This article reviews existing and emerging techniques of interrogating metabolism in brain cancer from well-established proton magnetic resonance spectroscopy to the promising hyperpolarized metabolic imaging and chemical exchange saturation transfer and emerging techniques of imaging inflammation. Some of these techniques are at an early stage of development and clinical trials are in progress in patients to establish the clinical efficacy. It is likely that in vivo metabolomics and metabolic imaging is the next frontier in brain cancer diagnosis and assessing therapeutic efficacy; with the combined knowledge of genomics and proteomics a complete understanding of tumorigenesis in brain might be achieved.
Key points
- •
Many existing and emerging techniques of interrogating metabolism in brain cancer are at an early stage of development.
- •
A few clinical trials that employ these techniques are in progress in patients with brain cancer to establish the clinical efficacy of these techniques.
- •
It is likely that in vivo metabolomics and metabolic imaging is the next frontier in brain cancer diagnosis and assessing therapeutic efficacy.
MR spectroscopy/NMR spectroscopy–based metabolomics in brain tumor
Metabolomics is the “systematic study of the unique chemical fingerprints that specific cellular processes leave behind”, the study of their small-molecule metabolite profiles. Recently, metabolomics in cancer research is gaining considerable importance. The application of Nuclear Magnetic Resonance (NMR)-based and Magnetic Resonance Spectroscopy (MRS)-based metabolomics as applied to brain cancer is an evolving area of clinical use and investigation. In brain tumors, diagnosing tumor type and grade non-invasively has been a clinical challenge. 1 H MRS/NMR–based metabolomics has been explored to identify elevated metabolites in malignant tissue specifically in contrast with normal brain. Interestingly, most of the 1 H MRS in vivo studies to date have been done on the brain. This is primarily owing to the reduced effects of motion and lipid contamination in the brain. The global metabolic profile of live cells or cell extracts from established glioma models has been determined using standard NMR methods, and validated using tumor biopsies obtained from animal models or patients that have been imaged using high-resolution magic angle spinning spectroscopy. Such approaches complement data to the in vivo findings.
Detected Metabolites Using 1 H MR Spectroscopy
1 H MRS is used extensively to monitor the steady-state levels of major endogenous cellular metabolites. For a full review of in vivo 1 H MRS-detectable metabolites, see De Graaf. In the field of neurooncology, the most prevalent metabolites in the 1 H MR spectrum are N -acetylasparate (NAA), total choline-containing metabolites (Cho), lactate (Lac), mobile lipids, creatine (Cre), glutamate (Glu), glutamine (Gln), Gln and Glu, glycine, glutathione, and 2-hydroxyglutarate (2-HG). The largest signal in normal healthy brain tissue is NAA and the NAA level typically decreases in gliomas. The Cho signal is a composite of free choline, phosphocholine and glycerophosphocholine, which are the precursors and breakdown products of the main membrane phospholipid phosphatidylcholine. The intensity of this peak is associated with cell proliferation and cell signaling, and is typically increased in cancer. Lactate is the end product of aerobic glycolysis and is enhanced in cancer as part of the Warburg effect. Lipids (long chain fatty acids), especially lipid droplets known as mobile lipids or triglycerides, are rarely observed in the normal brain, but are often increased in glial tumors and are associated with cell death and increased necrosis. The Cre signal is a composite of Cre and phosphocreatine, which are involved in energy metabolism via the creatine kinase reaction. Cre levels vary within normal brain regions and in some cases with tumorigenesis. The amino acid Glu is the most abundant amino acid in the brain and an essential neurotransmitter. In gliomas, glutaminolysis is often required for tumor growth as an anaplerotic source of carbon complementary to glucose metabolism. Finally, with the recent discovery of the isocitrate dehydrogenase (IDH) mutation, the most common mutation in oligodendroglioma and astrocytoma tumors, increased levels of 2-HG, which is produced from α-ketoglutarate (α-KG) by mutant IDH, serve as a clear metabolic indicator for the presence of the mutation within a tumor and can also be detected by 1 H MRS when the mutation is present.
From a technical perspective, it is important to note that the length of the echo time (TE) used in 1 H MRS sequences defines which metabolites can be detected. Using a short TE (<50 ms), most metabolites can be observed, but overlappings between resonances often hampers proper quantification; on the other hand, when using a long TE (>120 ms), only a few metabolites remain visible, but their respective resonances can be readily identified and quantified.
Detected Metabolites Using 13 C MR Spectroscopy
Early 13 C MRS/NMR investigations were used to monitor glucose metabolism and Lac turnover during steady-state hyperglycemia with stable isotopically labeled, [1- 13 C] glucose in C6 glioma-bearing rats. Labeling of glucose-derived [3- 13 C] Lac, [4- 13 C] Glu, [4- 13 C] Gln, and [1- 13 C] glycogen could all be detected. More important, increased labeled Lac with reduced labeling in Glu and Gln were observed when comparing tumor with normal contralateral brain, consistent with the Warburg effect and a reduction in flux into the tricarboxylic acid (TCA) cycle. 13 C MRS studies investigating glioblastoma (GBM) cells and a combination of 13 C-labeled glucose and 13 C-labeled Gln have also shed light on the possible role of Gln in high-grade brain tumors. Conversion of Gln to Lac via glutaminolysis was found to be sufficient to produce NADPH required for fatty acid synthesis. More recently, studies of primary human GBM models in mice infused with 13 C-labeled glucose further demonstrated not only increased glycolysis, but also active glucose metabolism via the TCA cycle to Glu and Gln, confirming that flux via pyruvate dehydrogenase was not suppressed in GBM. However, this study showed limited glutaminolysis. Using 13 C MRS to probe the fate of 13 C-labeled acetate in orthotopic brain tumors, a recent investigation demonstrated that acetate is oxidized via the TCA cycle, together with glucose, to generate labeled Gln and Glu. This identifies an additional metabolite that could help to meet the high biosynthetic and bioenergetic demands of GBM tumor growth. Future studies using additional 13 C-labeled substrates could be envisaged to shed further light on the metabolism of GBM and, as models are being developed, on the metabolism of lower grade brain tumors.
