Fig. 14.1
SE T1: comparison between images acquired in the same patient at 3.0 T (Allegra, Siemens) (a left) and 1.5 T (Vision, Siemens) (b right). The thin extra-axial right frontal blood collection, indicating a subacute subdural hematoma, is appreciated in both sequences
As far as it regards T2-weighted images, the greater spatial resolution, resulting in better anatomical detail and contrast due to the greater SNR, allows thicker slices and broader matrices to be used and small lesions such as contusions or DAI to be appreciated. A further advantage of high-field T2-weighted sequences is their ability to depict very small deposits of deoxy- or methemoglobin as markedly hypointense areas by virtue of magnetic susceptibility effects, which are amplified by the high field [17, 23].
High-field GE T2* sequences are more sensitive to the magnetic susceptibility of hemoglobin degradation products and are thus valuable for detecting DAI. A correlation between the number of blood-containing lesions, DAI lesions identified on T2*-weighted sequences, and GCS score was confirmed by Scheid et al. [14], whereas no relationship was documented between focal changes appreciable in T2*-weighted sequences and Glasgow Outcome Scale (GOS) scores [24], suggesting that lesion load may not predict prognosis in these patients (Fig. 14.2).
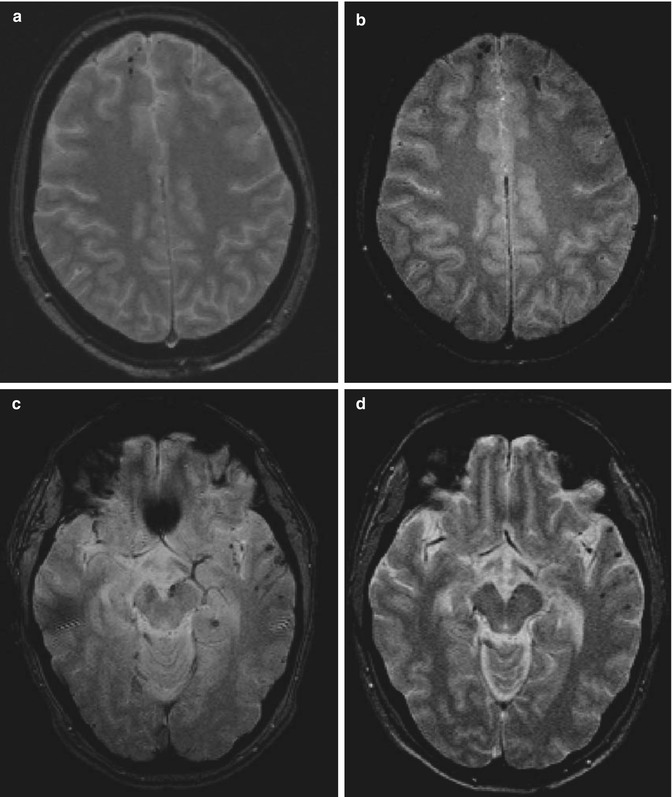
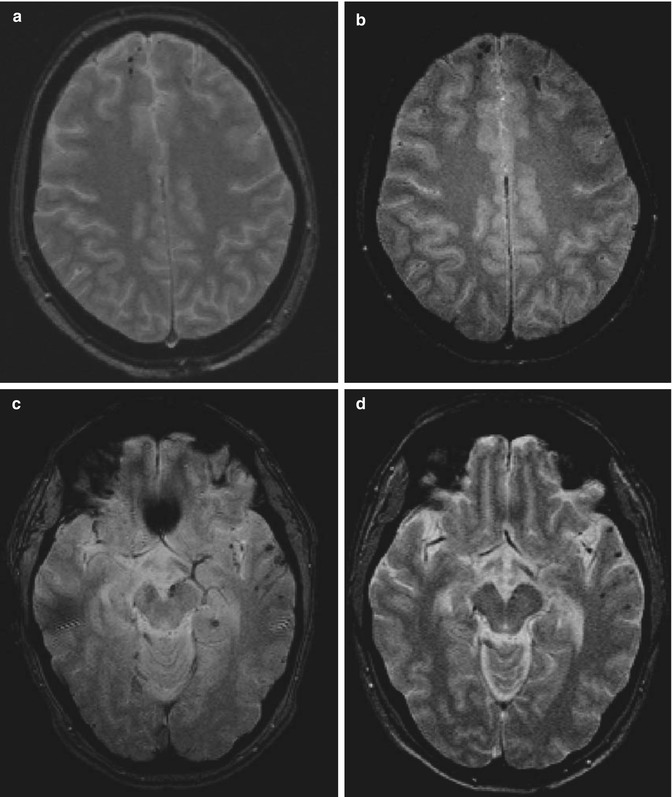
Fig. 14.2
GE T2*: comparison between images acquired in the same patient at 3.0 T (Allegra, Siemens) (a, c left) and1.5 T (Vision, Siemens) (b, d right). Bilateral frontal subcortical (a, b) and left temporal and mesencephalic (c, d) small focal DAI lesions are more numerous and better visualized at 3.0 T
EPI sequences are also more sensitive at 3.0 T than 1.5 T, to the detriment of image geometry. On EPI images, distortion is approximately 30 % greater at 3.0 T than 1.5 T but can be reduced to about 1 % using SSFSE [25]. Furthermore, also the accurate study of intracranial vessels, which is especially useful in patients with TBI and clinically suspected vessel dissection, carotid-cavernous fistula, or dural venous sinus thrombosis, benefits from high-field imaging [26].
