(1)
Section of Nuclear Medicine, Department of Radiology, Memorial Sloan-Kettering Cancer Center, New York, NY, USA
(2)
Department of Radiology, Memorial Sloan-Kettering Cancer Center, New York, NY, USA
Abstract
The average age of a cancer patient is 67. Sexagenarians with cancer are likely to have comorbidities at the time of diagnosis. Cancer patients > 55 years old have an average of ∼ 2.9 comorbidities, while cancer patients older than 75 have an average of 4.2 comorbidities. The likelihood of coronary artery disease as an etiology of this comorbidity increases with the age of the patient. Cardiovascular comorbidity is present in ∼ 20% of patients with neoplasm. These comorbidities increase the risk of a serious cardiovascular event during treatment. An additional risk factor is limited work capacity. If patients cannot perform 4 METS of work, their all cause mortality is increased. Patients with cancer and known cardiovascular comorbidity or risk factors such as diabetes, hypertension, smoking history, or limited work capacity should have medical clearance prior to invasive diagnostic procedures, major surgery, mediastinal radiation, and/or potentially cardiotoxic chemotherapy. Medical clearance should include a detailed cardiovascular history and physical examination. This information will permit calculation of a clinical score (such as POSSUM) to define the risk of adverse events as a result of a major surgical procedure, blood tests to determine hematologic and renal status, and if necessary, assessment of the patients work capacity. Stress testing with imaging should be done in patients with an intermediate risk of coronary heart disease and considered in patients with limited work capacity or advanced age. In selected patients, coronary CT angiography or coronary calcium score may be a suitable evaluation. In patients with cancer of the esophagus, breast, lung, melanoma, or lymphoma, chest-CT and PET/CT studies should be carefully evaluated to detect possible pericardial or myocardial involvement.
Chemotherapy may cause myocardial ischemia due to coronary spasm and decreased ventricular function due to irreversible or reversible myocardial damage, as well as repolarization abnormalities, which may result in fatal arrhythmia. Radiotherapy may accelerate the development of atherosclerosis of vessels in the radiation field and cause irreversible damage to myocardium in the radiation field. Myocardial perfusion imaging is useful to detect regions of acute ischemia or scar induced by therapy, while blood pool imaging is useful for serial monitoring of ventricular function.
Keywords
Heart imaging in the cancer patientCardiovascular comorbidities in the cancer patientCardiotoxic chemotherapyStress testing in cancer patientsHeart disease in cancer patientsCancer coexisting with cardiovascular diseaseDetermination of Preoperative Risk
A major role of preoperative cardiac assessment is identifying patients with severe ischemia, extensive myocardial scar, and reduced ventricular function, who may be at increased risk of a cardiac event in the course of therapy for their cancer. Pretreatment evaluation is particularly important in anticipation of lengthy surgical procedures often required for tumor resection. Several clinical factors are recognized as important prognostic indicators, including the work capacity of the patient. The unit of work capacity is the metabolic equivalent of task (MET). A MET is the ratio of the work required for the task compared to the resting metabolic rate [1]. One MET is equivalent to 3.5 ml/kg/min, the oxygen cost of sitting quietly. The ability to climb two flights of stairs or perform light to moderate housework is equivalent to about 4 METS of work. Inability to perform 4 METS of work is associated with increased length of stay and a higher rate of complications following major surgery [2, 3]. Additional risk factors include advanced age, presence of severe coronary disease, history of recent heart failure, complexity of the surgical procedure (including duration of anesthesia, likelihood of large fluid shifts in the course of the procedure), and likelihood of pain or other severe discomfort in the immediate postoperative and early recovery interval (up to 7 days). The surgical procedure often causes release of chemokines and platelet activation, increasing the vulnerability of the patient to a cardiovascular event.
There are several clinical scoring systems used to quantify the perioperative risk in these patients. A time-honored system developed by the American Society of Anesthesiologists in the 1940s employs readily available clinical and historical observations (Table 29.1). Patients in categories 3, 4, and 5 require more intensive monitoring, fluid replacement, and selective use of drugs during surgery. Patients with higher scores are at increased risk of perioperative events. While this system is useful in patients who are severely ill, there is limited ability to distinguish risk in patients with less severe disease.