Clinical 1 H MR Spectroscopy
Numerous studies have highlighted the potential benefits of using 1 H MRS to estimate metabolite levels in brain tumors in the clinic. When combined with similar spatial localization techniques that are used in generating anatomic MR images, this strategy can be used to produce maps of the variations in levels of choline containing compounds, Cre, NAA, Lac, and mobile lipids. With increased magnetic field strengths, improvements in scanner hardware and developments in software capabilities, the acquisition time for volumetric data is on the order of 5 to 10 minutes and the spatial resolution of the voxels obtained is typically 0.5 to 1 cm 3 . More recent advances in pulse sequence development and spectral editing schemes have facilitated the detection of metabolites with shorter T 2 relaxation times and lower signal-to-noise ratios such as Glu, Gln, Gln and Glu , and 2-HG, expanding the investigation of potential metabolic processes for both characterizing the spatial extent of gliomas and assessing therapeutic response.
For Tumor Characterization
The first studies to identify metabolic differences between gliomas and normal brain tissue date back to the mid-1990s and used a long TE (144 ms) 1 H MRS acquisition. Since then, numerous studies have shown that elevated levels of Cho and reduced levels of NAA together can distinguish regions of tumor from normal brain, define the spatial extent of abnormal metabolism owing to tumor beyond the contrast-enhancing lesion guide the selection of the biopsy site to the most aggressive part of the tumor and differentiate among tumor grades and types. The Cho-NAA index (CNI) is a metric that has been developed in the clinical setting to describe such changes and has been found to be more robust than ratios and absolute quantification. These in vivo results have been confirmed by correlating with both ex vivo histologic characteristics from image-guided tissue samples and, more recently, 1 H high-resolution magic angle spinning spectroscopy of tumor biopsies to show that regions with increased Cho and reduced NAA relative to normal brain that have a high probability of corresponding to tumor. However, despite the benefits of 1 H MRS imaging in improving sensitivity to metabolically active tumor and differentiating gliomas from metastatic disease, other disease processes such as inflammation can also cause a reduction in neuronal function while increasing cellularity, so alone MRS is not specific enough to differentiate tumor from inflammation.
For Predicting Outcome or Response to Therapy
In high-grade gliomas, higher Cho-to-Cre, higher Cho-to-NAA, higher Lac plus mobile lipids, and lower Cr-to-NAA abnormalities have been found to be associated with poor survival. In low-grade gliomas, fewer data sets are available on the prognostic value of MRS in the clinic. In one study, increased Cre was found to be a significant predictor for tumor progression and for malignant tumor transformation in grade II gliomas. Gliomas with decreased Cre seemed to have longer progression-free times and later malignant transformation. The information provided by MRS data is complementary to anatomic images and may often be more valuable than the contrast-enhancing lesion in assessing therapeutic response. The spatial extent of the metabolic lesion can also be used to plan focal therapy, such as external beam radiation therapy (RT) and gamma knife radiosurgery, and to assess the response to therapy. Alexander and colleagues showed that the mean tumor Cho/NAA ratio and normalized Cho decreased from baseline to after completion of external beam RT. In this study, patients who exhibited more than a 40% decrease in normalized Cho between midradiotherapy and postradiotherapy studies were associated with shorter survival times and faster disease progression. The Lac/NAA ratio at the fourth week of RT and the change in normalized Cho/Cre between baseline and week 4 of RT were also predictive of the outcome, suggesting the possible benefit of adaptive, response-based radiation treatment.
Although a breadth of MR imaging methods can provide extensive anatomic and functional information about brain tumors, the current use of colocalized 1 H MRS/NMR metabolomics approaches provides valuable information that can help clinicians to determine tumor margins, distinguish between progression and pseudoprogression, characterize tumor grade and IDH status, and predict response to therapy. Additionally, preclinical studies of brain tumor models continue to shed light on the complexities of glioma metabolism, leading to an improved understanding of cellular events that could be targetable for new therapeutic approaches. Furthermore, methods currently optimized in the preclinical setting and most notably the use of hyperpolarized agents, are poised to enter the clinic and will enhance the steady-state metabolic data with dynamic flux information that could further improve the detection of tumors and the early monitoring of therapeutic response. Collectively, the breadth of existing and emerging MRS methodologies available for the metabolic imaging of brain tumors could improve current paradigms significantly on diagnosis, treatment, and response assessment, advancing personalized patient care and quality of life.
MR spectroscopy/NMR spectroscopy–based metabolomics in brain tumor
Metabolomics is the “systematic study of the unique chemical fingerprints that specific cellular processes leave behind”, the study of their small-molecule metabolite profiles. Recently, metabolomics in cancer research is gaining considerable importance. The application of Nuclear Magnetic Resonance (NMR)-based and Magnetic Resonance Spectroscopy (MRS)-based metabolomics as applied to brain cancer is an evolving area of clinical use and investigation. In brain tumors, diagnosing tumor type and grade non-invasively has been a clinical challenge. 1 H MRS/NMR–based metabolomics has been explored to identify elevated metabolites in malignant tissue specifically in contrast with normal brain. Interestingly, most of the 1 H MRS in vivo studies to date have been done on the brain. This is primarily owing to the reduced effects of motion and lipid contamination in the brain. The global metabolic profile of live cells or cell extracts from established glioma models has been determined using standard NMR methods, and validated using tumor biopsies obtained from animal models or patients that have been imaged using high-resolution magic angle spinning spectroscopy. Such approaches complement data to the in vivo findings.