Ultrahigh-field systems (>3 T), which are currently under development and validation for clinical imaging, are expected to improve the in vivo imaging of TBI lesions, especially DAI. An improvement in image quality has already been shown at ultrahigh field in ex vivo studies [27]. A recently published in vivo studies at 7 T in a small patient cohort showed for the first time that ultrahigh-field imaging has a much higher potential than assumed in the depiction of small hemorrhagic DAI compared to 3 T [27].
14.4 Advanced High-Field Techniques in TBI
It is well established that standard MRI sequences underestimate traumatic brain injury [14, 28–31]. On the other hand, more advanced MRI techniques such as diffusion tensor imaging (DTI), magnetic resonance spectroscopy (MRS), and functional MRI (fMRI) are available at high fields. DTI is able to map fiber tracts and locate pathway disruption; MRS provides information on the altered metabolism, and fMRI can reveal, apart from functional activations, connectivity maps at rest (absence of a specific task). Thus, the combination of these techniques can provide important information regarding the relationship between the structural and functional brain architectures which provide a multivariate prognostic information. In fact, these new MRI techniques have been applied to TBI to gain insights into the mechanisms underlying the patient’s clinical condition, predict its evolution and thus prognosis, and optimize therapeutic strategies [32–39].
14.4.1 Diffusion Tensor Imaging
DTI is a diffusion-weighted imaging (DWI) technique that paved the way to new possibilities for investigating white matter in vivo as it provides information about white matter anatomy that is not available through any other methods.
DTI provides two essential types of information: a quantitative estimate of anisotropy and its spatial orientation. Tractography uses these microscopic data to track macroscopic axon fibers, and it is capable of noninvasive in vivo imaging of these structures [18, 19, 40]. In DAI, the white matter fibers are typically interrupted; measuring white matter anisotropy with DTI allows the tissue damage to be quantified [41] (Fig. 14.3).