Table 29.1
American Society of Anesthesiologists classification
Condition | 48 h Mortality |
---|---|
1. Normal healthy patient | 0.07% |
2. Mild systemic disease | 0.24% |
3. Severe systemic disease that limits activity | 1.40% |
4. Incapacitating systemic disease that is a constant threat to life | 7.50% |
5. Moribund patient not expected to survive >24 h with or without operation | 34% |
6. Declared brain dead whose organs are being removed for donor purposes |
There are several other clinical scoring systems. One system in wide use, the revised cardiac risk index (RCRI) proposed by Lee [4], considers six factors (Table 29.2). Each parameter in the scoring system gets one point, and the total number of points is summed to result in the score. The rate of cardiac death, nonfatal MI, and nonfatal cardiac arrest in the perioperative interval is as follows [5]: no risk factors, 0.4%; one risk factor, 1.0%; two risk factors, 2.4%; and three or more risk factors, 5.4%. While this system is an improvement over the anesthesiologists scoring system in patients with less severe disease, it does not provide a detailed enough score for defining the relative risk of surgery.
Table 29.2
Revised cardiovascular risk index (RCRI)
High-risk surgery (suprainguinal vascular, intraperitoneal, and/or intrathoracic surgery) |
History of MI or evidence of current ischemic heart disease |
History of heart failure |
History of cerebrovascular disease |
Diabetes mellitus requiring insulin |
Preoperative creatinine >2.0 mg/dL (177 umol/L) |
Another clinical scoring system, POSSUM (Physiologic and Operative Severity Score) and P-POSSUM (POSSUM predicted mortality) considers multiple factors, including age, presence of cardiac signs, dyspnea, pulse rate, Glasgow Coma Score, hemoglobin, white blood cell count, BUN, serum sodium and potassium, and abnormal rhythm on EKG (including atrial fibrillation). To determine risk, P-POSSUM calculators are available on the Internet [6]. The P-POSSUM calculator considers the type of surgery score and also considers the severity of the surgery (ranging from minor to complex major surgery), number of procedures, anticipated blood loss, peritoneal soiling, presence of metastases, and whether the surgery is elective, urgent, or emergent. A comprehensive discussion of patient evaluation is contained in the updated ACC/AHA guide on perioperative cardiovascular evaluation for noncardiac surgery [7].
Management of Cardiac Risk in Noncardiac Surgery
Background
About 200 million noncardiac surgical procedures are performed worldwide each year, with about one quarter of the procedures performed on patients over 65 years of age [8]. In the USA, about 20 million surgical procedures are performed under general anesthesia each year [9]. Of these patients, about 1 million will have a perioperative cardiac complication, including 50,000 with a myocardial infarction [10]. Approximately 20,000 patients with perioperative myocardial infarction will have a fatal outcome over the next 6 months (0.01% of all operations but 40% of those with infarction) [11]. Aging of the population suggests that a comorbidity of coronary disease will become more common. By the year 2055, > 20% of the US population will be over the age of 65, and World Health Organization estimates that 25% of the world’s population will be >65 in the second half of the twenty-first century. Patients over age 65 will account for approximately 40% of all surgical procedures [5]. The Surveillance, Epidemiology, and End Results database (SEER) statistics indicate that the median age of cancer patients is 67 years old. The lifetime risk of developing cancer is approximately 1 in 2 for men and 1 in 3 for women. In 2010, about 1,529,560 new cases of cancer were diagnosed in the USA, and about 11,400,000 Americans had a history of cancer [12]. The risk of multiple primary cancers ranges from 1% to 16%, depending on the type of cancer [13]. On the other hand, the American Heart Association reports that 16.3 million people carry a diagnosis of coronary artery disease. In patients 60–79-year age group, the disease is present in 22.8% of males and 13.8% of women [14]. As a result, it is likely that patients with cancer will have coronary heart disease as a comorbidity.
In the USA, approximately 5.3% of patients with coronary disease, but without cancer (about 935,000 people), experienced an acute myocardial infarction in 2010. The common concurrence of cancer and coronary disease raises the likelihood that a patient with cancer may have a cardiac event in the course of therapy.