Detected Metabolites Using 1 H MR Spectroscopy
1 H MRS is used extensively to monitor the steady-state levels of major endogenous cellular metabolites. For a full review of in vivo 1 H MRS-detectable metabolites, see De Graaf. In the field of neurooncology, the most prevalent metabolites in the 1 H MR spectrum are N -acetylasparate (NAA), total choline-containing metabolites (Cho), lactate (Lac), mobile lipids, creatine (Cre), glutamate (Glu), glutamine (Gln), Gln and Glu, glycine, glutathione, and 2-hydroxyglutarate (2-HG). The largest signal in normal healthy brain tissue is NAA and the NAA level typically decreases in gliomas. The Cho signal is a composite of free choline, phosphocholine and glycerophosphocholine, which are the precursors and breakdown products of the main membrane phospholipid phosphatidylcholine. The intensity of this peak is associated with cell proliferation and cell signaling, and is typically increased in cancer. Lactate is the end product of aerobic glycolysis and is enhanced in cancer as part of the Warburg effect. Lipids (long chain fatty acids), especially lipid droplets known as mobile lipids or triglycerides, are rarely observed in the normal brain, but are often increased in glial tumors and are associated with cell death and increased necrosis. The Cre signal is a composite of Cre and phosphocreatine, which are involved in energy metabolism via the creatine kinase reaction. Cre levels vary within normal brain regions and in some cases with tumorigenesis. The amino acid Glu is the most abundant amino acid in the brain and an essential neurotransmitter. In gliomas, glutaminolysis is often required for tumor growth as an anaplerotic source of carbon complementary to glucose metabolism. Finally, with the recent discovery of the isocitrate dehydrogenase (IDH) mutation, the most common mutation in oligodendroglioma and astrocytoma tumors, increased levels of 2-HG, which is produced from α-ketoglutarate (α-KG) by mutant IDH, serve as a clear metabolic indicator for the presence of the mutation within a tumor and can also be detected by 1 H MRS when the mutation is present.
From a technical perspective, it is important to note that the length of the echo time (TE) used in 1 H MRS sequences defines which metabolites can be detected. Using a short TE (<50 ms), most metabolites can be observed, but overlappings between resonances often hampers proper quantification; on the other hand, when using a long TE (>120 ms), only a few metabolites remain visible, but their respective resonances can be readily identified and quantified.
Detected Metabolites Using 13 C MR Spectroscopy
Early 13 C MRS/NMR investigations were used to monitor glucose metabolism and Lac turnover during steady-state hyperglycemia with stable isotopically labeled, [1- 13 C] glucose in C6 glioma-bearing rats. Labeling of glucose-derived [3- 13 C] Lac, [4- 13 C] Glu, [4- 13 C] Gln, and [1- 13 C] glycogen could all be detected. More important, increased labeled Lac with reduced labeling in Glu and Gln were observed when comparing tumor with normal contralateral brain, consistent with the Warburg effect and a reduction in flux into the tricarboxylic acid (TCA) cycle. 13 C MRS studies investigating glioblastoma (GBM) cells and a combination of 13 C-labeled glucose and 13 C-labeled Gln have also shed light on the possible role of Gln in high-grade brain tumors. Conversion of Gln to Lac via glutaminolysis was found to be sufficient to produce NADPH required for fatty acid synthesis. More recently, studies of primary human GBM models in mice infused with 13 C-labeled glucose further demonstrated not only increased glycolysis, but also active glucose metabolism via the TCA cycle to Glu and Gln, confirming that flux via pyruvate dehydrogenase was not suppressed in GBM. However, this study showed limited glutaminolysis. Using 13 C MRS to probe the fate of 13 C-labeled acetate in orthotopic brain tumors, a recent investigation demonstrated that acetate is oxidized via the TCA cycle, together with glucose, to generate labeled Gln and Glu. This identifies an additional metabolite that could help to meet the high biosynthetic and bioenergetic demands of GBM tumor growth. Future studies using additional 13 C-labeled substrates could be envisaged to shed further light on the metabolism of GBM and, as models are being developed, on the metabolism of lower grade brain tumors.
Clinical 1 H MR Spectroscopy
Numerous studies have highlighted the potential benefits of using 1 H MRS to estimate metabolite levels in brain tumors in the clinic. When combined with similar spatial localization techniques that are used in generating anatomic MR images, this strategy can be used to produce maps of the variations in levels of choline containing compounds, Cre, NAA, Lac, and mobile lipids. With increased magnetic field strengths, improvements in scanner hardware and developments in software capabilities, the acquisition time for volumetric data is on the order of 5 to 10 minutes and the spatial resolution of the voxels obtained is typically 0.5 to 1 cm 3 . More recent advances in pulse sequence development and spectral editing schemes have facilitated the detection of metabolites with shorter T 2 relaxation times and lower signal-to-noise ratios such as Glu, Gln, Gln and Glu , and 2-HG, expanding the investigation of potential metabolic processes for both characterizing the spatial extent of gliomas and assessing therapeutic response.
For Tumor Characterization
The first studies to identify metabolic differences between gliomas and normal brain tissue date back to the mid-1990s and used a long TE (144 ms) 1 H MRS acquisition. Since then, numerous studies have shown that elevated levels of Cho and reduced levels of NAA together can distinguish regions of tumor from normal brain, define the spatial extent of abnormal metabolism owing to tumor beyond the contrast-enhancing lesion guide the selection of the biopsy site to the most aggressive part of the tumor and differentiate among tumor grades and types. The Cho-NAA index (CNI) is a metric that has been developed in the clinical setting to describe such changes and has been found to be more robust than ratios and absolute quantification. These in vivo results have been confirmed by correlating with both ex vivo histologic characteristics from image-guided tissue samples and, more recently, 1 H high-resolution magic angle spinning spectroscopy of tumor biopsies to show that regions with increased Cho and reduced NAA relative to normal brain that have a high probability of corresponding to tumor. However, despite the benefits of 1 H MRS imaging in improving sensitivity to metabolically active tumor and differentiating gliomas from metastatic disease, other disease processes such as inflammation can also cause a reduction in neuronal function while increasing cellularity, so alone MRS is not specific enough to differentiate tumor from inflammation.
