Factors
IPS [21]
Male gender
Age >45
Stage 4
Hemoglobin <10.5 g/L
WBC count >15 × 109/L
Lymphocyte count <0.6 × 109/L or <8% WBC
Serum albumin <40 g/L
Risk increasing with each increase in number of adverse factors
IPI [62]
Age >60
Elevated serum LDH
ECOG performance status ≥2
Ann Arbor clinical stage 3 or 4
>1 Extranodal disease sites
# of factors
Risk group
0–1
Low
2
Low intermediate
3
High intermediate
4–5
High
Revised IPI [63]
Same as IPI
# of factors
Risk group
0
Very good
1–2
Good
≥3
Poor
FLIPI [69]
Age >60
Elevated serum LDH
Ann Arbor clinical stage 3 or 4
>4 Involved nodal disease sites
Hemoglobin level <12.0 g/dL
# of factors
Risk group
0–1
Low
2
Intermediate
≥3
High
Treatment and Follow-Up
The advent of multiagent chemotherapy in the 1960s beginning with MOPP (mechlorethamine, vincristine, procarbazine, and prednisone) has led to excellent overall response rates and cure of disease in many patients [23–26]. Cure rates are >90% for patients with early stage and favorable disease, but they are in the 65–70% range for advanced disease. A complete discussion of the evolution of therapy for HL is beyond the scope of this chapter, but general management strategies are presented to place the role of imaging into the therapeutic context.
The treatment course for early stage HL is combined chemotherapy and involved field radiation [27, 28]. The number of cycles of chemotherapy is guided by the favorable and unfavorable risk groupings. The course of chemotherapy is brief (2–4 cycles) for early stage, favorable disease and typically longer (6 cycles) for unfavorable disease [18, 27]. For both early stage favorable and unfavorable disease, the current chemotherapy regimen of choice based on efficacy and toxicity profiles is ABVD (adriamycin, bleomycin, vinblastine, dacarbazine) [8, 27, 29–32].
For early stage, favorable disease, there is no benefit of wide-field or subtotal nodal radiation over involved field radiation (IFRT, typically 30 Gy) when combined with chemotherapy [33]. Studies continue to investigate the optimal chemotherapy regimens, reduction of chemotherapy cycles, and radiation therapy doses in order to reduce toxicity. In attempts to reduce toxicity, the German Hodgkin’s Study Group (GHSG) H13 study is investigating variations of ABVD, omitting individual drugs (ABV versus AV versus AVD), for patients with early stage favorable disease. Two of the arms (ABV and AV) were closed early due to inferior outcomes [8]. The GHSG H10 trial randomized patients to 2 versus 4 cycles of ABVD and 20 versus 30 Gy of involved field radiation. The second interim analysis results were presented in 2004, and no differences in freedom from treatment failure or overall survival were seen [34]. The final results of this study are awaited.
Chemotherapy combinations and radiation doses are subjects of clinical trials for early stage unfavorable HL to improve the relatively low relapse rate (∼15%) and the primary refractory (∼5%) rates [27]. Comparisons of ABVD to more intensive BEACOPP (bleomycin, etoposide, doxorubicin, cyclophosphamide, vincristine, prednisone, and procarbazine) have yielded similar efficacy results between the regimens. Significantly more toxicity has been found with BEACOPP, so it has not been recommended for early stage disease [27, 35, 36]. The Stanford V regimen (which also includes radiation) is an option for those with unfavorable early disease; however, the original treatment schema should be closely followed [18, 37, 38]. Chemotherapy alone is an option for patients with early stage (favorable and unfavorable) disease depending on individual patient characteristics due to potential late term complications of radiation [18].
For advanced HL, ABVD has been shown to be superior to MOPP and hybrid regimens [30–32], and is typically given for 6 cycles. As with early, unfavorable HL, more intensive regimens are being investigated and compared to ABVD. The GHSG trials have demonstrated better outcomes with escalated BEACOPP than with ABVD [36], but more acute toxicity and secondary leukemias were seen. Escalated BEACOPP is more widely used in Europe, and NCCN guidelines recommend that escalated BEACOPP is an option for advanced HL, with IPS >4 [18]. It is not recommended for elderly patients with advanced HL due to acute toxicity [39]. Results from several randomized phase III trials are awaited: the European Organization for Research and Treatment of Cancer (EORTC) trial number 20012 comparing ABVD (8 cycles) to BEACOPP (4 escalated + 4 standard) and the Eastern Cooperative Oncology Group (ECOG) comparing ABVD to the Stanford V. The role of radiation therapy to areas of bulky disease in advanced HL is also still uncertain and under investigation.
About one-third of patients with HD are refractory to or relapse after initial therapy, and often receive high-dose chemotherapy and stem cell transplant [40]. Despite high response rates posttransplant [41–44], a significant number, 26–65%, of these patients relapse [37, 40, 43–46]. Relapse or decreased survival posttransplant has been associated with a number of risk factors including poor performance status at transplant, chemotherapy-resistant disease, increasing number of failed chemotherapy regimens, more than minimal or bulky disease at transplant, B symptoms at relapse, and extranodal disease at relapse [37, 40, 43–46]. Posttransplant, therapy options are limited due to patients’ decreased bone marrow reserve. Single-agent chemotherapy, local irradiation, or reduced intensity chemotherapy followed by allogeneic stem cell transplant can be considered, but therapy is most often given with palliative intent. This group is also suitable for early phase clincial trials.
LpHL has a different, more indolent natural history, and late relapses are more likely to occur compared to cHL [47]. Some consider lpHL to behave more like low-grade NHL. For patients without “B” symptoms, early stage disease is treated with radiation therapy alone. Chemotherapy plus IFRT is used for stage IB and IIB disease. Radiation may or may not be included with chemotherapy for treatment of advanced lpHL, There is no standard chemotherapy regimen for lpHL due to a lack of randomized controlled trials comparing different regimens [18]. Because lpHL routinely expresses the CD20 antigen, rituximab used as a single agent has been studied and very encouraging response rates have been reported [48–50]. Additional follow-up is needed to further assess the durability of the responses.
Data are limited regarding optimal follow-up of patients with HL after therapy. Generally, history, physical exam, and laboratory evaluations are performed every 2–4 months for 2 years after treatment, then every 3–6 months until year 5 and annually, thereafter. Thoracic imaging is performed every 6–12 months for 2–5 years then annually, while abdominal/pelvic imaging is less frequent [18]. The use of PET for surveillance is discussed further below. Additional careful follow-up is needed to monitor for late effects of treatment.