Events
Patients with a history of coronary heart disease are at much higher risk for perioperative ischemic events. In the first week after surgery, ischemic episodes occur in 24–41% of these patients. The majority of these events are clinically silent [15]. Major acute clinical events (infarction, heart failure, or cardiac death) occur in approximately 5%. A perioperative myocardial infarction, even if clinically silent, is associated with a hospital mortality rate of 15–25%. Of the patients who have a perioperative infarct, 75% are nontransmural [16]. For example, a study of 283 radical cystectomy patients, average operative time 7 h, patients’ average age 70, found coexistent coronary disease in 64 patients (23%) [17]. There were 31 patients with perioperative cardiac complications including new-onset arrhythmia in 22 and myocardial infarction in 10. Thirteen of these patients (42%) had a cardiac history. In the infarct group, there was a previous cardiac history in 4. All 10 MI patients had at least one other postoperative complication, such as sepsis or a major wound issue. This added stress of the postoperative complication likely caused tachycardia and possibly increased blood pressure, increasing myocardial oxygen demands. Pain or other factors associated with the complication may have contributed to coronary artery spasm, reducing coronary blood flow. The combination of factors could result in severe prolonged ischemia and infarction. The mean interval between surgery and MI was 3.5 days. Preoperatively, 5 of the 10 MI patients had normal stress tests, and two patients with abnormal stress tests had stents placed precystectomy.
The relationship between previous cardiac stent implantation and cardiac events in patients undergoing noncardiac surgery was explored in a retrospective analysis of 1,953 patients [18]. There were no significant differences between drug-eluting and bare-metal stents (in-hospital mortality ∼0.6%, MI ∼0.9%, or postoperative ischemic cardiac events 14%). There was a significant increase in the incidence of death or MI if the interval between stent placement and surgery was <42 days (42.4%), compared to 42 days to 1 year (24.5%) or > 1 year (11.7%).
It is frequently problematic to identify perioperative myocardial infarction since patients are usually receiving analgesia and may be intubated. In addition, electrocardiographic changes may be subtle. About 50% of perioperative infarcts are clinically unrecognized [19]. To determine the true incidence of perioperative myocardial damage, a series of 65 head and neck surgery patients had troponin I measured on postoperative day 3 to detect myocardial injury. Of the 65, 16 (25%) had an elevated troponin level (>0.032 mg/mL); only 5 patients had clinically apparent infarction [20]. Myocardial damage was not related to the length of surgery (243 min in patients with elevated troponin vs 205 min, p > 0.2) but did correlate with postoperative inflammation, measured by elevated CRP. To optimize the detection of perioperative myocardial infarction, the new worldwide standard definition of acute infarction is now applied to studies. The standard utilizes an increase in cardiac enzymes (preferably troponin) to > 99th percent of the laboratories normal values, but also includes new Q waves or sudden cardiac death.
Pathophysiology of Perioperative Myocardial Infarction
To reduce the risk of perioperative cardiovascular events, it is helpful to understand the pathophysiology of ischemia and infarction in these patients. Two retrospective autopsy studies evaluated the etiology of perioperative MI: 1. Dawood et al. compared fatal perioperative MI with fatal nonoperative MI [21]. The investigators evaluated the hearts of 42 patients who had elective or emergent noncardiac surgery and died of perioperative infarction within 30 days (MI occurred within 7 days in 40 patients). History of prior MI was present in 55%. Left main disease was found in 19% and triple vessel disease in 59%. Plaque fissure, rupture, or hemorrhage was found in 55% of patients, and intraluminal thrombus was present in 28% (likely an underestimate, due to spontaneous thrombolysis). Six patients had plaque disruption in more than one coronary artery, suggesting that there is a generalized vasculitis as a component of the patients’ atherosclerosis. 2. Cohen and Aretz [22] studied the hearts of 26 cases with fatal perioperative MI. Prior myocardial scar was present in 81% (compared to a clinical history of prior MI in only 19%); left main disease was present in 23% and multivessel disease in 88%. Plaque rupture was present 46%, and intracoronary thrombus was present in 35%.
These autopsy studies confirm the importance of multivessel coronary disease and the presence of vulnerable plaque as predictors of fatal coronary events. A history of coronary disease, however, may be lacking, as demonstrated in the study by Cohen and Aretz.
An additional factor in perioperative myocardial infarction (MI) is an increase in oxygen requirement secondary to increased heart rate or blood pressure in the perioperative environment. Continuous 12-lead ST segment trend monitoring during 48–72 h following vascular surgery demonstrated an average increase heart rate of ∼ 32 beats/min, often accompanied by increases in blood pressure, resulting in multiple prolonged episodes of ST depression ischemia. The duration of ischemic episodes was fivefold longer in patients with previous MI [23, 24]. These episodes likely reflect sympathetic surges. In the perioperative environment, the patient is more “vulnerable” to a cardiovascular event due to platelet activation, systemic inflammation, and anemia or fluid overload.