For Predicting Outcome or Response to Therapy
In high-grade gliomas, higher Cho-to-Cre, higher Cho-to-NAA, higher Lac plus mobile lipids, and lower Cr-to-NAA abnormalities have been found to be associated with poor survival. In low-grade gliomas, fewer data sets are available on the prognostic value of MRS in the clinic. In one study, increased Cre was found to be a significant predictor for tumor progression and for malignant tumor transformation in grade II gliomas. Gliomas with decreased Cre seemed to have longer progression-free times and later malignant transformation. The information provided by MRS data is complementary to anatomic images and may often be more valuable than the contrast-enhancing lesion in assessing therapeutic response. The spatial extent of the metabolic lesion can also be used to plan focal therapy, such as external beam radiation therapy (RT) and gamma knife radiosurgery, and to assess the response to therapy. Alexander and colleagues showed that the mean tumor Cho/NAA ratio and normalized Cho decreased from baseline to after completion of external beam RT. In this study, patients who exhibited more than a 40% decrease in normalized Cho between midradiotherapy and postradiotherapy studies were associated with shorter survival times and faster disease progression. The Lac/NAA ratio at the fourth week of RT and the change in normalized Cho/Cre between baseline and week 4 of RT were also predictive of the outcome, suggesting the possible benefit of adaptive, response-based radiation treatment.
Although a breadth of MR imaging methods can provide extensive anatomic and functional information about brain tumors, the current use of colocalized 1 H MRS/NMR metabolomics approaches provides valuable information that can help clinicians to determine tumor margins, distinguish between progression and pseudoprogression, characterize tumor grade and IDH status, and predict response to therapy. Additionally, preclinical studies of brain tumor models continue to shed light on the complexities of glioma metabolism, leading to an improved understanding of cellular events that could be targetable for new therapeutic approaches. Furthermore, methods currently optimized in the preclinical setting and most notably the use of hyperpolarized agents, are poised to enter the clinic and will enhance the steady-state metabolic data with dynamic flux information that could further improve the detection of tumors and the early monitoring of therapeutic response. Collectively, the breadth of existing and emerging MRS methodologies available for the metabolic imaging of brain tumors could improve current paradigms significantly on diagnosis, treatment, and response assessment, advancing personalized patient care and quality of life.
Gas chromatography/mass spectrometry–based metabolomics of brain cancer
Besides NMR-based metabolomics, metabolomics based on gas chromatography/mass spectrometry are increasingly applied in brain cancer research but at this point are mostly restricted to ex vivo samples. However, mass spectrometry imaging is emerging as a powerful tool for directly determining the distribution of proteins, peptides, lipids, neurotransmitters, metabolites, and drugs in situ and has enormous potential as a clinical tool for interrogating metabolism in brain cancer.
Gas Chromatography/Mass Spectrometry–Based Metabolomic Analysis of Cerebrospinal Fluid from Glioma Patients
Nakamizo and colleagues analyzed cerebrospinal fluid from patients with glioma using gas chromatography/mass spectrometry to correlate metabolomic profiles based on World Health Organization tumor grades, tumor location, contrast in MR images, and IDH mutations. Samples were separated into 3 groups: grade I and II glioma, grade III glioma, and glioblastoma (GBM). Grades I and II and grade III samples did not differ in their metabolomic content. However, in GBM samples, citric and isocitric acid were increased compared with the other 2 groups, and lactic acid was higher relative to grades I and II samples. Succinic, fumaric, and malic acid were decreased in GBM samples relative to grades I and II and grade III gliomas. For samples that originated from tumors proximal to the ventricles, high-grade tumors possessed elevated levels of citric and isocitric acid, and low-grade tumors had elevated levels of lactic acid, compared with samples originating in tumors distal to the ventricles. In high-grade tumors that displayed gadolinium enhancement in MR images, citric and isocitric acid levels were higher than those tumors that did not display this enhancement. Levels of citric, isocitric, and lactic acid were higher in samples with mutated IDH than those with wild-type IDH, whereas the combined signal from pyruvate and oxaloacetic acid was decreased in the mutated IDH samples. The correlation between overall survival and concentrations of these metabolites was also investigated. It was found that in malignant glioma (grades III and IV), elevated levels of lactic acid were correlated to lower overall survival. Although not significant, there was a trend between elevated levels of citric and lactic acid and lower overall survival in all gliomas.
Metabolomic Patterns in Glioblastoma and Changes During Radiotherapy
Wibom and colleagues examined the metabolic profile of GBM patients before and after radiotherapy. Extracellular fluid was extracted from the tumor and brain adjacent to tumor (BAT) via microdialysis before and during treatment, similar to the procedure performed by Tabatabaei and colleagues and was analyzed with gas chromatography time-of-flight mass spectrometry. Reference samples were also collected subcutaneously from the abdomen. Tumor tissue was found to express lower levels of glucose than BAT and the subcutaneous tissue. Several amino acids were found in greater quantities in the tumor relative to the BAT, including the essential amino acids, l -threonine, allothreonine, l -tryptophan, l -arginine, l -lysine, and l -valine. Glycine and Glu levels were also increased in the tumor compared with the BAT. After radiotherapy was administered, Glu and Gln levels increased in both tumor and BAT regions. Ethanolamine and glycerol seemed to decrease in the tumor but increase in the BAT in addition to glycerol. Although the metabolic profile varied among the patients, it was suggested that the trends in the tumor metabolome could be monitored individually to help assess the efficacy of radiotherapy.