As shown above, very important considerations in treating patients with HL are the long-term side effects of radiation (e.g., hypothyroidism, premature menopause, carotid and coronary artery stenosis, and secondary breast cancer) and chemotherapy (e.g., infertility, cardiomyopathy, secondary leukemia, and osteoporosis) [8]. Increased risk of death persists for more than 25 years in patients with HL due to late term treatment complications rather than HL itself [51, 52]. The results of the ongoing clinical trials will help guide further management strategies. In addition, strategies using interim PET for risk-adapted therapy may be advantageous for this purpose and for guiding dose intensification for those patients who are more likely to relapse and dose deintensification in those responding well. This is discussed in the section on PET and risk-adapted therapy.
Non-Hodgkin’s Lymphoma
Epidemiology and Etiology
In 2009, the estimated number of new cases of NHL in the USA was 65,980, and NHL was the sixth most common cancer in men and the fifth most common in women [3]. NHL resulted in about 19,500 deaths from the disease in 2009—ranking as the ninth and sixth most common cause of cancer deaths for men and women, respectively. The incidence of NHL has been rising since the 1970s, most markedly in the elderly population. The overall increase in incidence is out of proportion to that expected due to the aging population alone and the reasons for this are mostly unclear. Despite this, the death rate from NHL has been decreasing since 1997 due to improvements in therapy [3]. Most cases of NHL occur in individuals greater than 60 years of age.
Alterations of the immune system, certain viruses, and environmental exposures have been implicated in the etiology of NHL, with varying degrees of evidence. For example, both immune suppression (e.g., posttransplant) and immune stimulation (e.g., Sjogren’s syndrome, rheumatoid arthritis) have been associated with increased risk of NHL [53–55]. Viral-lymphoma associations have also been suggested. Environmental exposures linked to NHL include organochlorine-based pesticides, organic solvents, and wood products.
Pathology and HL Subtypes
NHL consists of a heterogeneous group of diseases that arise from malignant transformation of lymphoid tissues. Initial classification schemes were based primarily on morphology. Advancements in immunological, molecular, and genetic laboratory techniques have led to several updates and revisions of classification systems for NHL [56–58]. The ability to identify cell surface markers, cytogenetic and molecular markers led to the recognition that subtypes of NHL depend on the stage of the maturation when malignant transformation of the lymphocyte occurs as well as the location of the “normal lymphocyte counterpart” within the lymph node.
The currently used World Health Organization classification uses morphologic, immunophenotypic, genetic, and clinical features to define distinct NHL subtypes and was revised in 2008 [57, 58]. The revision further defined and refined definitions of early/in situ lesions, the relevant distinctions in subtypes based on age, and borderline diagnoses (hard to classify as one subtype versus another due to atypical or overlapping features). This is nicely reviewed by Jaffe [57]. The NHL subtypes are grouped by lineage (B cell or T cell/natural killer (NK) cell) and the maturity of the cell from which the malignancy is derived (Table 7.2). Most (80–85%) are B-cell neoplasms [9]. Generally, NHL may behave in a manner that is indolent, aggressive, or very aggressive. The aggressiveness of a tumor within a histologic subtype can be variable between patients, so it is not included in the official classification scheme.
B-cell lymphomas | T/natural killer-cell lymphomas |
---|---|
Precursor B lymphoblastic lymphoma/leukemia | Precursor T-cell lymphoblastic lymphoma |
Mature B-cell lymphoma | Mature T-cell lymphoma |
Chronic lymphocytic leukemia/small cell lymphoma | Adult T-cell lymphoma/leukemia |
Lymphoplasmacytic lymphoma | Mycosis fungoides (Sezary syndrome) |
Splenic marginal zone lymphoma | Primary cutaneous anaplastic large cell lymphoma |
MALT lymphoma | Anaplastic large cell lymphoma |
Normal marginal zone lymphoma | Peripheral T-cell lymphoma, unspecified |
Follicular lymphoma | Angioimmunoblastic T-cell lymphoma |
Mantle cell lymphoma | Extranodal NK/T-cell lymphoma, nasal type |
Diffuse large B-cell lymphoma | Enteropathy-type T-cell lymphoma |
Mediastinal (thymic) large B-cell lymphoma | Hepatosplenic T-cell lymphoma |
Intravascular large B-cell lymphoma | Subcutaneous panniculitis-like T-cell lymphoma |
Primary effusion lymphoma | Blastic NK cell lymphoma |
Burkitt’s lymphoma/leukemia |
Clinical Presentation, Evaluation and Staging, and Prognosis
NHL most commonly presents as painless adenopathy, similar to HL, but the rapidity of tumor growth and the exact presentation varies with the histologic subtype and aggressiveness of the tumor. NHL spreads in a manner less predictable than HL (more hematogenously than by contiguous nodal spread) and is more likely to involve extranodal sites than HL. Systemic “B” symptoms are found in 40% of patients, more common in the aggressive than indolent NHL subtypes [59]. Lymphomatous infiltration of the bone marrow may result in cytopenias, and the presentation of infiltration of other organs may be site specific.
A core or excisional biopsy is usually needed for diagnosis, in order to provide sufficient tissue. Fine needle aspiration alone and cytology often do not provide enough tissue for morphologic, immunologic, and genetic assessment. After diagnosis, the initial workup is similar to that of HL. History and physical exam, laboratory tests, and imaging are performed for staging and assessing organ function prior to treatment. Staging is best performed with PET for NHL subtypes that are typically [18F]FDG avid (i.e., diffuse large B-cell NHL). Although follicular lymphomas are typically [18F]FDG avid, indolent lymphomas may sometimes have low metabolic activity and in these instances PET for staging may be less helpful. Variability of [18F]FDG uptake and staging with PET in NHL is discussed further below.
Bone marrow biopsy is performed in nearly all patients at diagnosis of NHL, and lymphomatous involvement is found in 30–50% of them, more commonly with the indolent NHL subtypes [60, 61]. Laboratory testing is primarily used for prognostic scoring and to test baseline organ function prior to treatment.
The Modified Ann Arbor Staging System is also used for staging NHL [19, 20]. The Cotswold modification made some changes to improve the relevance of the Ann Arbor Staging System for NHL (versus HL), but a continued limitation was lack of inclusion of total tumor bulk instead of just the number of disease sites. Additional factors are important for determining prognosis and treatment for patients with NHL.
