Fig. 20.1
Observed survival rates for 37,166 patients with kidney cancer classified by the current AJCC staging classification. Data taken from the National Cancer Data Base (Commission on Cancer of the American College of Surgeons and the American Cancer Society) for the years 2001–2002. Stage I includes 18,912 patients; Stage II, 4,443; Stage III, 5,952; and Stage IV, 7,859. (Used with the permission of the American Joint Committee on Cancer (AJCC), Chicago, IL. The original source for this material is the AJCC Cancer Staging Manual, Seventh Edition (2010) published by Springer Science and Business Media LLC www.springer.com)
Table 20.1
TNM and stage grouping of renal cell carcinoma
Definitions of TNM | |
---|---|
Primary tumor (T) | |
TX | Primary tumor cannot be assessed |
T0 | No evidence of primary tumor |
T1 | Tumor 7 cm or less in greatest dimension, limited to the kidney |
T1a | Tumor 4 cm or less in greatest dimension, limited to the kidney |
T1b | Tumor more than 4 cm but not more than 7 cm in greatest dimension limited to the kidney |
T2 | Tumor more than 7 cm in greatest dimension, limited to the kidney (Fig. 43.5) |
T2a | Tumor more than 7 cm but less than or equal to 10 cm in greatest dimension, limited to the kidney |
T2b | Tumor more than 10 cm, limited to the kidney |
T3 | Tumor extends into major veins or perinephric tissues but not into the ipsilateral adrenal gland and not beyond Gerota’s fascia |
T3a | Tumor grossly extends into the renal vein or its segmental (muscle containing) branches, or tumor invades perirenal and/or renal sinus fat but not beyond Gerota’s fascia |
T3b | Tumor grossly extends into the vena cava below the diaphragm |
T3c | Tumor grossly extends into the vena cava above the diaphragm or invades the wall of the vena cava |
T4 | Tumor invades beyond Gerota’s fascia (including contiguous extension into the ipsilateral adrenal gland) |
Regional lymph nodes (N) | |
NX | Regional lymph nodes cannot be assessed |
N0 | No regional lymph node metastasis |
N1 | Metastasis in regional lymph node(s) |
Distant metastasis (M) | |
M0 | No distant metastasis |
M1 | Distant metastasis |
Anatomic stage/prognostic groups | |||
---|---|---|---|
Stage I | T1 | N0 | M0 |
Stage II | T2 | N0 | M0 |
Stage III | T1 or T2 | N1 | M0 |
T3 | N0 or N1 | M0 | |
Stage IV | T4 | Any N | M0 |
Any T | Any N | M1 |
The common utilization of ultrasound and radiology has increased the number of incidentally detected renal tumors. There is a great heterogeneity among small renal masses, which results in complexity in the management of patients. About 20% of masses are benign, 60% are probably indolent variants of RCC, and only 20% are potentially aggressive tumors [7, 26, 27]. CT is widely used to differentiate benign from malignant lesions [28]. Renal oncocytoma and angiomyolipoma represent the most common benign lesions which have to be differentiated from RCC [28]. Enhancement with intravenous contrast correlates with malignant disease in about 80% of cases, although small renal tumors and those in young women are more likely to be benign [7]. Small (≤4 cm) solid enhancing (becoming brighter on CT with contrast) renal masses must be considered highly suspicious for RCC [7]. Although RCC can appear as iso-, hyper- or hypodense lesions on native CT scans, it usually demonstrates a significant contrast enhancement and intratumoral areas of necrosis following i.v. application of contrast [28]. However, the management of these lesions has caused great controversy over the past decade. Benign lesions such as renal oncocytoma are most often homogenous lesions exhibiting hypodensity compared to the normal renal parenchyma following i.v. application of contrast dye. The sensitivity and specificity of helical CT scans for differentiating conventional RCC from the other subtypes have been demonstrated to be 74% and 100% when 84 H is used as the cutoff value in the corticomedullary phase and 84% and 91% when 44 H is used as the cutoff value in the excretory phase [29]. Triple-phase contrast abdominal CT is the current investigation of choice for the confirmation of diagnosis and staging in most instances [16].
Venous tumor thrombus represents a significant problem in RCC, with 15–25% of patients having some degree of venous invasion [28]. Since an aggressive surgical approach is the mainstay of these patients, good presurgical planning depends on the preoperative evaluation of RCC with determination of tumor thrombus stage. Involvement of the renal vein and extension into the inferior vena cava below or above the diaphragm will change the surgery strategy [28]. Furthermore, the detection of visceral metastases appears to be crucial since it has been shown that even patients with metastatic disease might benefit from radical nephrectomy followed by systemic immunotherapy in the case of a good performance status and the presence of lymph node and pulmonary metastases only. Tumor staging is accomplished mainly with CT which allows for assessment of local invasiveness, lymph node involvement, or other metastases [7].