Metabolomic Screening of Glioma Patients Reveals Diagnostic and Prognostic Information
Moren and colleagues conducted a study that compared the metabolic profiles of tumor samples and serum from different World Health Organization grades of GBM and oligodendroglioma via gas chromatography time-of-flight mass spectrometry. Correlations were determined between metabolite levels and survivability of the 2 diseases. Increased levels of 2-hydroxyglutaric acid, 4-aminobutyric acid (GABA), creatinine, glycerol-2-phosphate, glycerol-3-phosphate, ribitol, and myo-inositol were found in tumor samples, and lysine and 2-oxoisocaproic acid in serum from oligodendroglioma patients, relative to samples from GBM patients. Compared with oligodendroglioma samples, levels of mannitol and phenylalanine in GBM tumor samples and cysteine from serum were increased. Survival groups were split into short survival (4 months) and long survival (3 years), where the survival is measured as the time after diagnosis. In GBM, elevated levels of glycerol-3-phoshate, myo-inositol, ribitol, and fructose were associated with long survival. In oligodendroglioma levels of ribitol, myo-inositol and spermidine were higher for long survival patients, whereas short survival patients expressed elevated levels of glycine and aminomalonic acid.
Metabolomics of Human Cerebrospinal Fluid Identifies Signatures of Malignant Glioma
The first study to delineate the metabolic differences in cerebrospinal fluid samples between malignant and nonmalignant glioma patients was investigated by Locasale and colleagues through the use of a liquid chromatography/tandem mass spectrometry. Several metabolites that were significantly altered between the malignant and nonmalignant samples were identified. A correlation between tumor size and metabolite levels was also determined. Acetylcarnitine, acetoacetate, phenylpropiolic acid, and cholesteryl sulfate were correlated positively with tumor size whereas myo-inositol and cytidine were correlated negatively.
The Metabolomic Signature of Malignant Glioma Reflects Accelerated Anabolic Metabolism
The metabolic differences between various World Health Organization grades of glioma tumors were analyzed by Chinnaiyan and colleagues via ultrahigh performance liquid chromatography/tandem mass spectrometry and gas chromatography/mass spectrometry platforms. There was a poor distinction of grade III tumors from either grade II or grade IV owing to overlap in their metabolomic profiles. Amino acid metabolism was altered in grade IV tumors, including increased levels of glutathione and tryptophan and a decrease in Cre levels. An analysis of lipid metabolism in grade IV tumors revealed higher levels of essential and medium chain fatty acids and metabolites associated with carnitine metabolism and lower levels of glycolipids, lysoplipids, and sterols. Although phosphoenolpyruvate and 3-phosphoglycerate levels were increased in grade IV tumors, there was an overall decrease in carbohydrate metabolism. Nucleotide metabolism, particularly pyrimidine catabolism, was found to increase in grade IV tumors. Random forest (RF) analysis was performed to determine which metabolites contributed the most to changes in global metabolism among the different grades of tumors. 2-HG was found to be the most significant biochemical that best delineates tumor grades. An investigation of glycolysis and the oxidative energy metabolism resulted in a more than 7-fold increase in the glycolytic intermediates 3-phosphoglycerate and phosphoenolpyruvate in grade IV tumors compared with grade II tumors. Furthermore, the metabolites 6-P-gluconate, ribose-5-phosphate, serine, and glycine, which contribute to the glycolytic pathway, are significantly increased in grade IV tumors. Interestingly, Gln, which is found to be a major component of tumorigenesis, was not found to be associated with tumor grade.
Hyperpolarized MR imaging and metabolic imaging in brain cancer
MR imaging invaluably benefits many brain diseases patients due to its noninvasive investigation. 13 C MRS has been studied as another diagnostic modality in the brain tumor model of animals and in patients with brain tumor because 13 C labeling compounds provide specific metabolic information with less background noise and can provide dynamic metabolic analysis with 13 C flux analysis. However, the low inherent sensitivity compared with proton MRS must be compensated for in clinical applications. Recently, the novel rapid dissolution process of dynamic nuclear polarization technique was developed to retain strongly polarized nuclear spins in the liquid state. This method can provide more than a 10,000-fold signal enhancement enough to investigate the metabolic changes at the cellular level. Many 13 C labeled compounds are used for detecting the abnormality of metabolic fluxes or the accumulation of metabolites in human cancer such as succinate, α-KG, and pyruvate and ethyl pyruvate. Hyperpolarized 13 C pyruvate and α-KG are now studied actively for brain cancer metabolism and the first clinical trial with hyperpolarized 13 C pyruvate in GBM patients is underway at the University of California, San Francisco (UCSF). This same group at UCSF conducted the first in human clinical study of metabolic imaging in prostate cancer using hyperpolarized 13 C pyruvate.
[1- 13 C] Pyruvate
A number of preclinical studies in brain tumors have shown that one can distinguish brain tumors from normal tissue by examining in vivo metabolism and assess early response to treatment of high-grade gliomas in animal models. Recently, hyperpolarized 13 C pyruvate kinetic data were acquired from a healthy cynomolgus monkey brain using optimized 13 C coils and pulse sequences which demonstrated the feasibility of using hyperpolarized 13 C pyruvate for assessing in vivo metabolism for brain tumor patients in the near future, like the first human clinical trial using 13 C metabolic imaging for prostate cancer. All these findings suggest that hyperpolarization may be a promising tool for noninvasive cancer diagnosis and treatment assessment in the clinical setting.