The histopathologic subtype primarily determines the prognosis for patients with NHL. Within each subtype of NHL, disease-specific genetic alterations and molecular markers may provide additional information for predicting outcomes and guiding management. The International Prognostic Index (IPI) was developed in patients with aggressive NHL (Table 7.1) [62]. Four risk groups were identified (low = IPI 0–1, low intermediate = IPI 2, high intermediate = IPI 3, and high = IPI 4–5) with decreasing 5-year overall survival with increasing IPI score (73%, 51%, 43%, and 26%, respectively) [62].
The original IPI was developed and validated prior to the routine use of rituximab in regimens to treat NHL. Sehn et al. [63] retrospectively evaluated the use of the IPI in 365 patients with diffuse large B-cell NHL treated with rituximab, cyclophosphamide, doxorubicin, vincristine, and prednisolone (R-CHOP). Using the original IPI groupings, only 2 (not 4) risk groups were identified. The revised IPI redistributed the risk groups (very good = IPI 0, good = IPI 1–2, poor IPI 3–5), and this model was felt to better predict outcome. In the rituximab era, the risk group with 4-year survival <50% was no longer identified [63].
Additional variations of the IPI have been developed, including age- and stage-adjusted IPIs to account for the variability of prognoses within these groups [62, 64]. The usefulness of the IPI also has been shown to provide prognostic information at the time of relapse [65–68].
There are two versions of the IPI for patients with indolent follicular NHL (FLIPI 1 and 2), since the original IPI was designed and evaluated in patients with aggressive NHL. Using the first FLIPI version, overall survival decreases with increasing FLIPI score based on three risk groups (low, intermediate, and high) and provides prognostic information for this population, even in the setting of rituximab [69, 70]. Validation of FLIPI 2 is ongoing.
Treatment and Follow-Up
As with the natural history and prognosis of NHL, treatment varies and depends on the specific NHL subtype. Detailed management strategies, including recommended chemotherapy regimens, are provided by a panel of experts in the NCCN guidelines [71]. Excellent review articles are available for specific questions and agents for the treatment of NHL, including new biologic agents and treatment strategies [72–77]. We will review overall management strategies for the most common NHLs—diffuse large B-cell NHL and follicular NHL. These are also probably the most commonly encountered by the nuclear medicine physician or radiologist. Discussion of treatment for the other subtypes is beyond the scope of this chapter and can be found elsewhere [71, 78].
Indolent NHL is generally considered incurable at present. Patients respond to therapy but may have multiple relapses and long disease-free or progression-free periods. A major question for those with indolent NHL is when to initiate therapy. Before rituximab, studies did not show an overall survival advantage for early initiation of therapy in asymptomatic patients despite improvements in remission rates and disease-free survival, even in patients with advanced disease [79, 80].
General indications for treatment of indolent NHL are symptoms, organ compromise, rapid or steady progression of tumor, bulky disease, cytopenias due to lymphoma, and patient preference or eligibility for a clinical trial [71]. The treatment plan and aggressiveness of the chemotherapy regimen used should be tailored for the patient’s disease and clinical status. Common regimens include purine analogs, alkylators, anthracyclines, and rituximab alone or in combination with chemotherapy. Zevalin consolidation has also been FDA approved as a first line therapy of NHL.
Sousou and Friedberg nicely reviewed the pivotal studies that investigated the role of rituximab in patients with indolent lymphoma [81]. Treatment with rituximab alone [82–84] and with chemotherapy [85–88] has improved overall response rates and PFS for patients with indolent NHL in both the frontline and maintenance settings [89]. There is a suggestion toward improved overall survival, but longer term follow-up is needed and perhaps more prominently in certain subsets of patients [90, 91]. Areas of continued study for rituximab are used with immune stimulators to improve efficacy, optimal schedule for maintenance dosing, and resistance mechanisms. Other monoclonal antibodies other than CD20 are also being developed and investigated [92].
In the relapsed setting, indolent NHL may be treated in a similar manner to initially untreated disease. Overall response rates to rituximab in the relapse setting range from 40% to 60% with complete responses in 10–20% and median PFS of 8–24 months [84, 93, 94]. RIT is more active and produces better results than unlabeled antibody alone, and its use in relapsed (as well as the frontline) indolent NHL is further discussed below [95, 96]. Autologous and allogeneic transplants have been performed safely and with antitumor activity. If there is a role for transplant in indolent lymphoma, the optimal role is still being defined.
For aggressive B-cell NHL, systemic chemotherapy is the mainstay of treatment. Diffuse large B-cell NHL is the most common aggressive NHL and is discussed as an example. Several commonly used regimens are R-CHOP and R -CVP, with increasing use of R-bendamustine in the United States. [97–99]. For nonbulky early stage disease, IFRT may or may not be added depending on the number of chemotherapy cycles given. Higher doses of IFRT (30–40 Gy) are added to 6–8 cycles of chemotherapy for early stage, bulky disease. The preference at some major centers is to treat patients with advanced disease on a clinical trial; otherwise, a full course of chemotherapy is recommended [71, 97]. Studies continue to investigate various strategies and schedules for administering R-CHOP.
The role of high-dose therapy and transplant for initial therapy of aggressive NHL is controversial and the subject of clinical trials. [18F]FDG PET is being used as a prognostic indicator in several of these risk-adapted therapy trials, and these studies are discussed in the section on risk-adapted therapy. In the relapse setting, there are several salvage chemotherapy regimens that may be used with the ultimate goal of transplant in those patients who respond.
T-cell NHL accounts for ∼15% of all lymphomas. Most T-cell lymphomas are clinically aggressive and with a few exceptions (anaplastic large cell, early stage T/NK cell, nasal) most are incurable. CHOP and CHOP-based therapy may be used for initial treatment in these settings [100]. Other approaches have been investigated for T-cell NHL subtypes including investigations of biologic agents (bevacizumab [101] and histone deacetylase inhibitors [102]) and agents against other lymphocyte surface antigens e.g. CD52 (alemtuzumab [103]).
Follow-up for patients with NHL also depends on the subtype of NHL, treatment intent, and the patient’s clinical symptoms. For those treated with curative intent, history and physical exam, laboratory testing, and imaging occur with decreasing frequency over the first 5 years, and then annually. Patients must be monitored for late complications of therapy, which are similar to those discussed for HL.