Exceptional circumstances where alternate imaging modalities would be preferred include contrast allergy, functional renal impairment, and pregnancy, where MRI may be used. Furthermore, MRI can be used as a problem-solving modality when the CT findings are nondiagnostic. CT may be followed by MRI if the contrast enhancement at CT is indeterminate or in case of suspected pseudoenhancement. The main MRI feature indicating potential malignancy of a renal tumor is enhancement after i.v. gadolinium administration. However, enhancement at MRI cannot be measured as easily as enhancement at CT [24]. For the differentiation between thrombus and tumor thrombus in the renal vein and inferior vena cava, the use of gadolinium contrast is indicated, since the enhancement of the thrombus indicates a tumor [24]. Preoperative MRI accurately identifies the presence and nature of tumor thrombus with a sensitivity, specificity, and accuracy of 100%, 89%, and 92%, respectively [30]. The role of MRI in RCC imaging is still mainly in differentiating benign lesions versus malignant lesion in patients who cannot undergo CT with i.v. iodinated contrast media or in cases with nondiagnostic CT results [24]. Both CT and MRI perform highly in T-staging of local tumor extent and M-staging of distant metastases. However, both CT and MRI perform relative poorly in N-staging of lymphadenopathy [26, 28].
Current Role of PET in Kidney Cancer
[18F]FDG
Primary Diagnosis. Management of small renal tumors continues to change. and now urologists seek to incorporate new and important information as they plan surgery. Interference of excreted [18F]FDG by the kidneys into the urinary tract accounts for the difficulty in identifying renal cancers that accumulate [18F]FDG but at levels that do not allow discrimination from normal urinary excretory tissue [31]. Various studies have been performed that have confirmed that [18F]FDG PET offers little advantage over conventional imaging in the detection of primary renal malignancy [31–36]. As a result of variable sensitivity that ranges from 40% to 94%, [18F]FDG PET is not used in the detection of primary RCC [31–37]. Kang et al. demonstrated a sensitivity and specificity of 60% and 100% for [18F]FDG PET for the detection of primary RCC (CT: 92% and 100%) [36]. Aide et al. reported in a prospective study (n = 53) that [18F]FDG PET does not offer any advantage over CT for the characterization of renal masses [32]. In the characterization of renal masses, a high rate of false-negative results was observed, leading to a sensitivity, specificity, and accuracy of 47%, 80%, and 51%, respectively, versus 97%, 0%, and 83%, respectively, for CT. However, in this study [18F]FDG PET appeared to be an efficient tool for the detection of distant metastasis in RCC. [18F]FDG PET detected all the sites of distant metastasis revealed by CT, as well as eight additional metastatic sites, leading to an accuracy of 94% versus 89% for CT. Although the role of [18F]FDG PET in the diagnosis of RCC is limited, [18F]FDG PET/CT may be used in both staging and restaging RCC and especially for the identification of visceral and bone metastases.
Recurrent and Metastatic Disease. Early detection and management of metastatic disease has the potential to improve prognosis and quality of life. A meta-analysis found that [18F]FDG can be useful in restaging and detection of metastatic disease, based on its acceptable sensitivity and specificity [38]. Figure 20.2 illustrates an example of recurrent and metastatic kidney and bladder cancer. Safei et al. performed [18F]FDG PET for restaging in 36 patients with advanced RCC and suspected recurrence at distant sites [39]. PET correctly classified 21/25 (84%) of the biopsied lesions (sensitivity and specificity: 88% and 75%). In a small retrospective study (n = 25), Jadvar et al. reported a sensitivity of 71%, specificity of 75%, accuracy of 72%, negative predictive value of 33%, and positive predictive value of 94% for the performance of PET in detection of recurrent and metastatic RCC [40]. Dilhuidy et al. obtained similar results for sensitivity and positive predictive value, i.e., 75% and 92%, respectively [41]. The authors concluded that, when positive, an [18F]FDG PET scan may modify the decision made. When negative, it should not modify decision making especially for surgery, owing to its low sensitivity. A detailed analysis of sensitivity and specificity of [18F]FDG PET versus CT was reported by Kang et al. in a study including 66 patients with suspected or known RCC [36]. For retroperitoneal lymph node metastases and/or renal bed recurrence, the sensitivity and specificity for [18F]FDG PET were 75.0% and 100.0% and for CT 92.6% and 98.1%, respectively. PET had a sensitivity of 75% and a specificity of 97.1% for metastases to the lung as compared to 91.1% and 73.1%, respectively, for chest CT, while PET had a sensitivity of 77.3% and specificity of 100% for bone metastases as compared to 93.8% and 87.2% for combined CT and bone scans. Overall, the specificity of PET was generally higher than that of any other test or a combination of CT and bone scan (for bone lesions). One limitation of the study is that a PET scanner was used and not a combined PET/CT scanner, which is more accurate in staging malignancies.