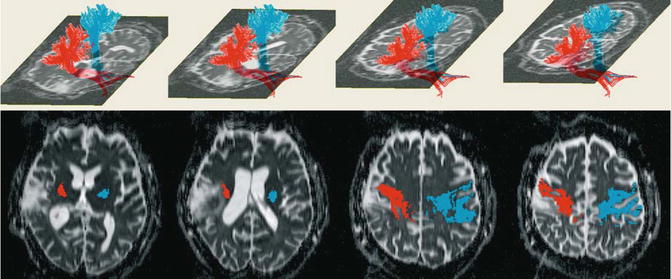
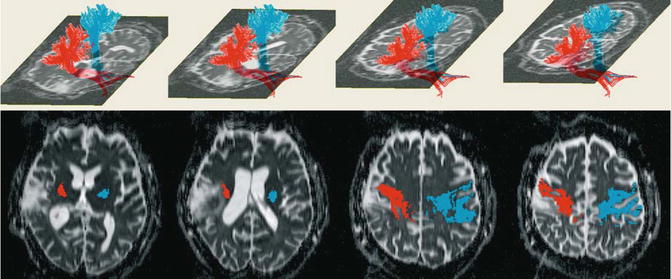
Fig. 14.3
Tractography at 3.0 T (Allegra, Siemens): visualization of motor areas and pyramidal fibers in an extensive right frontal contusion. The right primary motor area and pyramidal fibers are depicted less clearly than in the contralateral area
Based on these considerations, Huisman et al. [35] proved that DTI can detect structural white matter changes and that these changes correlate with clinical parameters such as GCS score in the acute phase and at discharge. On the other hand, Zheng et al. [42] demonstrated the superior performance of DWI in identifying DAI compared with standard (T2-, T2*-weighted, FLAIR) sequences, but did not address its clinical relevance and thus the potential correlations with prognosis.
The technical characteristics of high-field MR have enhanced the quality of DTI data, particularly the spatial resolution, the spatial deformation induced by magnetic field inhomogeneities, and image SNR, thus improving the accuracy of nerve fiber tracking on mild, moderate, and severe TBI. Furthermore, the advantages of applying DTI to patients with head trauma are:
An ability to gain information on the microscopic brain damage responsible, alone or in association with the macroscopic damage, for the patient’s clinical state.
An ability to document and quantify the brain plasticity phenomena underpinning clinical recovery, which may be enhanced using pharmacological and rehabilitation therapies.
The possibility of assessing the therapeutic response even in those patients on whom clinical studies do not provide adequate information; moreover, the quantitative data offered by DTI are not influenced by potential CNS side effects of drug therapy or invasive procedures (e.g., intubation).
The possibility of using DTI data to predict outcome in trauma patients, since neither standard MR findings nor clinical parameters, such as GCS scores, can predict their future clinical condition.
The potentiality of DTI to map and correlate the disruption in fiber tracts to clinical outcomes led researchers to adopt this technique for the quantification of the primary and secondary damage in DOC patients [43]. Recently, a large literature focused on this topic, i.e., to identify by DTI the different disorder of consciousness [e.g., vegetative state (VS) vs. minimally conscious state (MCS)] [44] or to correlate DTI findings with injury severity and clinical outcome [45–49].
Actually, the use of DTI to determine DOC severity, to aid diagnosis, and to ascertain prognostic outcomes is limited to the research setting. Despite this, DTI has proved a powerful tool as it grants insight into the pathogenesis and specific WM abnormalities underlying different DOC states, casting light on the neural basis of consciousness and the clinical features associated with DOC.
14.4.2 MR Spectroscopy
MR spectroscopy is a molecular-based neuroimaging technique that reflects the intracellular metabolic status that can be influenced by the presence of a microscopic injury.
The advantage of using 3.0 T MRS consists of an enhancement of the chemical shift with better separation of the metabolite peaks and high SNR [50]. MRS can document a reduction in the N-acetylaspartate (NAA) peak, a neuronal and axonal marker, and in ostensibly normal white matter free of macroscopic focal changes weeks or months after the trauma, and the extent of this reduction significantly correlates with scores on prognostic measures like GOS and Disability Rating Scale (DRS) [36, 37, 50]. Concomitant elevation of the levels of other metabolites, such as myo-inositol (Ins) and choline (Cho), is to be ascribed to glial proliferation or an inflammatory process [51–53]. Studies of white matter devoid of focal changes have demonstrated that the levels of these metabolites can eventually revert to normal [52] as well as a progressive reduction in NAA associated with increased Cho [51] or decreased Ins peaks [36]. Shutter et al. [37] studied a group of trauma patients in the subacute phase who underwent MRS within a week of the trauma to detect metabolic changes that could predict clinical outcome. Elevation of metabolite levels such as glutamate/glutamine (Glx) and Cho in apparently normal white and gray matter was found to be highly predictive of long-term adverse outcome with an accuracy of 89 % (Fig. 14.4).