Nuclear Medicine Imaging for Lymphoma
Nuclear medicine imaging has had a role in the evaluation of patients with lymphoma since the 1970s [104]. Gallium-67 citrate imaging was shown to be useful for detecting and staging lymphoma [105, 106]. Israel, Front et al., among others, showed that 67Ga-citrate was better than anatomic CT imaging for monitoring response of lymphoma to therapy [107–110]. This was because nonviable but sometimes large residual lesions remained on CT posttreatment. Gallium-67 imaging also provided prognostic information, with a negative scan favoring a better outcome after treatment and as soon as after 1 cycle of chemotherapy [107–111]. Other agents were also evaluated, for example, indium-111 bleomycin, but they were not shown to be more efficacious than gallium-67 [112, 113].
Today, gallium scanning has essentially been replaced with [18F]FDG PET/CT imaging, which offers higher resolution images and higher sensitivity for disease detection, particularly for lower grade lymphomas [113–118]. Bone scanning has also been used for detection of lymphomatous involvement in bone; however, these typically marrow-based lesions may be falsely negative on bone scan. In the PET era, bone scanning is considered if patients present with bone pain and a PET scan is not available [8]. Compared to other numerous malignancies where PET/CT is useful, its various roles for evaluating patients with lymphoma probably make this disease the one where PET/CT is most integrated into management strategies. The current roles of PET (disease detection, staging, intratherapy and posttreatment assessment) will now be reviewed. Numerous ongoing clinical trials investigating the role of interim PET, obtained after 1–4 cycles of chemotherapy, for risk-adapted therapy will then be discussed.
[18F]FDG PET for Detecting and Histologic Grading of Lymphoma
Most lymphomas accumulate [18F]FDG [2]. Weiler-Sagie et al. [119] recently readdressed this issue in a large retrospective study of 766 patients with newly diagnosed lymphoma referred for initial staging with [18F]FDG PET/CT. [18F]FDG-avid disease was found in all patients with HL, 94% with aggressive NHL and 83% with indolent NHL. The histologic subtypes of lymphoma less likely to be [18F]FDG-avid were small lymphocytic lymphoma (SLL) (%[18F]FDG avid—83%), enteropathy-type T-cell lymphoma (67%), extranodal marginal zone lymphoma (67%), MALT marginal zone lymphoma (54%), lymphomatoid papulosis (50%), and primary cutaneous anaplastic large T-cell lymphoma (40%) [119].
To some extent, the location of lymphomatous involvement may also hinder its detection regardless of histopathologic subtype. The cutaneous location accounted for one-third of the 48 non-[18F]FDG-avid cases in Weiler-Sagie et al.’s study [119], including two with diffuse large B-cell lymphoma. The other most common locations of non-[18F]FDG-avid disease were bowel, bone marrow, effusions, and mucosal surfaces [119]. Clearly tumor burden has an effect and low tumor burden can be falsely negative.
Weiler-Sagie et al. did not address the degree of [18F]FDG uptake in the histologic subtypes of lymphoma, but this has been done previously [119]. Broadly speaking, low-grade lymphomas take up less [18F]FDG than high-grade lymphomas [120–122]. Standardized uptake values (SUV—a semiquantitative measure of tumor metabolism) of greater than 10 had a high likelihood of being aggressive and excluded low-grade lymphoma with a specificity of 81% in one study of patients with NHL [122]. Despite this, there is substantial overlap of [18F]FDG uptake between lymphoma grades with some low-grade tumors exhibiting high levels of [18F]FDG uptake (Fig. 7.1). The level of [18F]FDG uptake likely lies along a continuum based on the histologic grade of the lymphoma, but in an individual patient, the level of [18F]FDG uptake is probably not an adequate single surrogate marker for histologic grading of lymphoma.
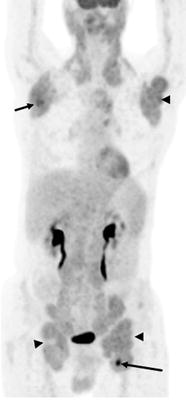
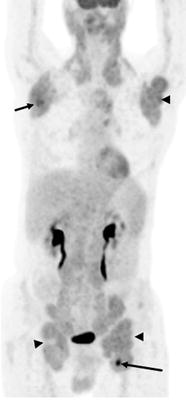
Fig. 7.1
A 65-year-old man with a history of chronic lymphocytic leukemia/small lymphocytic lymphoma (CLL/SLL). There is mild to moderate [18F]FDG uptake in bilateral cervical, axillary, para-aortic, iliac, and inguinal lymph nodes consistent with indolent NHL (arrowheads). Within the left inguinal adenopathy, there is a focus of more intense [18F]FDG uptake (arrow, SUVlbm max = 10.6). Biopsy of the more intense focus in the inguinal region revealed Richter’s transformation. Another area of more moderate uptake suspicious for early transformation can be seen in the right axilla (arrow)
One exception may be when a disproportionately high accumulation of [18F]FDG is seen in a single lesion compared to the others (Fig. 7.1). This may be indicative of transformation of a low-grade lymphoma to a high-grade lymphoma in the same patient, and [18F]FDG PET and PET/CT could help guide biopsies of these lesions [122]. Bruzzi et al. [123] retrospectively evaluated 37 patients with chronic lymphocytic leukemia (CLL), with and without signs and symptoms of transformation, with PET/CT. Using a SUVmax cutoff of 5, the negative predictive value of PET for detecting transformation was high (97%); however, the specificity and positive predictive value were low (80% and 53%, respectively) [123].
Bodet-Milin et al. [124] used PET/CT to prospectively guide biopsy in 38 patients with indolent NHL (n = 23 follicular, n = 10 CLL, n = 2 Waldenstrom macroglobulinemia, n = 3 marginal zone NHL) and clinical suspicion for transformation. Biopsy was obtained at the most accessible site with the highest SUVmax and 17 patients had histologic transformation on biopsy. The median SUVmax and gradient SUVmax (highest SUVmax − lowest SUVmax) were higher for those with histologic transformation. Using receiver operator curve analysis, a SUVmax threshold of 14 was optimal for identifying transformation (sensitivity/PPV ∼ 94%, specificity/NPV ∼ 95%) [124]. As expected, sensitivity or specificity could be improved one way or the other with higher and lower thresholds. Bodet-Milin’s study differed from Bruzzi’s in several regards—all patients had a clinical suspicion of transformation, and higher threshold values for transformation likely decreased the false-positive rate. They suggest that a higher threshold may be reasonable for patients with follicular versus chronic lymphocytic leukemias/small lymphocytic lymphoma (CLL/SLL), as CLL/SLL typically has less [18F]FDG uptake at baseline [124]. These data together suggest that PET may be useful for detecting transformation, but tissue confirmation is still required for confirmation at the present time.