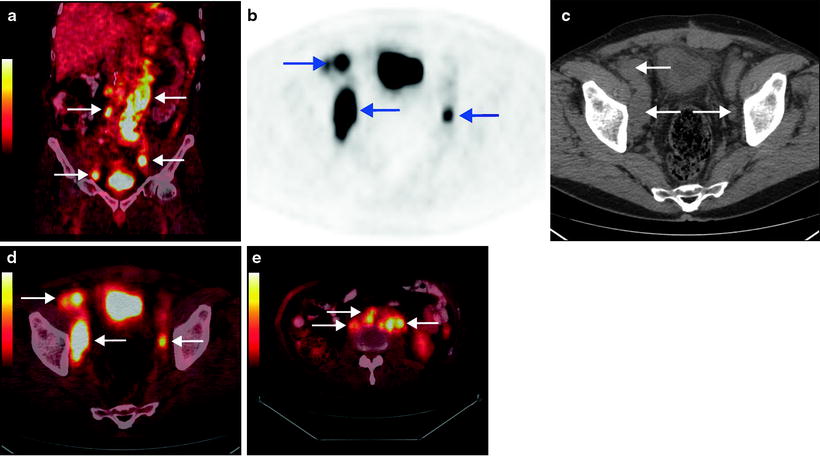
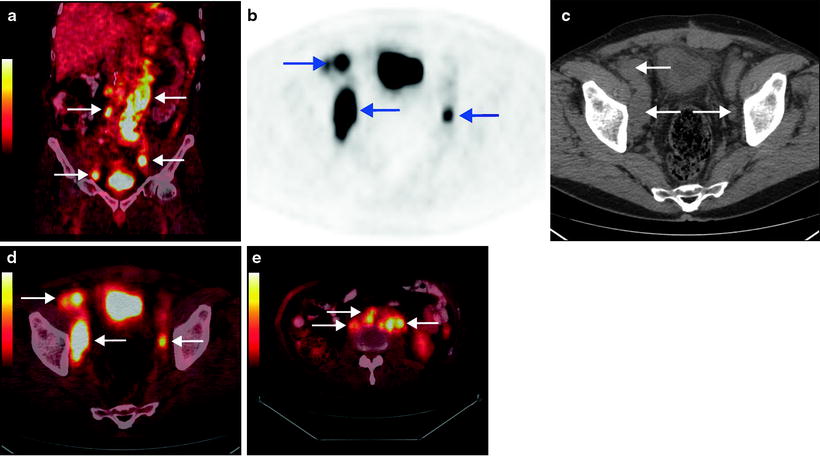
Fig. 20.2
Bladder cancer and renal cancer. Follow-up after treatment. Images from a patient with a history of bladder cancer with lymph node metastases initially treated with chemotherapy. Six years later, the right kidney was removed because of renal cell carcinoma. The patient was referred to [18F]FDG PET/CT 6 months after the operation in order to obtain a status of both the bladder and renal cancer. Coronal fused image shows several foci with increased [18F]FDG uptake in lymph nodes in the abdominal and pelvic area (a). Transaxial PET (b), CT (c), and fused (d) images show foci with increased [18F]FDG uptake in regional lymph nodes at both sides of the bladder. Several retroperitoneal lymph node metastases are seen on transaxial fused image (e)
In a recent large study by Park et al., a hybrid PET/CT scanner and [18F]FDG was used in the follow-up (mean 24.3 months, range 4–88 months) after surgical treatment of 63 patients with advanced RCC [42]. The [18F]FDG PET/CT accurately classified the presence of a recurrence or metastasis in 56 (89%) patients. [18F]FDG PET/CT had an 89.5% sensitivity, 83.3% specificity, 77.3% positive predictive value, 92.6% negative predictive value, and 85.7% accuracy in detecting recurrence or metastasis, which was not significantly different from the results with conventional methods (chest radiography, abdominopelvic CT, and whole body bone scan). The conventional methods had a sensitivity, specificity, PPV, NPV, and accuracy of 94.7%, 80.0%, 75.0%, 96.0%, and 85.7%, respectively. The authors concluded that for the surveillance of high-risk RCC, [18F]FDG PET/CT had results that were as good as conventional methods. Furthermore, they concluded that [18F]FDG PET/CT appears to be an effective surveillance tool, with the advantage of coverage of the entire body in one procedure and no risk of renal functional damage or allergy to contrast agents and with good performance.
Assessment of Response to Therapy. Recently, Vercellino et al. evaluated the accuracy of [18F]FDG PET/CT in assessing early response to sunitinib treatment of 12 patients with metastatic RCC [43]. Patients had an [18F]FDG PET/CT at baseline and another one for follow-up at the end of the first cycle (at day 42). For each examination, all lesions were registered and the maximum standardized uptake value (SUVmax) was measured. The metabolic response on PET was assessed (using European Organization for Research and Treatment of Cancer criteria), and morphologic response on CT at day 84 after two cycles (using Response Evaluation Criteria in Solid Tumors) was used as the reference standard. Early PET/CT findings, after one cycle of sunitinib, were consistent with later CT results in 9 patients of 11 assessable patients: 1 patient progressed on PET and CT, 7 patients had stable disease, and 1 had a partial response. The other 2 patients had a metabolic partial response on PET and stable disease on CT. However, one of these patients achieved a partial response later in follow-up. The results indicate that [18F]FDG PET/CT may be used for the early evaluation of response to sunitinib in metastatic RCC. However, larger studies are needed to evaluate the role of [18F]FDG PET/CT in the assessment of response to sunitinib treatment.