Preoperative testing for patients at risk of perioperative events should identify patients at high risk for these occurrences. Investigators compared a preoperative dobutamine echocardiogram to intraoperative transesophageal echocardiography (TEE) [25] in 54 patients scheduled for major vascular surgery. Preoperative dobutamine stress tests induced new wall motion abnormalities in 17 patients. Intraoperative echocardiography detected new wall motion abnormalities in 23 patients. A perioperative ischemic or infarct event occurred in 15 patients: 10 had a dobutamine-induced wall motion abnormality and a new wall motion abnormality on intraoperative TEE; in 4 patients, an abnormality was only detected on the intraoperative TEE.
A review of stress myocardial perfusion imaging in 787 cancer patients [26] identified ischemia or scar in 36% (36% of those stressed with adenosine, 36% of those stressed with dobutamine, and 38% of those stressed with exercise). During a 3-year follow-up, 84 patients (11%) had a cardiac event (cardiac death in 51 patients, 61% of those with a cardiac event). Cardiac events occurred in 6% of patients with a normal stress MPI and in 19% of those with ischemia, scar, or both. Ischemia on MPI was associated with a 24% likelihood of a cardiovascular event, while scar was associated with a 19% likelihood. However, the overall 3-year survival in these patients was only 44%, suggesting that ischemic heart disease played a less of a role in survival than the patient’s cancer.
Perioperative and Intraoperative Management
The surgery performed on cancer patients frequently requires hours of general anesthesia and clamping and release of major vessels and may involve significant blood loss/transfusion replacement. These factors contribute to the release of inflammatory cytokines which may increase the thrombogenicity of preexisting atheroma, leading to an embolic, thrombotic, or vasospastic event. Many patients have a significant elevation of resting heart rate postoperatively, associated with release of catecholamines due to transient hypoxia, extubation, postoperative pain, increased or decreased circulating blood volume (excess or insufficient replacement of blood or fluid), and transient increase in inflammatory states due to increased production/release of tumor necrosis factor, interleukin, and CRP due to tissue injury [16]. Persistent tachycardia increases myocardial oxygen consumption, raising the likelihood that patients with moderate and severe coronary stenoses may develop ischemia. To reduce the likelihood of perioperative infarction these patients should be treated with beta blockers, with the goal of maintaining a heart rate of 60 or less and minimizing surges in blood pressure if there is no contraindication.
Approach to Evaluation of the Presurgical Patient
The initial approach is a medical or cardiology consultation to evaluate both the status of the patients and the risk of the surgical procedure. These risk factors shown in Table 29.3 are then combined with the known cardiac risk (cardiac death and nonfatal infarction) of the surgical procedure:
Table 29.3
Perioperative risk factors
Acute cardiac conditions Acute coronary syndromes Decompensated heart failure Significant arrhythmias Severe valvular disease |
Clinical risk factors History of ischemic heart disease History of compensated or previous heart failure History of cerebrovascular disease Diabetes mellitus Renal insufficiency (creatinine >2 mg/dL) |
Minor risk predictors Age > 70 Abnormal EKG Rhythm other than sinus Uncontrolled hypertension |
>5% Vascular surgery >5% (aortic, peripheral vascular surgery)
1–5% Intraperitoneal and intrathoracic surgery
Carotid endarterectomy
Head and neck surgery
Major orthopedic surgery
Prostate and surgery of the urinary bladder
<1% Endoscopy
Cataract surgery
Breast surgery
In patients with an intermediate likelihood of a perioperative cardiovascular event, based on the clinical scoring, noninvasive testing is warranted.
Patients at intermediate risk for coronary events, primarily those with a score of >1 on the revised cardiac risk index (see Table 29.2), appear to be the group with the greatest benefit from preoperative testing. In a meta-analysis of six preoperative diagnostic tests, Kertai et al. found the sensitivity of an abnormal exercise electrocardiogram, myocardial perfusion study, or dobutamine echocardiogram each to offer >74% (confidence interval >60%) prediction for perioperative events. Impaired left ventricular function had a surprisingly low sensitivity and poor positive predictive value for perioperative cardiac events [27].
Mangano and colleagues demonstrated that effective pre- and intraoperative beta blockade reduces the incidence of perioperative cardiovascular events particularly in low-risk patients and, second, that preoperative revascularization does not reduce perioperative morbidity and mortality: “Coronary revascularization prior to noncardiac surgery is only indicated in unstable patients and patients with left main disease” [28]. The reason for this observation about preoperative revascularization is likely due to the difference in etiology between infarcts occurring perioperatively and those occurring outside this environment.