[18F]FDG PET for Initial Staging of Lymphoma
It is well established that [18F]FDG PET is superior to 67Ga-citrate scintigraphy [114–118] and improves primary staging of lymphoma as compared to CT [125–129]. For nodal status, this is primarily due to the ability of [18F]FDG PET to detect lymphoma in normal sized lymph nodes that would be considered benign by CT size criteria. [18F]FDG PET also detects more extranodal lesions, for example, in the liver, spleen, bone, and lung, than CT does (Fig. 7.2), with less radiation exposure to the patient.
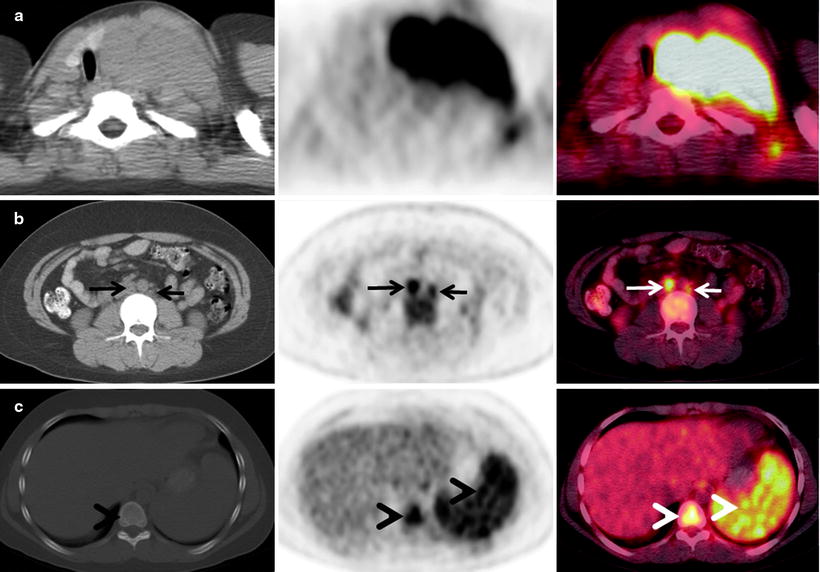
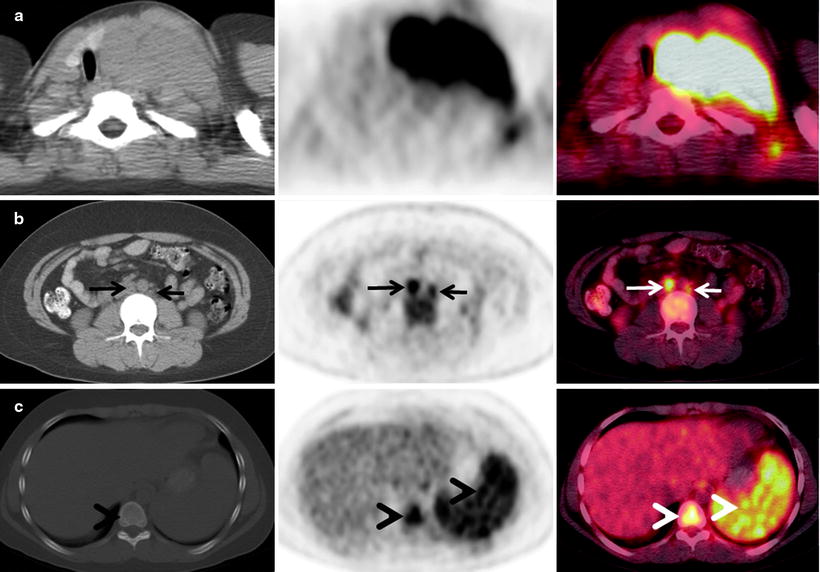
Fig. 7.2
A 34-year-old woman with Hodgkin’s lymphoma presented for staging with a PET/CT scan. The diagnostic CT scan with intravenous contrast was done prior to the PET scan and showed enlarged lymph nodes in the neck and upper mediastinum extending to but not below the aortic arch (not shown). This was consistent with stage 2 disease. The PET/CT scan shows that the mediastinal mass has intense [18F]FDG uptake (a). The PET scan also showed numerous [18F]FDG-avid lymph nodes below the diaphragm (b, arrows) and in the bone marrow and spleen (b and c, arrowheads). The patient was upstaged to stage 4 and received systemic chemotherapy (for a–c—left: CT; center: PET; right: fused PET/CT)
Biopsy is the gold standard to detect lymphomatous involvement in the bone marrow and is a routine component of staging procedures. Bone marrow biopsy and [18F]FDG PET have been reported to be approximately 80% concordant [127, 130, 131]. [18F]FDG PET is more likely to detect bone marrow disease when greater than 30% of the marrow is involved. False-negative [18F]FDG PET scans for bone marrow disease (PET negative, biopsy positive) occur if less bone marrow is involved, in lower grade histologies, or if the primary nodal disease is not [18F]FDG-avid. Misinterpretation of diffuse [18F]FDG uptake in the marrow due to concurrent systemic illness or recent administration of hematopoietic growth factors can potentially lead to false-positive [18F]FDG PET scans. This can be avoided by a carefully elicited clinical history. Sampling error on biopsy may also result in a “false-positive” PET study, which is actually true positive.
In one study, bone marrow involvement could be confirmed in 7 of 10 patients with negative iliac crest biopsies by [18F]FDG PET directed rebiopsy (N = 4) or anatomic imaging (N = 3) [131]. A meta-analysis investigating the use of [18F]FDG PET for detecting bone marrow disease revealed sensitivities in the 54–74% range and specificities in the 91–95% range [132]. Only a small number of studies evaluating Hodgkin’s lymphoma were included. When evaluating an [18F]FDG-avid focus in the bone, it is important to note that the concurrent CT scan may appear normal up to 50% of the time [133] and the context and entire distribution of uptake must be considered. [18F]FDG PET is complementary to, but cannot replace, bone marrow biopsy (Fig. 7.3).