[11C]Acetate
New radiotracers based upon compounds that are selectively accumulated by RCC or radiopharmaceuticals, which are not excreted in the urine, may be useful for imaging of RCC. Thus, other PET tracers than [18F]FDG have been used in RCC in the past. The potential of [11C]acetate as a metabolic tracer for RCC has been investigated in three small studies [44, 45]. Acetate is converted to acetyl coenzyme A in the mitochondria, followed by rapid clearance as carbon dioxide through the citric acid cycle. In a study including 18 patients with RCC, Shreve et al. reported increased uptake of [11C]acetate in renal malignancies, with no significant urinary excretion into the urinary tract [45]. In a recent study, Oyama et al. reported similar results [44]. In this study, 20 patients with suspected renal tumors were included, one of whom had 3 lesions. The [11C]acetate PET results were compared to CT and histology from surgery. In 18 patients (18/20), 20 tumors were diagnosed as RCC. Of the 20 RCC, 14 (70%) showed positive PET findings, and 6 were negative. The authors concluded that [11C]acetate is a possible tracer of RCC. In contrast, Kotzerke et al. reported that in 18 patients with suspected renal tumors, the [11C]acetate accumulation in most kidney tumors was not higher than in normal kidney parenchyma [46]. In the study, 21 patients underwent [11C]acetate PET. Tumor localization was known from CT/MRI. Histology was available in 18/21 patients. The results in these 18 patients were reported. Thus, the results on [11C]acetate are conflicting and larger prospective studies are needed to clarify the role of [11C]acetate PET/CT in RCC.
18F-MISO
Recently, radiolabeled nitromidazoles, 18F-fluoromisonidazole (18F-MISO), have emerged as noninvasive techniques for assessing tumor hypoxia. Nitromidazoles are effective against organisms thriving in hypoxic environments and are being within hypoxic yet metabolically active cells. Thus, 18F-MISO has been used in a number of malignant tumors, where hypoxia has been identified as a risk factor in the prognosis of the tumors. Because RCC is resistant to radiation and chemotherapy, it behaves like a tumor that contains hypoxic cell populations. Lawrentschuk et al. used 18F-MISO in 11 patients with RCC and in four patients with other tumors in order to assess regional hypoxia in renal tumors [47]. A trend of increased 18F-MISO uptake in RCC was demonstrated in RCC tumors as compared to normal tissue. However, although 18F-MISO scans showed significant uptake in other solid tumors, there was only mild 18F-MISO uptake in the present RCC.
18F-Fluorothymidine
Another new tracer in RCC, 18F-fluorothymidine (18F-FLT), which is a radiopharmaceutical based on the nucleic acid thymidine and is able to measure cellular proliferation, has been used in a single case report with a difficult case of renal TCC in a long-standing cyst [48]. Recently, Wong et al. evaluated the uptake of 18F-FLT in RCC (n = 27 patients) and compared to [18F]FDG uptake and immunohistochemical analysis of cellular proliferation (Ki-67) [49]. All 27 patients had preoperative [18F]FDG and 18F-FLT PET scans. A strong correlation was found between both 18F-FLT and [18F]FDG uptake in RCC and Ki-67 on histopathology. The mean SUVmax for 18F-FLT and [18F]FDG in tumors were 2.53 and 2.60, respectively. The results indicate that 18F-FLT may allow for prediction of more aggressive phenotypes of RCC. However, larger studies are needed to evaluate the role of 18F-FLT in RCC.
124I-cG250
Immuno-PET involves radiolabeling of monoclonal antibodies (mAbs) with positron emitters that bind to an antigen on tumor cells. In recent years, much focus has been on the antibody cG250, which reacts against carbonic anhydrase IX (CAIX). CAIX is overexpressed in clear cell RCC, and the G250 antigen is expressed on the cell surface of more than 90% of clear cell RCC and is not expressed in normal kidney [50–53]. CAIX is involved in cellular pH regulation and has been implicated in some pathogenic processes including tumor progression and is thought to play a role in regulating cell proliferation in response to hypoxic condition [54]. High CAIX expression has been demonstrated in malignancies (renal, ovarian, colorectal, lung, brain, and bladder cancers). CAIX is associated with hypoxia and appears to be a marker of poor prognosis [55]. CAIX expression is regulated by the hypoxia-inducible factor 1α (HIF-1α). Mutation of the VHL genes leads to accumulation of HIF-1α and activation of downstream targets including CAIX [56]. Now CAIX is the best and most powerful diagnostic and predictive marker of clear cell RCC [52, 55].