In a retrospective study of 271,082 patients ≥40 years old undergoing intermediate- to high-risk noncardiac surgical procedures, 23,991 had preoperative noninvasive stress testing (including graded exercise treadmill testing, exercise or dipyridamole nuclear perfusion imaging, and stress (exercise or dobutamine) echocardiography). Patients with intermediate and high risk of cardiovascular events (using the RCRI criteria) who had stress tests had improved one year survival and reduced length of hospital stay [29]. A possible explanation for this outcome is that patients who had stress testing were more likely to be admitted to a monitored bed after surgery.
Myocardial Perfusion Imaging in Preoperative Assessment
Myocardial perfusion imaging (MPI) can be performed using single photon or positron-emitting tracers. The most common procedures employ single photon agents, which permit patients to be studied with exercise or pharmacologic stress. Exercise stress has the advantage of providing information about a patients work capacity (see above), evidence of electrocardiographic ischemia, and information about the relative distribution of myocardial perfusion at rest and stress. Exercise stress is particularly useful in patients below the age of 70, with no evidence of neuropathy, orthopedic impairment, lung disease, or impaired mental activity.
Patients who cannot exercise can be stressed with intravenous administration of vasodilators (dipyridamole, adenosine, or regadenoson) or catecholamine agonists (dobutamine). Vasodilator stress MPI has the advantages of a sensitivity equal to exercise stress for the detection and localization of ischemia and a straightforward and rapid acting means of reversing the vasodilator effects (intravenous aminophylline). A major contraindication to the use of dipyridamole or adenosine, bronchospasm, can be minimized in patients who are not actively wheezing, with inhalation of one or two puffs of albuterol before administering the vasodilator. Regadenoson, on the other hand, is a selective adenosine alpha-2 agonist. This vasodilator does not cross-react with the receptors causing bronchoconstriction, reducing the need for albuterol and reducing the likelihood of inducing bronchospasm in patients with a history of asthma.
Dobutamine stress is typically a longer procedure than vasodilator stress, often requiring the addition of atropine to achieve the desired heart rate endpoint. Like vasodilator stress, dobutamine can be reversed pharmacologically with the short-acting intravenous beta blocker esmolol. Atropine, however, has a half-life of ∼2.5 h and, in addition to dry mouth and blurred vision, may cause urinary retention. To minimize these side effects, vasodilator stress is preferred for pharmacologic stress testing.
A meta-analysis of 24 studies reporting results on 14,918 patients with known or suspected coronary disease compared the cardiac outcome of pharmacologic (dipyridamole, adenosine, or dobutamine) or exercise stress MPI [30]. Patients were followed for 12–55 months (median 22 months for pharmacologic stress and 20 months for exercise). There were 343 cardiac deaths and 216 nonfatal MIs in the pharmacologic stress group and 180 cardiac deaths and 277 nonfatal MIs in the exercise group (total event rate = 6.8%). In the pharmacologic stress group, the annualized event rate for cardiac death and MI (hard events) for patients with normal perfusion scans was 1.78%, while it was 9.98% for patients with abnormal images. In the exercise stress group, the pooled hard event rates were 0.65% for patients with normal images and 4.3% for those with abnormal images. These data suggest that both pharmacologic and exercise stress gated single photon emission computed tomography (SPECT) myocardial perfusion scans identify patients with a significant risk of cardiac events; however, even in the high-risk group, the event rate is <10%.
A report of 394 cancer patients who had preoperative single photon MPI, described normal scans in 201 patients, while 97 had ischemia, 80 had scar, and 16 had ischemia and scar [31]. Nine patients had perioperative events; all had abnormal scans: 4 scar, 3 ischemia, and 2 scar and ischemia. Similar results were found in patients undergoing surgery for peripheral vascular disease [32].
Positron emission tomography (PET) myocardial perfusion imaging has advantages over single photon studies. Spatial resolution with PET is ∼5 mm versus ∼11 mm for SPECT. Robust attenuation correction minimizes false positive scans due to breast or diaphragmatic attenuation. In addition, PET images can provide information on absolute coronary blood flow and perfusion reserve. Reduced perfusion reserve occurs in patients with small vessel disease (as seen in diabetic patients and subjects with syndrome X) and may be seen in patients with diffuse triple vessel disease, where regional perfusion may appear normal. Although oxygen-15-labeled water is the preferred tracer for determining regional myocardial perfusion, it is not widely available. If the imaging facility has a nearby cyclotron, [13N]ammonia, with its 10 min half-life, is a suitable tracer (in spite of high liver uptake following rest injection). In the absence of an on-site cyclotron, PET perfusion studies are performed with generator-produced rubidium-82. The 75 s half-life rubidium-82 daughter is eluted from the strontium-82 generator directly into the patient. The short half-life permits administration of 30–50 mCi at rest during a dynamic 5–7 min data acquisition. The vasodilator (typically dipyridamole or regadenoson) is administered, followed by a second infusion of rubidium-82 with a dynamic acquisition.