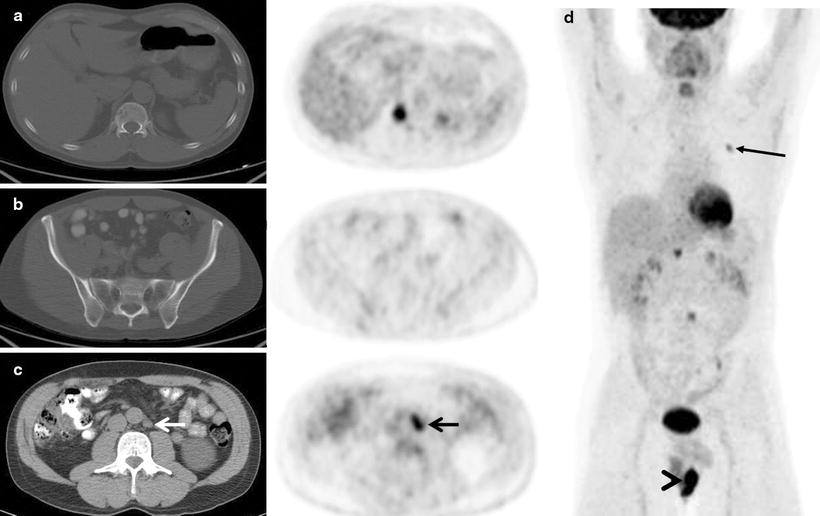
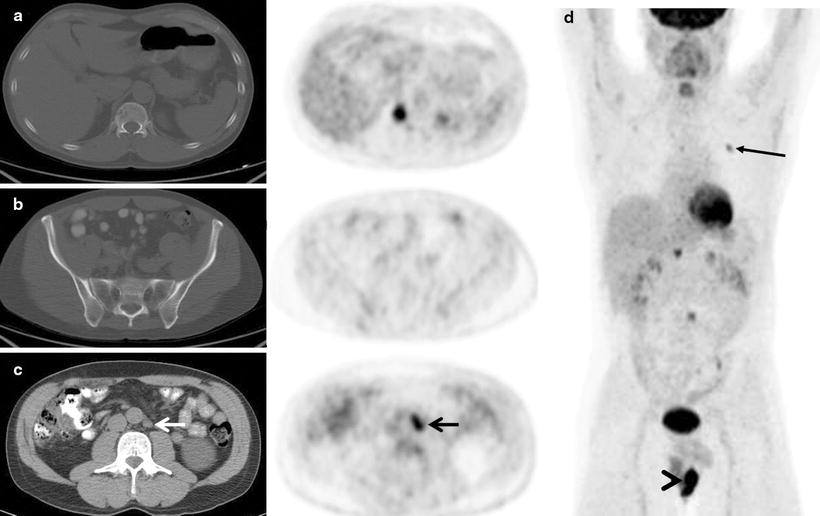
Fig. 7.3
A 55-year-old man with diffuse large B-cell lymphoma presented for a staging PET/CT scan. A focus of intense [18F]FDG uptake fusing to lytic changes in the right T11 vertebral body is seen (a). Biopsy of this lesion confirmed diffuse large B-cell lymphoma. Bone marrow biopsy was negative for lymphomatous involvement. Not surprisingly, the PET scan in the region of standard iliac crest biopsies showed normal marrow uptake. The PET and marrow biopsy were discordant in this case for lymphomatous involvement. Other sites of disease in the marrow were also found in the left rib (d, maximum intensity projection image, arrow). Additional extranodal disease was found in a scrotal mass (d, maximum intensity projection image, arrowhead), and the only nodal disease was a 1.5 × 1.2 cm left para-aortic lymph node with intense [18F]FDG uptake (c, arrows). Overall, the findings were consistent with stage 4 disease (a: top row; b: middle row; c: bottom row. For a–c—left: CT; right: PET)
The addition of [18F]FDG PET to lymphoma staging procedures correctly alters stage and leads to changes in management in 8–48% of cases [125, 127, 129, 134, 135]. Patients are upstaged more often and consequently receive more aggressive initial therapy; however, downstaging also occurs (Fig. 7.4). The inclusion of [18F]FDG PET in the primary staging of lymphoma has substantial impact on patient care. Although this has also been shown to be true for low-grade follicular NHL, the role of PET in the staging of other indolent NHLs (i.e., CLL/SLL) may be more limited [136–139].
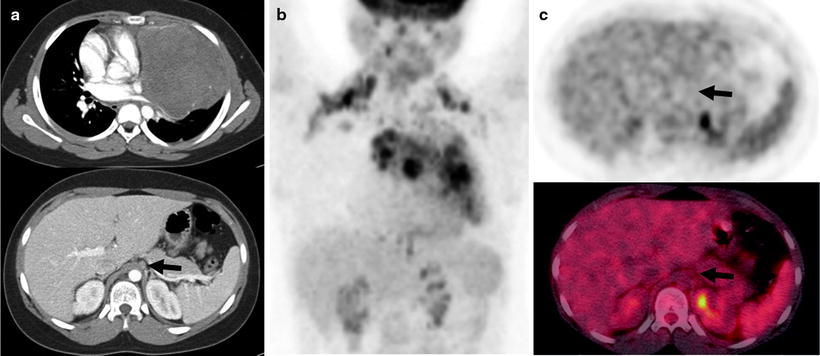
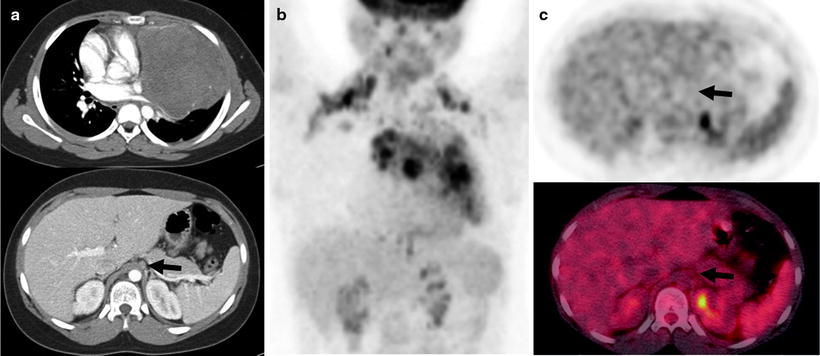
Fig. 7.4
A 26-year-old man with Hodgkin’s lymphoma underwent staging procedures. A diagnostic CT scan with intravenous contrast shows the large mediastinal mass (a—right top and bottom rows). There was a borderline abnormal size celiac axis lymph node suspicious for stage 3 disease (a—bottom row, arrow). The PET/CT scan shows abnormal uptake in the mass and nodes above the diaphragm (b), but no abnormal uptake in the celiac axis node below the diaphragm (c, left top and bottom rows, arrows). Physiologic renal excretion of [18F]FDG is also seen in the posterior and medial left upper quadrant. The PET downstaged the patient from stage 3 to stage 2, and he received a short course of chemotherapy and radiotherapy (a: left column—transaxial IV contrast CT scan slices; b: center column—coronal PET image; c: right column—transaxial PET (top) and fused PET/CT (bottom) images)
Despite the above support for the use of [18F]FDG PET in lymphoma staging, the early studies had several limitations. The studies often included small, heterogeneous patient populations, lacked gold standards at each disease site, and due to the nature of the disease, all [18F]FDG-positive (or negative) lesions could not be verified histologically. Furthermore, the definition of “PET positive” and interpretative criteria may not have been as well refined before experience was gained in image interpretation. For example, in at least 1.2% of patients referred for an oncology PET scan, a second, incidental primary tumor will be found [140].
