Chapter 12
Image Receptors
• Explain how the latent image is formed.
• Describe film characteristics, including speed, contrast, latitude, and spectral sensitivity.
• Describe the purpose and function of intensifying screens.
• Explain how screens can be characterized based on the type of phosphor, spectral emission, and screen speed.
• Describe factors that affect screen speed.
• State the automatic film processing stages and their function.
• Discuss the purpose of replenishment, recirculation, and temperature control during automatic film processing.
• Identify important quality control measures to ensure good radiographic quality.
• State the importance of and methods for silver recovery.
• Describe the design of cassette-based detectors.
• Describe the design of cassetteless detectors.
• Explain the process of image acquisition using cassette-based detectors.
• Explain the process of image acquisition using the three general types of cassetteless detectors.
• Explain the process of image extraction and processing for cassette-based and cassetteless systems.
• Describe digital image display and postprocessing functions.
• Explain the use of exposure indicators for cassette-based systems and dose-area product for cassetteless systems.
• Correctly identify the role of kVp, mAs, and geometric factors with digital systems.
• Identify quality control tests and test patterns used with digital systems.
• Describe the Picture Archiving and Communication System, including its role, principal systems, and challenges.
detective quantum efficiency (DQE)
Digital Imaging and Communications in Medicine (DICOM)
modulation transfer function (MTF)
photostimulable phosphor (PSP) plate
Picture Archiving and Communication System (PACS)
Radiographic Film
Film Construction
Radiographic film acquires the image and must then be chemically processed before it is visible. As a result, film serves as the medium for image acquisition, processing, and display. Several types of radiographic film are still used in medical imaging departments. Depending on the specific application, film manufacturers produce film in a variety of sizes ranging from 20 × 25 cm (8 × 10 inches) to 35 × 43 cm (14 × 17 inches). The composition of film can be described in layers (Box 12-1). The most important layer for creating the image is the emulsion layer. The emulsion layer is the radiation-sensitive and light-sensitive layer of the film. The emulsion of film consists of silver halide crystals suspended in gelatin. Silver halide is the material that is sensitive to radiation and light. The emulsion layer is fairly fragile and must have a layer composed of a polyester base so that the film can be handled and processed, yet remain physically strong after processing. Most film used in radiographic procedures has a blue dye or tint added to the base layer to decrease eye strain when viewed on a view (illuminator) box.
Latent Image Formation
Physical imperfections in the silver halide crystals are the site of the latent image formation and are described in detail in Box 12-2.
Several sensitivity specks with many silver ions attracted to them become latent image centers. These latent image centers appear as radiographic density on the manifest image after processing. It is believed that for a latent image center to appear, it must contain at least three sensitivity specks that have at least three silver atoms each. With more exposure to the film, more metallic silver is visualized as radiographic density.
Film Characteristics
Current manufacturers of medical imaging film offer a wide variety of films. These differ not only in size and general type, but also in film speed, film contrast, exposure latitude, and spectral sensitivity.
Film Speed
Film Contrast and Film Latitude
Film contrast refers to the ability of radiographic film to provide a certain level of image contrast. High-contrast film accentuates more black and white areas, whereas low-contrast film primarily shows shades of gray. As discussed in Chapter 9, film latitude is closely related to film contrast. The latitude of film affects the range of radiation exposures that can provide diagnostic optical densities. Films manufactured to display higher contrast have a narrow exposure latitude compared with low-contrast films having a wider exposure latitude.
Intensifying Screen Characteristics
As with radiographic film, the construction of screens can be described in layers (Box 12-3). The phosphor layer, or active layer, is the most important screen component because it contains the phosphor material that absorbs the transmitted x-rays and converts them to visible light. The most common phosphor materials consist of chemical compounds of elements from the rare earth group of elements. Rare earth elements are those that range in atomic number from 57 to 71 on the periodic table of the elements; they are referred to as rare earth elements because they are relatively difficult and expensive to extract from the earth.
Screen Speed
Several factors affect how fast or slow an intensifying screen is, including absorption efficiency, conversion efficiency, thickness of the phosphor layer, and size of the phosphor crystal (Table 12-1). The presence of a reflecting layer, an absorbing layer, or dye in the phosphor layer also affects screen speed.
TABLE 12-1
Factors Affecting Screen Speed, Recorded Detail, and Patient Dose
Factor | Screen Speed | Recorded Detail | Patient Dose |
Phosphor layer thickness | |||
Phosphor crystal size | |||
Reflecting layer | Increased | Decreased | Decreased |
Absorbing layer | Decreased | Increased | Increased |
Dye in phosphor layer | Decreased | Increased | Increased |
The final factors that affect screen speed are the presence or absence of a reflecting layer, a light-absorbing layer, or light-absorbing dyes in the phosphor layer. A reflecting layer is used to increase screen speed by reflecting light back toward the film (Figure 12-1). A light-absorbing layer or light-absorbing dyes present in the phosphor layer are used to decrease screen speed by absorbing light that would otherwise reach and expose the film.
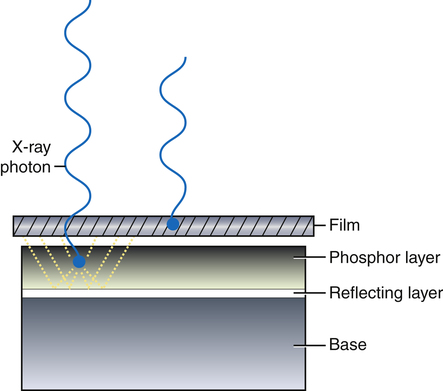
The reflecting layer redirects the visible light emitted by the screen phosphor toward the film emulsion to increase screen speed.
Screen Maintenance
The film-screen contact test is easily accomplished, but it requires a special wire mesh test tool. The wire mesh tool is placed on the cassette in question and radiographed with an appropriate technique. The resultant radiograph (Figure 12-2) is then viewed from a distance of approximately 6 feet to determine any areas of unsharpness, which indicate poor recorded detail. Areas of poor contact appear darker than areas of good contact because of the increased spreading of the light photons.
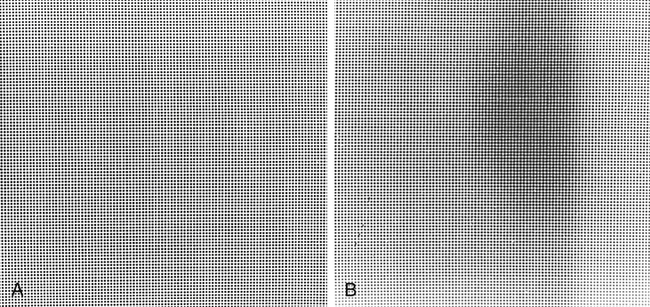
Image produced with a wire mesh test tool. A, Proper film-screen contact. B, Poor film-screen contact. (A, courtesy Fluke Biomedical; B, courtesy Barbara Smith Pruner.)
Automatic Film Processing
Components
An automatic film processor (Figure 12-3) is a device that encompasses chemical tanks, a roller transport system, and a dryer system for the processing of radiographic film. The processing of a radiograph occurs in four stages: developing, fixing, washing, and drying. Each stage has its specific function and processing method (Table 12-2).
TABLE 12-2
Automatic Film Processing Stages |
Washing: Removes fixing solution from the surface of the film. |
Drying: Removes 85%-90% of the moisture from the film. |