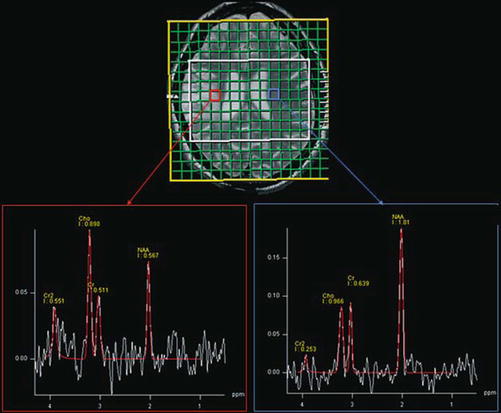
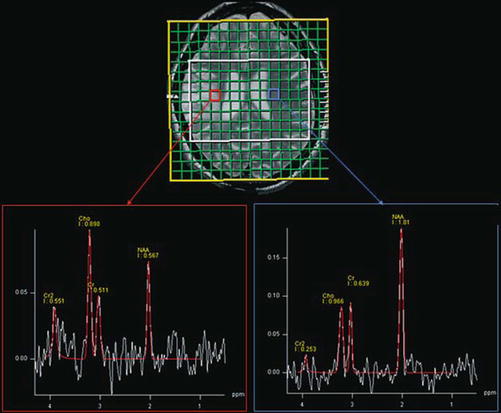
Fig. 14.4
Magnetic resonance spectroscopy with TE = 135 ms. The left spectrum was obtained from a volume of interest located in the right corona radiata, showing reduced NAA/Cr ratio reflecting neuronal loss. The right spectrum obtained from a VOI positioned contralateral to the lesion is normal
14.4.3 Functional MRI
fMRI studies typically measure signal changes induced in the blood volume, flow, and oxygenation when the normal/patient is performing a given task. The resulting acquired signal is hemodynamically coupled to the actual neural activity. In the past decades, fMRI has been used to identify regions specialized in cognitive and/or motor tasks, but more recently, the interest shifted toward a novel fMRI approach, i.e., functional connectivity MRI (fcMRI), which focuses on characterization of the brain’s intrinsic functional architecture especially at rest (absence of a specific task) (RS-fMRI) [54]. Therefore, high-field MR, one of whose advantages is a high sensitivity to the BOLD signal, could find applications in the study of the phases of motor and cognitive recovery after TBI, as suggested in the literature [38, 39, 55–57]. In particular, the degree of activation of specific brain areas at fMRI could provide an index of hemodynamic and functional activity in ostensibly healthy tissue in patients with DAI. Longitudinal variations of this index in conjunction with structural parameters and clinical examination could thus assume a prognostic value.
Typically, in task-based fMRI, data are collected with blocked design experiments in which the contrast between two conditions is evaluated in the presence of specific tasks [38, 39, 55–57]. At rest, on the other hand, participants do not perform any active task and are simply instructed to remain still either fixating or with eyes closed.
In a recent study [38], the observed cognitive and behavioral recovery significantly correlated with connectivity changes of resting-state networks (RSNs) in a group of severe TBI patients after a period of intensive neurorehabilitation. In particular, the cognitive recovery was more consistent in patients exhibiting the strongest changes in DMN connectivity (Fig.14.5).