The half-life of common PET tracers, 11C (20.4 min) and 18F (109.7 min), is in general too short for use with mAB in vivo. Positron emitters with longer half-lives, like 124I (4.2 days), are better suited to the slow pharmacokinetics of radiolabeled mAbs. The optimum uptake of such antibody conjugates in tumors is normally several days for intact immunoglobulins [57]. Recently, 124I-labeled antibody chimeric G250 (cG250) has been used to evaluate primary RCC. Divgi et al. assessed whether 124I-cG250 PET could predict clear cell RCC in 26 patients with renal masses who were scheduled to undergo surgical resection by laparotomy [58]. 124I-cG250 PET accurately identified 15 of 16 clear cell RCC, and all nine non-clear-cell renal masses were negative for the tracer (Fig. 20.3). The sensitivity of 124I-cG250 PET for clear cell RCC in this pilot trial was 94% (95% CI 70–100%). The negative predictive value was 90% (55–100%), and specificity and positive predictive accuracy were both 100% (66–100% and 78–100%, respectively). 124I-cG250 PET shows promising results in distinguishing clear cell RCC from other subtypes. Preoperative identification of tumor type could have important implications for the choice of treatment for renal cancers. Thus, 124I-cG250 PET may be a useful diagnostic imaging tool in the clinical management of renal masses. Imaging with 124I-cG250 PET has the potential to change clinical management, in patients where a conservative approach (renal impairment, comorbid disease) may be appropriate in G250 negative tumors. These patients may be selected for nephron-sparing surgery or observed. It has been proposed that a 124I-cG250 PET scan may be useful as an alternative to biopsy. However, this has to be evaluated further in larger clinical trials.
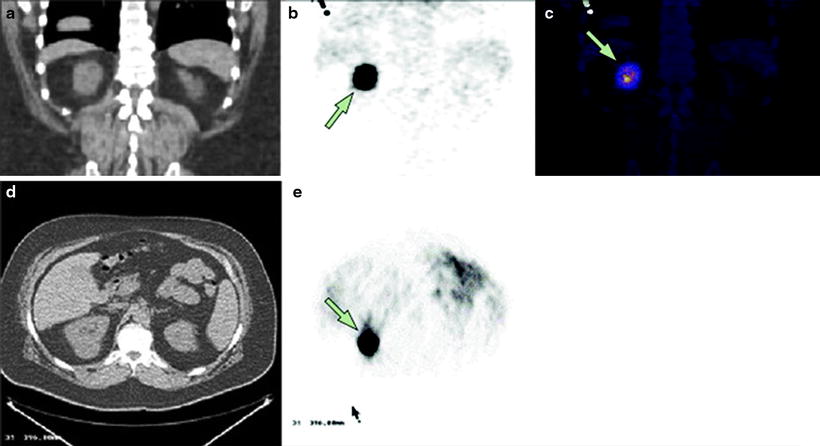
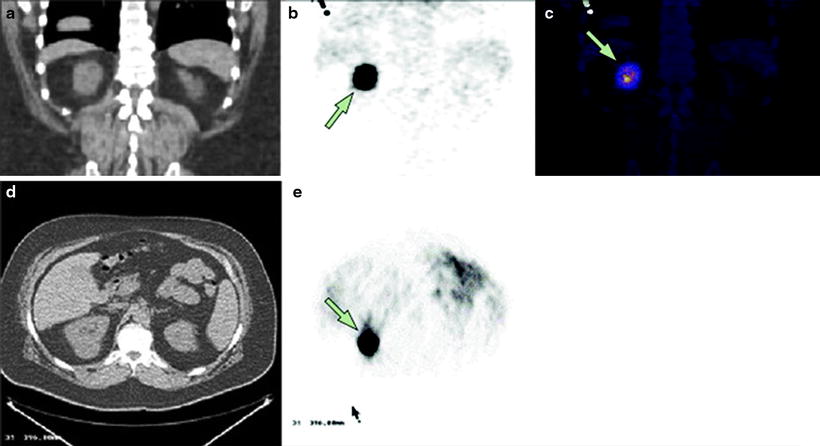
Fig. 20.3
124I-cG250 PET/CT imaging of clear cell renal carcinoma. Images from a patient with biopsy-proven clear cell renal carcinoma: representative coronal CT (a), PET (b), and fused image (c), representative transaxial CT (d), and 124 I-cG250 PET (e) images. (From Divgi CR, Pandit-Taskar N, Jungbluth AA et al. Preoperative characterization of clear-cell renal carcinoma using iodine-124-labeled antibody chimeric G250 (124I-cG250) and PET in patients with renal masses: a phase I trial. Lancet Oncol. 2007;8:304–310, with permission from Elsevier)
Radioimmunotherapy of Kidney Cancer
RCC is a radio- and chemotherapy-resistant tumor, which has a high morbidity and mortality when metastasized. The current treatment options demonstrate limited efficacy and severe side effects. Therefore, there is a need for new therapeutic strategies for RCC. The most important alternative to conventional therapy is the targeted tumor therapy where selective molecules are used to direct the anticancer drugs to the tumor to prevent damage in healthy tissues. For targeted therapy, peptides and antibodies possess a key position as drug vectors [59]. Antibodies bind with high affinity and specificity to cell-surface antigens and do not need to be internalized to evolve their effect. This results in minimal toxicity to normal tissues. For efficient tumor therapy, the favorable targeting properties of antibodies and peptides must be combined with a cell-toxic agent. For this requirement, radioactive nuclides are especially suitable. Thus, endoradiotherapy combines the favorable targeting properties of peptides and antibodies with the effect of radiation-induced cell death. Furthermore, the drugs that are applied cannot only be labeled with therapeutic nuclides but also with diagnostic ones. This allows imaging and dose estimation of the compound prior to treatment and thus a levelheaded therapy planning. The targeted therapy generally causes moderate side effects. The side effects are mostly pronounced in the organs involved in the clearance of the drug (kidney and liver), and the dose-limiting toxicity of delivering high-dose RIT is generally hematological [51, 60]. The most common use of radiolabeled antibodies is the treatment of multiple metastatic diseases. Furthermore, antibodies are very efficient in the therapy of medullary hematological malignancies and myoablation [61].