[1- 13 C] α-Ketoglutarate
Mutations in IDH 1 and 2 present in 70% of low-grade gliomas and 5-10% of glioblastoma in adults. These mutations are associated with the production of the oncometabolite 2-HG instead of α-KG in the brain tumor, which contributes to the formation and malignant progression of gliomas. This accumulated 2-HG can be detected using in vivo proton MRS acquisitions in patients on a 3T MR imaging scanner and this noninvasive 2-HG detection shows its feasibility as a diagnostic and prognostic biomarker. Also, the injected hyperpolarized 13 C α-KG interrogated the accumulation of hyperpolarized 2HG or the conversion to hyperpolarized 13 C Glu, a potential biomarker for glioma.
Acetate Metabolism in the Brain
Many studies done 10 to 15 years ago show the high use of acetate directly in the brain and its specific use as a glial fuel. Recently, this dependence on acetate as an energy source has been found not only in glial cells but also in GBM and brain metastases. Most studies determine and compare the amount and rate of conversion of isotopically labeled acetate relative to labeled glucose using direct infusion techniques. In one such study, the kinetic parameters for 2- 13 C acetate transport and conversion was determined by infusing the compound into rats at different rates. The labeled compound was found to quickly accumulate in the brain, but its conversion to other metabolites is the rate-limiting step. With plasma concentrations around 2 to 3 mmol/L, the rate of acetate use was 0.5 μmol/g per minute and its transport into the brain was calculated to be 0.96 μmol/g per minute. In addition, the 13 C label enrichment of the C4 position of Gln was 44% and the C4 position of Glu was 14% with 2- 13 C acetate infusion. Similar studies have been done in humans with plasma concentrations of 1 mmol/L and show similar incorporation of the 13 C label of acetate into Gln and Glu in the brain.
The use of acetate in GBM and brain metastases was found using both ex vivo and in vivo NMR techniques after infusions of 1,2- 13 C acetate in human orthotopic tumor mouse models. The acetate-to-glucose ratio in five GBM tumor models was around 5-fold higher than in normal control brain tissue. The rate of incorporation of acetate into the citric acid cycle was found to be directly correlated to the amount of acetyl-coenzyme A (CoA) synthetase enzyme 2 (ACSS2). In humans, there are 3 acetyl-CoA synthetase enzymes—ACSS1, ACSS2, and ACSS3. Both ACSS1 and ACSS3 are found in the matrix of the mitochondria; however, ACSS2 is found mainly in the cytosol of the cell. In GBM, the amount of ACSS2 was found to be directly correlated to the grade of the tumor with high staining in (100% positive) in grade IV tumors compared with normal brain tissue. These recent findings have initiated new metabolic research in acetate and cancer progression.
Hyperpolarized 1- 13 C Acetate
Acetate was one of the first compounds to be hyperpolarized using solid state dynamic nuclear polarization. Since its initial polarization, several improvements in formulation and use of other techniques have significantly improved the percentage of polarization and increased the amount (final concentration) of hyperpolarized acetate that can be generated. The compound has been polarized using both the preclinical dynamic nuclear polarization polarizer and the clinical polarizer. Our laboratory recently reported a method of chemically converting hyperpolarized 1,2- 13 C pyruvate to hyperpolarized 1- 13 C acetate, 13 C-bicarbonate, and 13 C carbon dioxide using chemical reaction–induced multimolecular polarization. Even with these improvements in 1- 13 C acetate polarization, significant progress needs to be made for its rate of conversion to Gln, Glu, acetyl-CoA, and citrate to be useful in diagnostic imaging in the time scale of polarization (<5 minutes). A thorough discussion and execution of using hyperpolarized acetate to diagnosis metabolic changes in diabetic rats was published recently. One of the main mechanisms acetyl-CoA gets shuttled into the mitochondria is through its conversion into acetylcarnitine through carnitineacetyltransferase-1 and then back to carnitine and acetyl-CoA in the mitochondria thorough carnitineacetyltransferase-2. The Stodkilde–Jorgensen group could not detect a difference in the conversion rate of hyperpolarized acetate to acetylcarnitine in the time window of hyperpolarization and stated this is probably owing to low rate of conversion of the enzyme. They observe similar results to the 13 C nonhyperpolarized infusion studies done in the brain; the transport of acetate into specific tissues is fast; however, the rate of conversion is the rate-limiting step and is significantly slower than hyperpolarized 1- 13 C pyruvate. Another problem with 1- 13 C acetate is that other metabolites that would incorporate the 13 C label such as citrate have similar chemical shifts to acetate. Using in vivo spectroscopy, it is thus difficult to separate the hyperpolarized resonance of hyperpolarized 1- 13 C acetate from its metabolite 5- 13 C citrate. Other compounds might be better suited to interrogate the citric acid cycle or fatty acid synthesis in the brain in the time scale of polarization.
A recent paper by Rolf Gruetter’s laboratory illustrates another method of using the hyperpolarization of 1- 13 C acetate. His group generated a pulse sequence that transferred the polarized signal in 1,2- 13 C acetate from the carbonyl carbon (C1) to the methyl carbon (C2) in the brain of a rat and from the C1 position to a proton on the methyl group of acetate. This method allows for a whole new group of metabolites and chemical resonances to be observed. This transfer sequence could allow for hyperpolarized acetate to become clinical metabolic imaging agent.
Hyperpolarized Metabolic Spectroscopy in Brain Cancer Cell Lines
Hyperpolarized MRS has been applied in brain tumor cell line models in efforts to potentially inform medical decision making in novel and clinically useful ways. Various studies have used this technique, which could provide information on the diagnosis, treatment monitoring, and prognosis of brain tumors in a noninvasive manner.