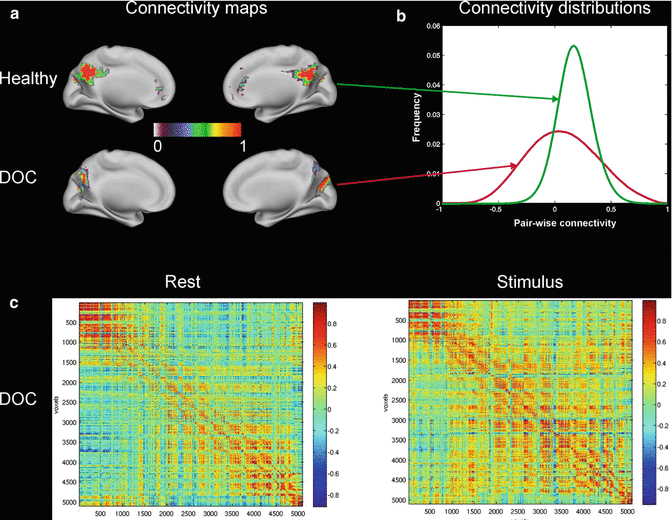
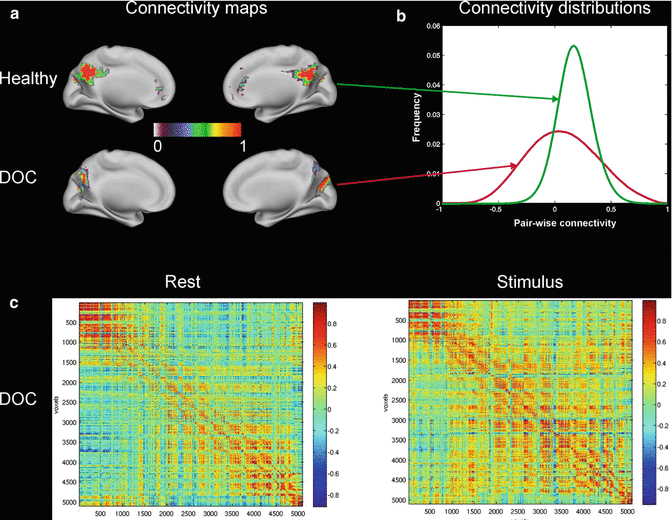
Fig. 14.5
Functional connectivity in resting DOC patients. (a) Connectivity maps in one representative DOC patient and in a healthy subject. Compared to the healthy subject, the DOC patient shows a pattern of increasing disruption in the connectivity maps of the default mode network. (b) The distribution of the overall connectivity values at rest shows that the distribution of connectivity in the DOC case shows greater spread than in a healthy subject. In the healthy subjects, the connectivity is stronger on average and also tightly centered around its mean value, i.e., the connectivity dispersion is smaller. (c) The cross-correlation matrix estimated at rest before (REST) or during an auditory stimulation (STIM) in DOC patient. A 60 % increase in the connectivity can be noted between the two conditions
Resting-state connectivity studies are appealing in DOC patients since they do not require the ability to participate in an active task in the scanner and it has been proposed as a prognostic tool in comatose patients [58–60]. The main advantage is that the identified functional systems (or networks) provide a wide coverage of several functional domains which can be assessed in a single acquisition session [54]. Therefore, there has been renewed interest, throughout the past decade, in the use of functional neuroimaging to distinguish between patients in vegetative state and individuals who are in a minimally conscious state or who exhibit locked-in syndrome [61, 62].
A recent study [59] describes the possibility to extract, from functional connectivity data at rest and during an auditory emotional stimulation, parameters able to characterize important global and local connectivity features, such as the degree of clustering, mean path length, and modularity, in DOC patients and characterize the clinical profile and diagnosis (Fig. 14.6). These results and the existing literature indicate the functional neuroimaging holds considerable promise for indicating valid biomarkers for the recovery and diagnosis in TBI patients. Further, it could generate important insight on the neural basis of consciousness and on the target for therapeutic and neurorehabilitative intervention.