The first mAbs being investigated for radioimmunoscintigraphy and radioimmunotherapy (RIT) were murine antibodies, which can provoke an immune response in humans. As a result, human anti-mouse antibodies (HAMAs) are almost invariably formed, which on repeating administrations, complex the circulating mAbs resulting in an accelerated clearance from the blood. Thus, the production of HAMAs inhibits the effectiveness of the second administrated dose of radiolabeled mAbs [60]. When using chimeric antibodies and humanized antibodies, the immune response is reduced in the patients. The most commonly used radionuclides for RIT are beta-emitters, but Auger and alpha-emitters can also be used. Iodine-131 (131I), yttrium-90 (90Y), and luthetium-177 (177Lu) are the most commonly used beta-emitters in RIT [60]. These radionuclides differ with respect to physical half-life, the presence or absence of gamma rays, the energy of the β-particles, and the range of the β-particles in tissue [62]. For minimal cancer disease (tumor only few mm in diameter), medium energy β-emitters, such as 131I and 117Lu, may be the best choice while in larger tumors high energy β-emitters, such as 90Y, are more suitable. RIT has been most efficacious in patients with hematological malignancies, especially in patients with non-Hodgkin’s lymphoma (NHL). In 2002 and 2003, the first two radiolabeled murine anti-CD20 mAbs were approved for clinical use by the FDA: 90Y-Ibritumomab (Zevalin®, Biogen, IDEC, Cambridge, MA) and 131I-Tositumomab (Bexxar®, Corixa, Seattle, WA, USA) [60].
Several clinical studies have been conducted on the feasibility, toxicity, and efficacy of RIT using radiolabeled mAb G250. In a phase I/II RIT study, escalating activity doses of 131I-G250 were administrated to patients with metastatic RCC [63]. Seventeen out of 33 patients showed stabilization of disease progression. However, the murine G250 mAb was highly immunogenic since all the patients developed an HAMA response. After development of the chimeric form of G250, 131I-cG250 was used in studies [64–66]. The maximum tolerated dose (MTD) of 131I-cG250 in metastatic RCC was determined in a phase I radioactivity dose escalation trial in patients with advanced metastatic RCC [67]. The MTD was determined to be 2,220 MBq/m2, with hematological toxicity as the dose-limiting factor. Fractionation of the dose was performed in a phase I study by Divgi et al. [66]. Steffens et al. investigated the effect of two sequentially high doses of 131I-cG250 [65]. Of the 18 patients evaluated (3 not receiving the second RIT because of MTD), five patients had stabilization of their disease, lasting 3–12 months. No partial or complete responses were seen in the study. In order to optimize cG250 RIT of RCC, the therapeutic properties of cG250 labeled with four radionuclides were tested in nude mice with human RCC xenografts [68]. Tumor growth was delayed most effectively by 177Lu, followed by 90Y and 186Re and least by 131I. The different nuclear properties of these four radionuclides are shown in Table 20.2. The results from the in vivo study indicate that 177Lu and 90Y should be considered better candidates for RIT with cG250 than 131I. Currently, a phase I/II therapy trial is ongoing, investigating the efficacy of 177Lu-DOTA-cG250 in patients with advanced RCC (NCT00142415). Preliminary results demonstrated excellent tumor targeting of RCC lesions and indicated that 177Lu-cG250 treatment could stabilize previously progressive metastatic RCC [69]. Other strategies may be used to improve the clinical efficacy of cG250, i.e., tumor pretargeting, high-dose RIT with bone marrow support or transplant, or use of high linear energy transfer (LET) particles emitting radionuclides [51].