As with single photon imaging, the images are reconstructed, placed in standard cardiac orientation, and evaluated for the regional distribution of perfusion, regional and global ventricular function, as well as perfusion reserve (Figure 29.1).


Fig. 29.1
Abnormal rubidium-82 PET myocardial perfusion scan at stress and rest. Short axis (top rows) vertical long axis (middle rows) and horizontal long axis (bottom rows) slices demonstrate severe and extensive ischemia involving the anterior, apical and septal walls. The left ventricular (LV) cavity is dilated on stress imaging consistent with transient ischemic dilation, a poor prognostic factor. Furthermore, on gated images (not shown), the patient’s LV ejection fraction decreased from 68% at rest to 46% during stress, an additional indicator of poor prognosis. Finally, the calculated coronary flow reserve was 0.92, which is severely decreased. Cardiac catheterization performed one day later confirmed the presence of severe triple vessel coronary artery disease
Tumor Involving the Myocardium or Pericardium
Primary cardiac tumors are rare: the most common primary cardiac neoplasm, accounting for 75% of cases, is a benign myxoma [33]. Malignant tumors of the heart are much less common, with an incidence of 0.0017–0.0028% [34]. Sarcomas account for about 95% of primary malignant tumors, while lymphoma accounts for the remainder [35]. The lesions are usually detected and characterized with ultrasound, computed tomography (CT), or magnetic resonance imaging (MRI). The role of radionuclide imaging, including [18F]FDG PET/CT, myocardial perfusion studies, or receptor specific imaging in these lesions, is unclear.
Secondary tumors occur 100–1,000 times more frequently than primary tumors [36]. Autopsy studies demonstrate that at the time of demise, ∼10% of patients with metastatic tumors have myocardial or pericardial involvement. Cardiac involvement occurs by direct extension, hematogenous, or retrograde lymphatic spread [37]. Cardiac involvement impairs function in ∼30% of patients. Depending on the etiology, myocardial involvement may be seen on myocardial perfusion images (usually as a focal area of decreased perfusion), [18F]FDG PET/CT (especially in patients with minimal myocardial [18F]FDG uptake, as a focal region of increased [18F]FDG localization—particularly in patients with melanoma or lymphoma), or with receptor agents such as 111In-pentetreotide (as a focal region of increased myocardial localization). Figure 29.2 shows an example of abnormal [18F]FDG uptake in a patient with myocardial involvement secondary to lymphoma.


Fig. 29.2
Abnormal myocardial uptake of [18F]FDG secondary to involvement by lymphoma. Top left panel: Maximum intensity projection (MIP) image of a patient with multiple [18F]FDG-avid lymphomatous lesions in the neck and chest. Other panels: Axial PET, axial CT and axial fused PET/CT slices showing intense focal [18F]FDG uptake at the apex of the left ventricle corresponding to a low attenuation mass in the myocardium, representing a lymphomatous deposit. (Courtesy of Tim Akhurst, Memorial Sloan Kettering Cancer Center, New York, NY)
Metastatic tumors that involve the pericardium include lung cancer and breast cancer (usually by direct involvement), leukemia, melanoma, and lymphoma [38]. Pericardial involvement can result in pericardial effusion, cardiac tamponade, or less frequently constrictive pericarditis. Long-term survivors of breast cancer or lymphoma (particularly those treated with radiation) may have chronic constrictive pericarditis.
It is important to recognize that a common site of intense, benign, myocardial [18F]FDG uptake is brown fat in the atrial septum due to lipomatous hypertrophy of the interatrial septum [39] (Fig. 29.3). This phenomenon is associated with obesity and advanced age. In patients with lung cancer, esophageal cancer, or metastatic melanoma, with lesions adjacent to the heart, additional imaging studies, such as MRI, may be required to make the distinction between lipomatous hypertrophy and myocardial metastasis [40]. In patients with [18F]FDG uptake in interatrial lipomatous hypertrophy, the intensity may vary on serial scans [41].