With regard to diagnosing brain tumors, the differing signal levels provided by hyperpolarized 13 C-labeled pyruvate and 13 C-labeled Lac can be used to distinguish brain tumor and normal brain tissue. In one study, different GBM cell lines (U251 and U87) were used to generate orthotopic xenografts in the rat brain. Levels of 13 C-labeled pyruvate and 13 C-labeled Lac were elevated in the tumor tissue compared with the normal brain tissue. Furthermore, the pattern of histopathology in the cell lines studied correlated with the amount of signal detected. The U251 GBM cell line exhibits large areas of necrosis and hypoxia; in comparison, the U87 GBM cell line has very little necrosis and hypoxia. The signal to noise ratio of hyperpolarized 13 C-labeled Lac, pyruvate, and total carbon was significantly greater in U87 tumors than in U251 tumors; this was thought to be owing to the greater number of viable cells in the U87 line.
Compressed sensing has been used with hyperpolarized MRS to evaluate tumor tissues with heterogeneous metabolic profiles owing to varying levels of necrosis and hypoxia. Compressed sensing allows for the reduction of acquisition time and higher spatial resolution than conventional imaging; this permits the characterization of tumors with a heterogeneous nature like GBM. Differentiation of tumor tissue that was hypoxic/necrotic, nonhypoxic/nonnecrotic, or normal brain tissue in an orthotopic human GBM xenograft model was possible using this technique. Specifically, highly necrotic and hypoxic tumor tissue had absent or low levels of 13 C-labeled pyruvate and 13 C-labeled Lac, whereas tumor tissue with minimal levels of necrosis and hypoxia had high levels of 13 C-labeled pyruvate and 13 C-labeled Lac. If used in the clinic, hyperpolarized pyruvate with MRS and compressed sensing could be particularly useful in noninvasively determining tumor tissue types in GBM.
In addition to characteristic signal levels of hyperpolarized 13 C-labeled metabolites in tumor versus normal brain tissue, the apparent rate constant ( k PL ) for the conversion of pyruvate to Lac can also be used to distinguish cancer and normal tissue. Orthotopic xenografts of C6 glioma had larger Lac/pyruvate ratios as well as a larger k PL compared with normal tissue. Furthermore, k PL may even be a more robust marker than the Lac/pyruvate ratio in distinguishing cancerous brain tissue. The k PL has significantly less variability than the Lac/pyruvate ratio when used to differentiate tumor tissue from normal brain tissue.
Hyperpolarized techniques could also be applied to monitor treatment response in brain tumors; tumor response to RT and to different methods of chemotherapy, such as temozolomide (TMZ), everolimus, and LY294002, have been studied with hyperpolarized 13 C-labeled metabolites as a metric for response. TMZ is an alkylating agent used to treat brain tumors and is the first-line agent in treating GBM. Everolimus and LY294002 are drugs that target the PI3K/AKT/mammalian target of rapamycin pathway, which regulates the cell cycle. Using conversion of hyperpolarized pyruvate to Lac as a metric, tumor response to radiation treatment by a C6 glioma orthotopic tumor model was examined. By 72 hours after irradiation, the ratio of hyperpolarized Lac in tumor-to-maximum pyruvate in blood vessels was decreased by 34% compared with untreated tumors.
Treatment with TMZ was found to decrease the hyperpolarized pyruvate/Lac ratio in an orthotopic rat model of human GBM. This response could be detected just 1 day after TMZ treatment, whereas imaging-based evidence of tumor volume shrinkage owing to treatment did not occur until 5 to 7 days after treatment. These results were supported by a second study that used a bioreactor to maintain GBM cells treated with TMZ during hyperpolarized imaging. TMZ-treated cells showed a decrease in conversion of hyperpolarized pyruvate to Lac compared with untreated cells. Additionally, the authors of the study found that treatment with TMZ correlated with a decrease in pyruvate kinase, which is a glycolytic enzyme that indirectly controls pyruvate to Lac conversion.
In hyperpolarized studies of everolimus treatment in an orthotopic rate model of GBM, a significant drop in hyperpolarized Lac/pyruvate ratio compared with control was observed 7 days after treatment. At this same time point, conventional MR imaging was unable to detect a difference in tumor size between the treated and control group. It was not until 15 days after treatment that inhibition of tumor growth was appreciated on MR imaging. Bioreactor studies of everolimus and LY294002 treated GBM cells support these results. Decreased hyperpolarized Lac levels were observed in cells treated with these drugs compared with untreated controls. In addition to decreased hyperpolarized Lac levels, decreased phosphocholine levels were found to correlate with treatment using these agents. This is expected because the PI3K/AKT/mammalian target of rapamycin pathway controls both the synthesis of phosphocholine and Lac via a common transcription factor hypoxia inducible factor-1-α.
The balance between glycolysis and oxidative phosphorylation can provide important information regarding treatment response. Because many cancer cells derive most of their energy via glycolysis and normal cells via oxidative phosphorylation, the ability to detect if a tumor mainly uses one pathway over the other can indicate continued malignancy or positive response to treatment. One study was able to detect and quantify conversion of hyperpolarized 13 C-bicarbonate from 13 C-pyruvate in vivo for the first time. Because CO 2 is a byproduct of the flux from pyruvate to acetyl-CoA, bicarbonate can be used as a surrogate marker for mitochondrial metabolism, which is predominant in normal cells. The study found that Lac levels were significantly greater in glioma, and bicarbonate levels were significantly greater in normal brain tissue.