Table 20.2
Nuclear properties of radionuclides used in radioimmunotherapy of RCC
Nuclide | Half-life (days) | Average β energy (Mev) | Average range (mm) | Photon radiation (keV) |
---|---|---|---|---|
131I | 8.0 d | 0.181 | 0.91 | 364 (82%) |
177Lu | 6.7 d | 0.133 | 0.67 | 113 (6%) |
186Re | 3.7 d | 0.362 | 1.8 | 208 (11%) |
90Y | 2.7 d | 0.935 | 3.9 | 137 (9.4%) |
Bladder Cancer
Clinical Background
Bladder cancer is the most common cancer of the urinary system and is the fourth most common malignancy in men, following prostate, lung, and colon cancer [6]. Bladder cancer incidence is increasing. However, the mortality rate has somewhat stabilized. Incidence peaks in the sixth and seventh decades of life, although a trend toward presentation at younger ages has been suggested [70]. Because of long-term survival and the need for lifelong routine monitoring and treatment, the cost per patient of bladder cancer from diagnosis to death is the highest of all cancers in USA [71]. Rates in males are three to four times those in females [72]. Incidence rates are high in many southern and eastern European countries, in parts of Africa and the Middle East, and in North America [72]. TCC accounts for over 90% of cases and is by far the most common epithelial tumor of the bladder [73]. The second most common cell type is squamous cell cancer (5%), and less than 2% are adenocarcinomas [70]. Several causative risk factors are associated with urothelial tumors. Of these, smoking is one of the most important factors and is consistently associated with the development of TCC. Cigarette smoking increases bladder cancer risk two- to fourfold, and 30–50% of all bladder cancer is caused by cigarette smoking [74]. The latency period is approximately 20–30 years. Painless microscopic or gross hematuria is the initial symptom in over 80% of the patients with urothelial cancer of the bladder, which is why TCC needs to be excluded in patients presenting with these symptoms [74].
Diagnosis
The standard method of diagnosing bladder cancer continues to be based on direct visualization of the bladder with cystoscopy and subsequent biopsy/resection [75, 76]. In an effort to improve the sensitivity of bladder cancer diagnosis, fluorescence cystoscopy continues to evolve. Several prospective randomized clinical trials have demonstrated increased detection of bladder tumors, particularly Ta tumors and carcinoma in situ (CIS) when using fluorescence cystoscopy [77 78]. One of the distinguishing features of TCC is that it has the highest recurrence rate for any cancer, thereby making rigorous posttherapeutic surveillance, often referred to as “urothelial surveillance,” necessary in all patients [79]. These tumors are also commonly multifocal. They involve any part of the urothelium in a seemingly random manner. Biomarkers: Despite the development of several commercially available urinary markers for diagnosis and surveillance of bladder cancer, urinary cytology continues to play an important role in the diagnosis and surveillance of bladder cancer [80]. The specificity of cytology approaches 100%, whereas other markers generally outperform cytology with respect to sensitivity, especially for low-grade disease [75].
Treatment of Superficial Bladder Cancer
Most bladder cancers are “non-muscle invasive” at the time of initial diagnosis. Roughly 70% of newly diagnosed bladder cancer is superficial disease [74]. They are either confined to the mucosa (stage Ta, CIS) or invade the lamina propria but not the muscularis propria (stage T1). Among the superficial cancer group, approximately 70% presents as Ta lesions, 20% as T1, and 10% as CIS [74]. Stage Ta tumors, especially those which are low grade, have a low risk of progression over time, and there is a trend toward more conservative management of these tumors. Therefore, in the majority of these patients, management can be limited to resection and subsequent observation [81]. Stage T1 tumors represent greater challenge to the urologist. Although these tumors are grouped into the category of “superficial,” their risk of progression is much higher over time. The heterogeneity of T1 disease complicates its treatment. It remains a challenge to determine which patient is at high risk of progression versus the patients who will do well with adjuvant intravesical therapy. The European Association of Urology (EAU) and American Urological Association (AUA) have similar recommendations for induction and maintenance treatment in patients based on clinical and pathologic risk factors [81, 82]. Low-risk patients (low-grade Ta) can be monitored on surveillance protocols. Conversely, those patients with high-risk superficial disease (CIS, T1, high-grade Ta, multiple tumors, and > 3-cm tumors) should strongly be considered for adjuvant intravesical therapy. The most widely used agents include the immunotherapy drugs Bacillus Calmette-Guérin (BCG), interferon alpha (IFN-α), and the chemotherapy agent mitomycin C (MMC), among others [82]. BCG represents the only agent known to reduce progression into muscle-invasive bladder cancer [82]. BCG has proven superiority over intravesical chemotherapy with respect to CIS but not for papillary tumors alone [76, 83]. Despite the efficacy of BCG, a substantial number of patients recur or progress. Thus, several studies have used novel agents as well as combination therapy utilizing BCG as a component in an attempt to delay tumor recurrence/progression yielding mixed results [75, 76].
Treatment of Muscle-Invasive Disease
The primary modality for treatment of clinically localized muscle-invasive bladder cancer is surgery, and developments in this field focus on minimizing the morbidity of surgery. Radical cystoprostatectomy with bilateral pelvic lymphadenectomy remains the gold standard for management of muscle-invasive bladder cancer [75, 76]. However, the precise limits of that lymph node dissection are debated [75]. Data from a large group of patients support the aggressive surgical management of invasive bladder cancer [84]. Long-term disease-free survival of up to 70% can be achieved in most patients with organ-confined disease [75, 84]. Bladder preservation strategies (TUR, chemotherapy and radiation therapy, individually or in combination) are sometimes used instead of cystectomy to limit treatment-associated morbidity especially in patients who are unwilling or not medically suited to undergo radical surgery. Neoadjuvant and adjuvant chemotherapy have been utilized in an attempt to improve outcomes for patients with high-risk muscle-invasive disease. Systematic chemotherapy using multidrug regimens is the standard therapy for metastatic disease, and the classic combination is the four-drug regimen MVAC (methotrexate, vinblastine, doxorubicin, and cisplatin) [76, 85, 86].