[18F]FDG PET/CT Versus PET Alone
A majority of studies investigating the use of metabolic imaging in lymphoma have evaluated [18F]FDG PET alone. PET/CT improves lesion localization and provides more reliable tumor measurements. Tatsumi et al. [141] reported that combined PET/CT imaging may be better than either modality alone. In 53 patients with lymphoma, 48 of 1,537 findings were discrepant between PET and CT; 40 were correct PET findings, 5 were correct CT findings, and 3 remained uncertain. Nine patients were only staged correctly with PET/CT versus CT alone [141]. Allen-Auerbach et al. [142] found that PET/CT was more accurate than PET alone for the detection of lymphoma (94% versus 84%, respectively). PET/CT improves lesion localization, allows detection of lesions that might be missed by either modality alone, and provides more reliable tumor measurements.
Posttherapy Response Assessment by [18F]FDG PET and PET/CT
Residual masses on anatomic imaging in patients with lymphoma are common posttherapy but may not always indicate the presence of active malignancy [143]. A major advantage of PET is its ability to detect functionally viable tumor cells within the mass, distinguishing active lymphoma from fibrosis/necrosis. The information gained from posttherapy PET also provides prognostic information [129, 144–148]. Patients with PET-positive disease posttherapy (Fig. 7.5) have lower 1- and 2-year progression-free and overall survival rates (0–54%) than those who are PET negative (83–95%) (Fig. 7.6), regardless of the presence of a residual mass on CT. PET positivity posttherapy predicts subsequent relapse. Therefore, the results of a posttherapy PET scan may have important therapeutic implications.
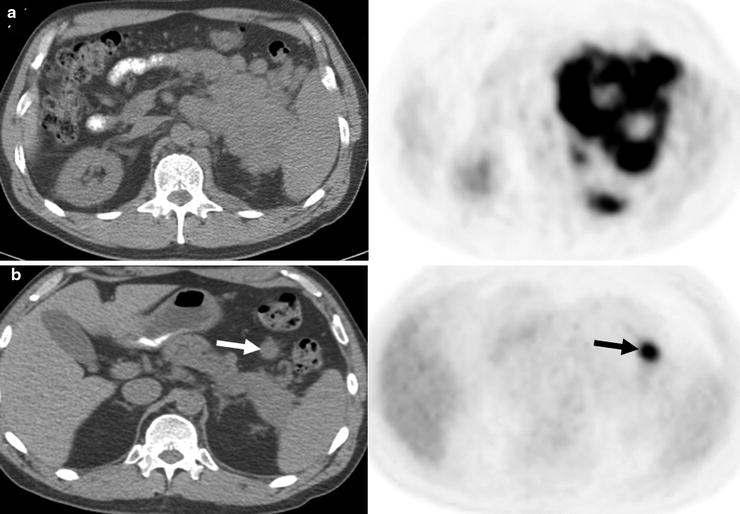
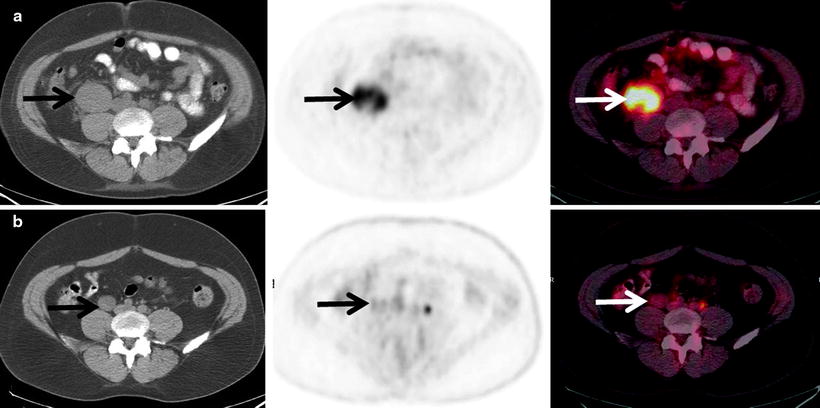
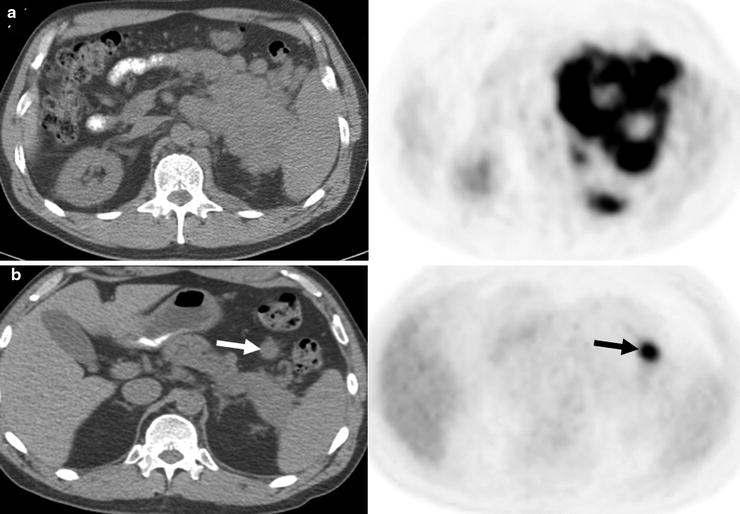
Fig. 7.5
Pre- and posttherapy PET scans in a patient with diffuse large B-cell lymphoma. The pretherapy PET scan shows intense [18F]FDG uptake in a left upper quadrant mass, adjacent nodules, and left paravertebral regions (a: top row). After 6 cycles of R-CHOP, a 2.3-cm nodule remained in the left upper quadrant with intense [18F]FDG uptake (b: bottom row, arrows) consistent with residual active lymphoma (positive posttherapy PET scan). A positive posttherapy PET scan is associated with a poor prognosis (for a and b—left: CT; right: PET)
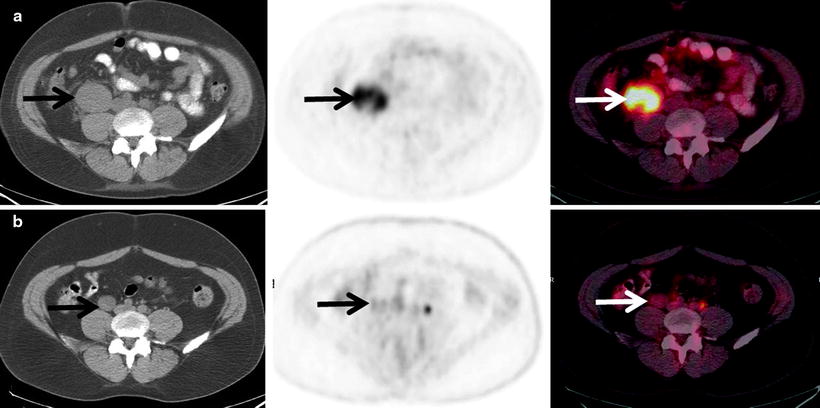
Fig. 7.6
Pre- and posttherapy PET scans in a patient with diffuse large B-cell lymphoma. The pretherapy PET scan shows intense [18F]FDG uptake in a right psoas mass (a: top row). After 6 cycles of R-CHOP, the PET scan was negative for active lymphoma with tumor uptake equal to background (b: bottom row). Although residual microscopic disease is not excluded, a negative posttherapy PET scan is associated with a good prognosis even if a residual mass remains on a CT scan. The residual [18F]FDG uptake may be due to posttherapy inflammation (for a and b—left: CT; center: PET; right: fused PET/CT)
Despite its improved prognostic implications, a negative posttherapy PET scan does not exclude the presence of residual microscopic disease that falls below the resolution of the PET scanner [129, 144–148]. The resolution of modern PET scanners falls between 0.5 and 1.0 cm [149] which converts to about 108–109 tumor cells [150]. Patients who are PET negative with a residual mass are more likely to recur than those who are PET negative without residual mass [129, 144–148].