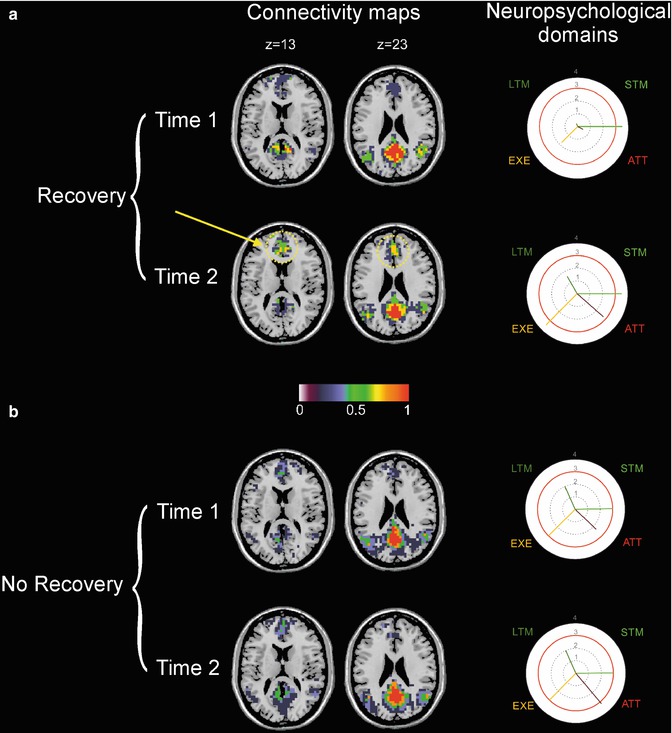
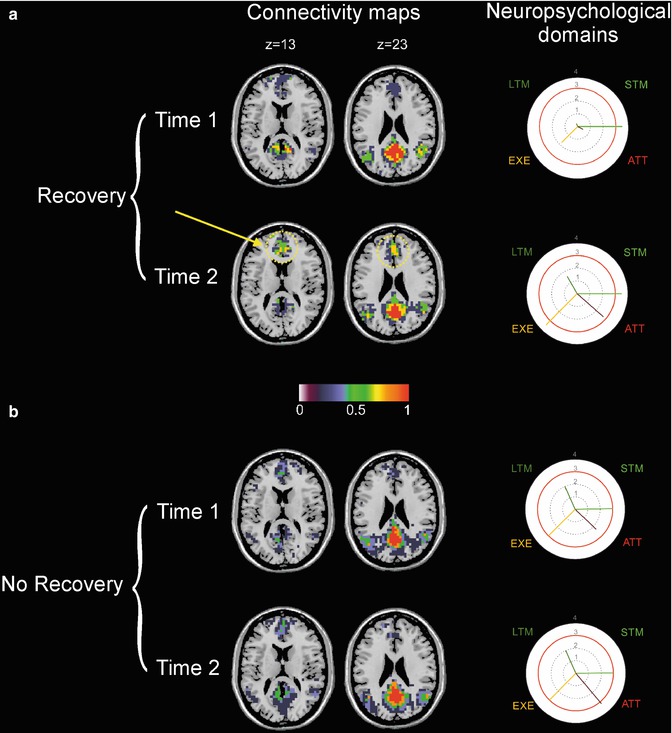
Fig. 14.6
Connectivity maps and neuropsychological domains of recovery and no recovery groups of acquired brain injury patients over time. (a) For the recovery group, the left/right angular gyrus, the precuneus, and regions of the ventromedial prefrontal cortex (vMPFC) show stable connections over time with the posterior cingulate cortex (PCC). The consistency of connections between time 1 and time 2 of the vMPFC (yellow-dotted circle) increased from approximately 30 % up to 70 %. Recovery group shows a statistically significant improvement of all neuropsychological domains. (b) The consistency maps of no recovery group show an involvement of the main nodes of the default mode network with a stable consistency of approximately 30 % at both times. No significant changes are observed between T1 and T2 in the neuropsychological domains. Long-term memory (LTM, green), executive functions (EXE, yellow), and attention (ATT, red)
14.4.4 Conclusions and Future Prospective
In conclusion, high-field MR has the potential to enhance the accuracy of morphostructural, metabolic, and functional studies of the brain parenchyma in trauma patients, advancing the knowledge of the relationships between changes in nerve fiber ultrastructure and clinical symptoms and allowing the quantification of the brain plasticity phenomena that follow spontaneous clinical recovery or administration of pharmacological and rehabilitation therapies.