Fig. 29.3
Temporal variability of benign [18F]FDG uptake in lipomatous hypertrophy of the interatrial septum (LHIS) in a patient with breast cancer. Axial PET (left) and axial fused PET/CT (right) slices of the chest at baseline (bottom panels), 12 month followup (middle panels) and 19 month followup (top panels) demonstrate variable levels of [18F]FDG uptake in the atrial septum corresponding to a fat density structure on CT
Cardiac Function
First-pass and equilibrium radionuclide ventriculography (RVG) techniques were developed in the early 1970s [42]. Early studies reported an excellent correlation of RVG-derived left ventricular ejection fraction (LVEF) with values obtained by cardiac catheterization contrast ventriculography [43–45]. The patient population benefitting most from monitoring of cardiac function by RVG are those receiving potentially cardiotoxic therapies. Cancer patients who receive systemic chemotherapy are at risk for acute and chronic cardiovascular complications (Table 29.4) [46].
Table 29.4
Important cardiovascular side effects of cancer drugs
Side effect | Cancer drug | Rate/incidence | Mechanism | Reversible | Irreversible |
---|---|---|---|---|---|
Cardiovascular ischemia | Antimetabolites | ||||
5-Fluorouracil | Frequent | Vasoconstriction | +++ | + | |
Capecitabine | Infrequent | Vasoconstriction | +++ | + | |
Anti-VEGF drugs | |||||
Bevacizumab | <=3.8% | Arterial thromboembolism | ++ | ||
Sunitinib | <=3% | Arterial thromboembolism | ++ | ||
Sorafenib | <=3% | Arterial thromboembolism | ++ | ||
Arrhythmias | Anthracyclines | Dose dependent | Supraventricular, calcium overload | ++ | |
Anti-HER2/ErbB2 | |||||
Lapatinib | QTc prolongation, torsade | ||||
Anti-VEGF drugs | |||||
Sunitinib | Dose dependent | QTc prolongation, torsade | |||
Contractile dysfunction/ heart failure | Anthracyclines | Dose dependent | Myocardial cell death | +++ | |
Anti-HER2/ErbB2 | |||||
Trastuzumab | Dysfunction 3–18.1% | Myofibrillar disorganization | +++ | + | |
Heart Failure 0.4–3.6% | + | + | |||
Lapatinib | Dysfunction 1.4% | ? | + | ||
Heart failure 0.2% | + | ||||
Anti-VEGF drugs | |||||
Bevacizumab | Heart failure 1–3% | ? | + | ||
Sunitinib | Heart Failure 8–15% | Mitochondrial dysfunction | + | ||
Arterial hypertension | Anti-VEGF drugs | ||||
Bevacizumab | Any grade | ||||
Grade 3/4 | |||||
Sunitinib | Any grade | ||||
Grade 3/4 | |||||
Sorafenib | Any grade | ||||
Grade 3/4 |
Acute manifestations of chemotherapy-related cardiotoxicity include hypertension, QTc prolongation, pericarditis/myocarditis, supraventricular and ventricular arrhythmias, and acute coronary syndromes. Such manifestations can occur immediately upon initiation of therapy up to 2 weeks after completion of treatment, but rarely cause clinical symptoms and usually resolve spontaneously. Many of these effects are dose related and transient and are often ameliorated by withdrawal of the drug, or reduction of the dose. On the other hand, the most feared complication of therapy-related cardiotoxicity is the chronic-progressive form, which can potentially culminate in overt congestive heart failure.
Chronic-progressive Cardiotoxicity
Chronic-progressive chemotherapy-related cardiac dysfunction (CRCD) can occur early, within one year of therapy, or may develop late, greater than one year after the conclusion of therapy. Two distinct subtypes of CRCD are now recognized: type I and type II [47]. Type I CRCD is typified by anthracycline-associated cardiac toxicity, which is dose related, irreversible, and associated with characteristic ultrastructural abnormalities. Type II CRCD is characterized by the toxicity related to the monoclonal antibody trastuzumab, which is not dose related, does not appear to occur in all patients, and is not associated with significant ultrastructural abnormalities. Type II CRCD also appears to be reversible, with a high likelihood of recovery.