A bioreactor study of GBM cells with mutated IDH1 showed elevated levels of hyperpolarized 2-HG compared with GBM cells with wild-type IDH1. IDH mutation was also found to correlate with decreased activity of branched chain amino acid transaminase. Branched chain amino acid transaminase catalyzes the transamination of branched chain amino acids while converting α-KG to Glu. Thus, in cells with IDH1 mutation, a decrease in branched chain amino acid transaminase activity is expected to correlate with decreased Glu production. This was found to be the case in a bioreactor study of GBM cells with IDH1 mutation; hyperpolarized 13 C-Glu production was decreased in mutant cells compared with wild-type cells. As biomarkers of metabolic imaging, hyperpolarized 2-HG and Glu may potentially provide useful information in regards to detecting IDH1 mutation and treatment monitoring of brain tumors with IDH1 mutation.
Currently, the main tool for cancer diagnosis and monitoring is 18 fluoro-2-deoxyglucose (FDG)-PET. In brain tumors, however, FDG-PET shows increased signal in surrounding brain tissue owing to the high uptake that masks the signal generated by the tumor itself. Furthermore, pseudoprogression is a misleading phenomenon in which there is contrast enhancement of the tumor after treatment with radiotherapy or chemotherapy despite positive tumor response to the treatment. Diagnosis and treatment monitoring with hyperpolarized metabolic techniques could help mitigate this confounding clinical problem.
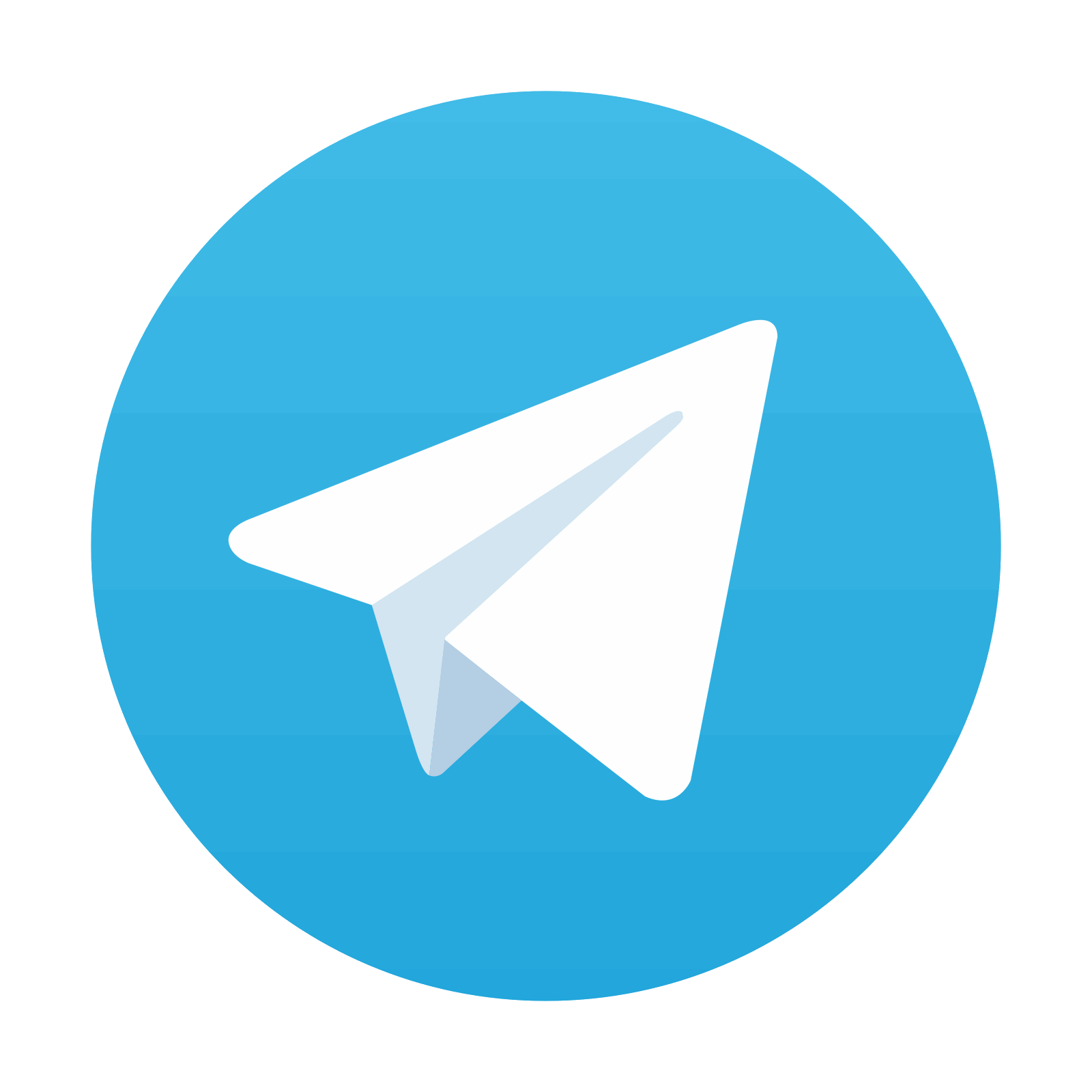
Stay updated, free articles. Join our Telegram channel

Full access? Get Clinical Tree
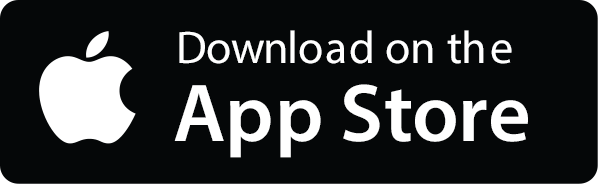
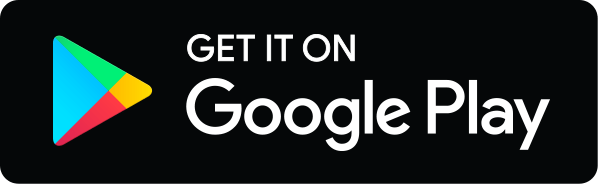