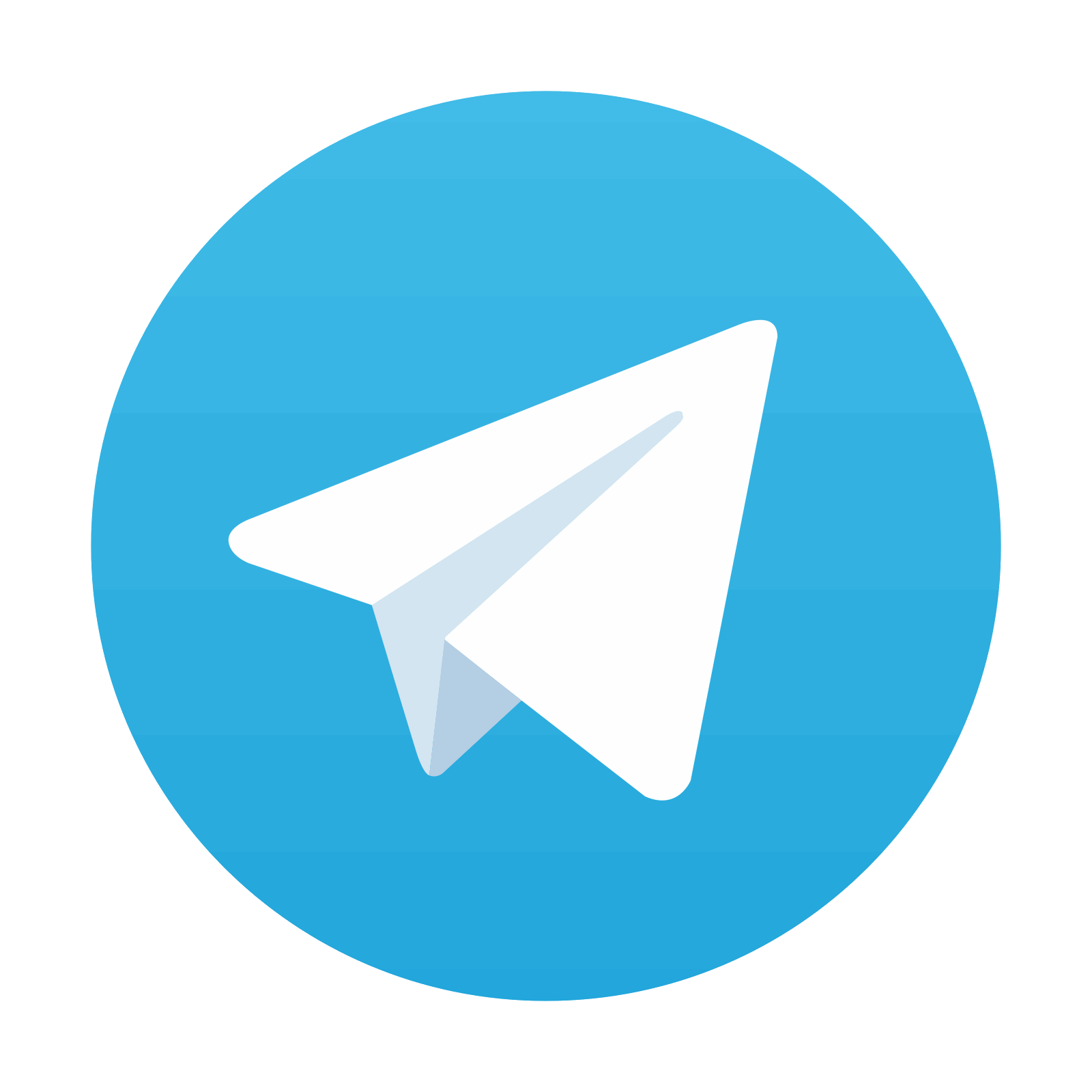
Stay updated, free articles. Join our Telegram channel

Full access? Get Clinical Tree
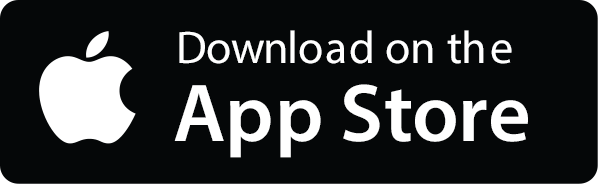
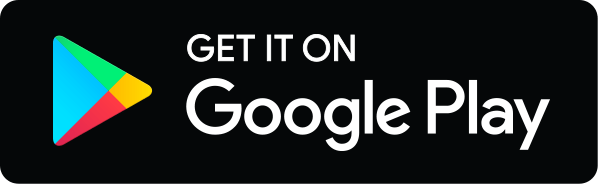