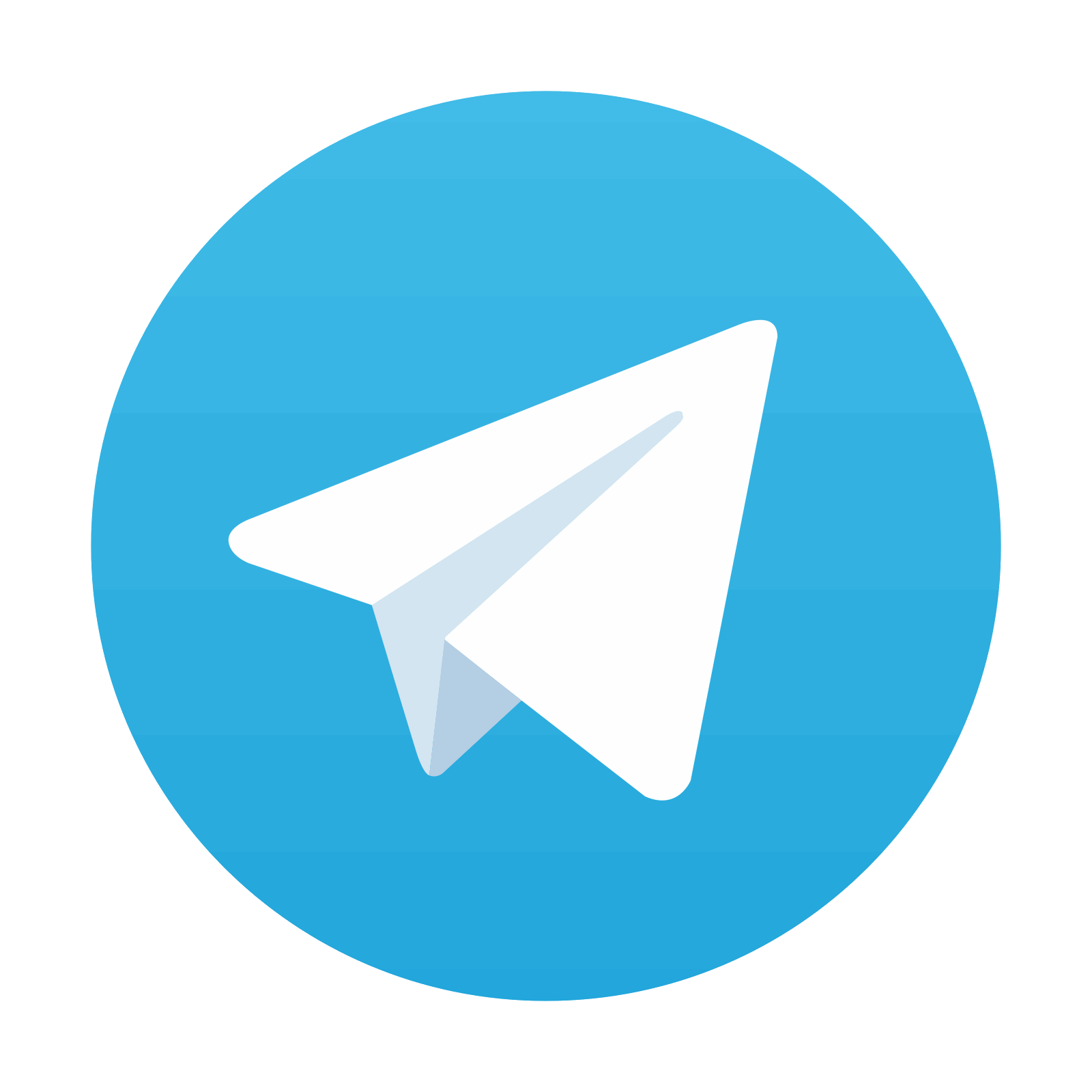
Stay updated, free articles. Join our Telegram channel

Full access? Get Clinical Tree
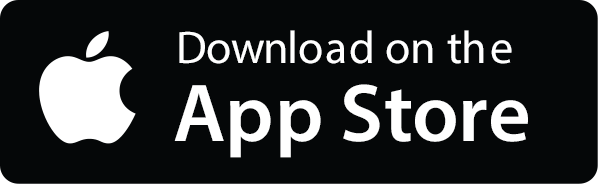
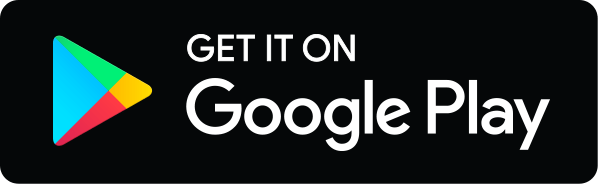