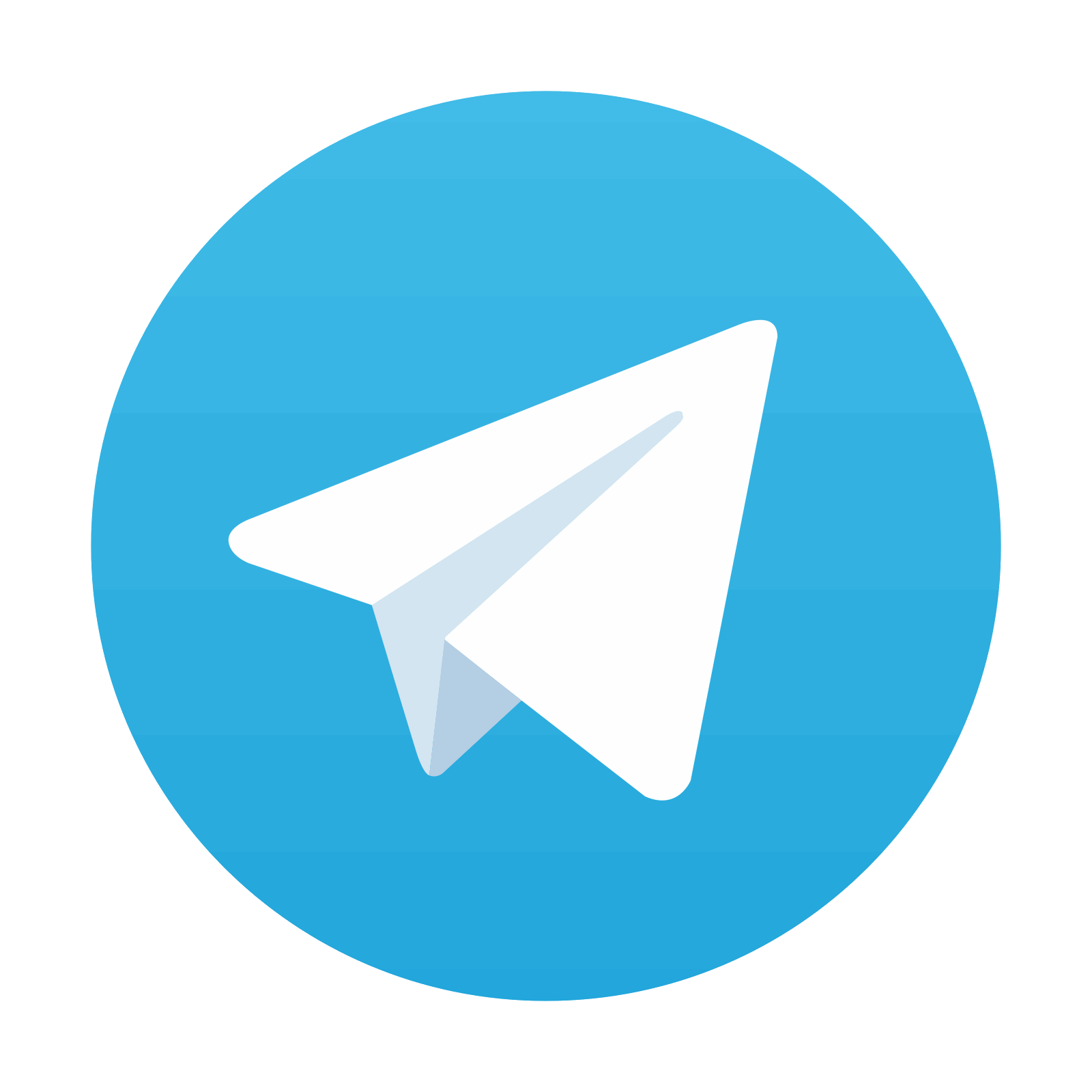
Stay updated, free articles. Join our Telegram channel

Full access? Get Clinical Tree
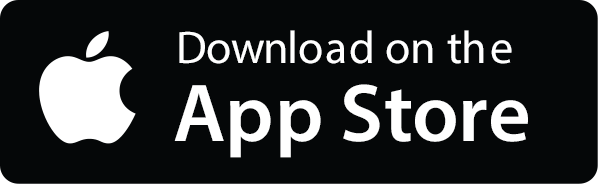
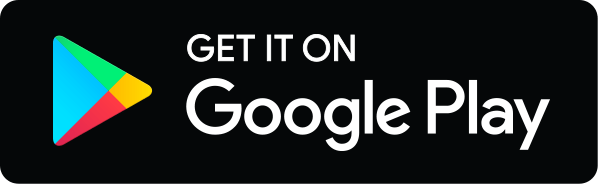