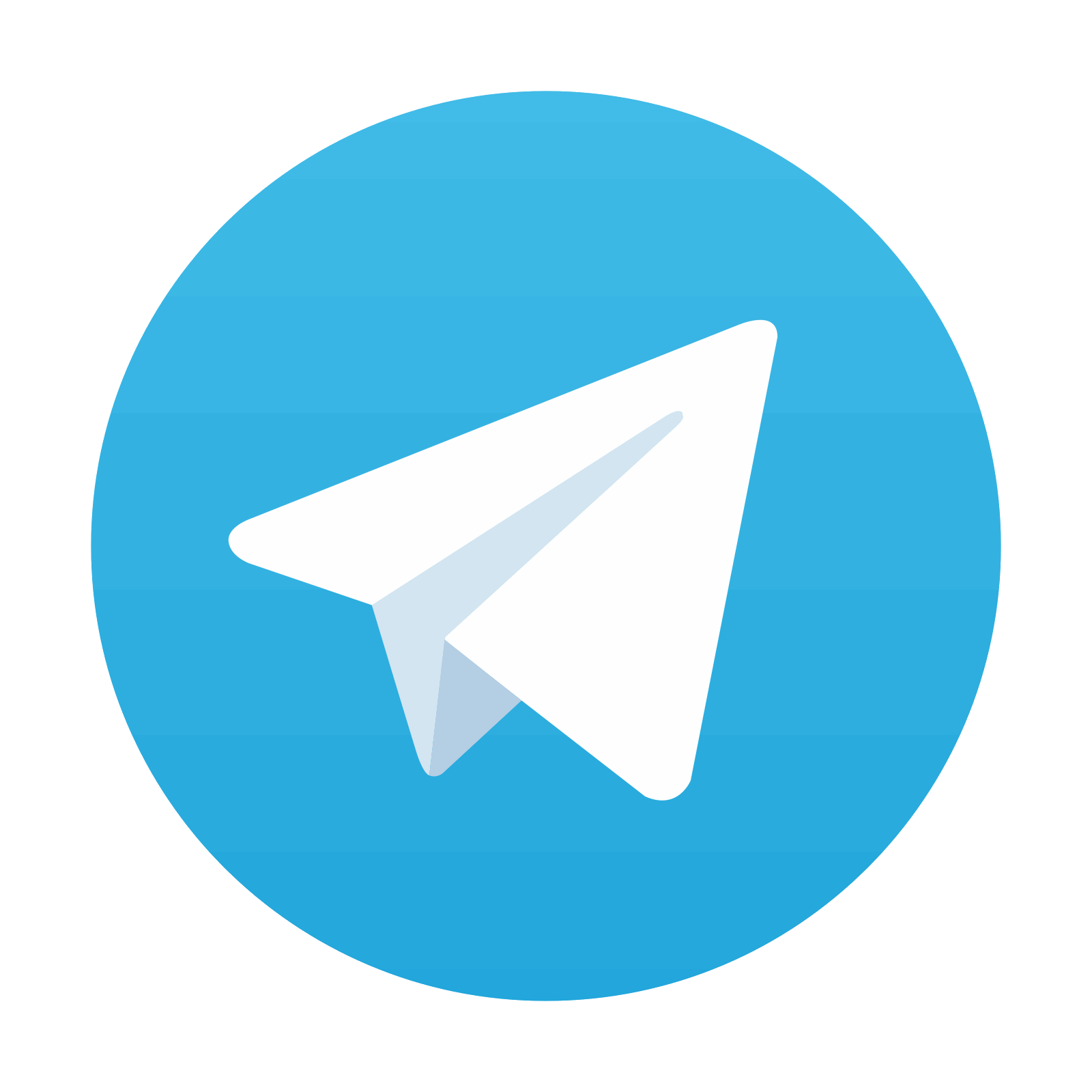
Stay updated, free articles. Join our Telegram channel

Full access? Get Clinical Tree
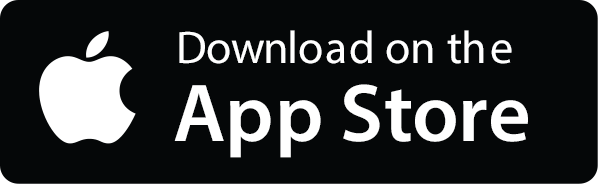
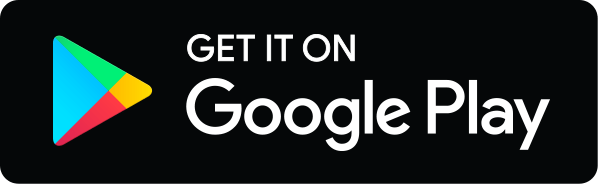