The optimal time to obtain a posttherapy PET is a frequent question of referring oncologists, but the answer is not completely resolved. A minimum of 10 days postchemotherapy has been recommended to avoid false-negative scans due to the early treatment effect of “stunning” [151]. Longer and more variable times post-external beam radiation have been suggested [152], and optimal interval times post-RIT are beginning to be investigated and discussed in the section on RIT [153, 154].
Integration of [18F]FDG PET and PET/CT into Tumor Response Criteria
The major criteria for evaluating response to therapy in lymphoma, the International Workshop Criteria (IWC) [155], were revised in 2007 to include a posttherapy assessment by [18F]FDG PET [156]. Previously, the IWC were based on clinical and laboratory findings and changes in tumor size on anatomic imaging [155]. The revision was based on the numerous studies showing the predictive value of the posttherapy PET scan and a retrospective study in 54 patients with aggressive NHL [157].
In this study, PET scans were obtained 1–16 weeks after the completion of therapy (94% within 2–12 weeks) and were interpreted qualitatively as positive or negative. A positive scan was defined as focal or diffuse uptake in a location incompatible with normal anatomy [157]. A complete response required a negative PET scan, regardless of the size of a residual mass on CT and a negative bone marrow biopsy if it was positive before therapy. The integrated PET and IWC response criteria more accurately classified lymphoma response by identifying a subset of partial responders by IWC who had a complete metabolic response by [18F]FDG PET and a more favorable prognosis [157]. The criteria were subsequently found to be applicable to indolent NHL and HL [158, 159].
In addition to the response criteria, recommendations were also made about when to use PET in clinical trials in lymphoma. Pretreatment and posttreatment response assessments with PET were routinely recommended for typically [18F]FDG-avid tumors (DLBCL and HL), although the pretreatment scan was strongly recommended, but not mandatory. Our view is that a pretreatment PET scan is mandatory. Generally, for tumors that would have been considered by many to be incurable (FL and MCL) and tumors that are inconsistently [18F]FDG-avid, PET was only recommended if the response rate was a trial endpoint (for both) and if the baseline scan was positive since PET is more accurate than CT, a baseline and follow up PET/CT is often obtained in these lymphomas, as well.
A consensus report from the Imaging Subcommittee of the International Harmonization Project in Lymphoma on the acquisition and interpretation of posttherapy PET scans accompanied the revised IWC [160]. The interpretation criteria proposed are summarized in Table 7.3. Several additional notable recommendations were also made: (1) baseline scans are “strongly encouraged” to aid posttherapy scan interpretation, (2) IV contrast CT scans may complement the posttherapy PET scan depending on clinical and baseline information, but the size of lesions on CT should always be reported, (3) new lung lesions on a posttherapy scan must be interpreted with caution to avoid false positives due to inflammation or infection, and (4) specific recommendations on interpretation of bone marrow and residual extranodal disease are discussed. The incremental value of contrast CT appears modest, in some studies, over a non contrast CT. (e.g. Schafer).
Table 7.3
Scales for interpreting interim and posttherapy PET scans
Revised IWC [160] | Kasamon et al. [174] | ECOG criteria [190] | London criteria [189] | |
---|---|---|---|---|
Scale type | Visual | Visual | Visual | Visual |
Background comparator | Mediastinal blood pool | Mediastinal blood pool | Liver | Mediastinal blood pool Liver |
Negative | Lesions ≥ 2 cm Uptake ≤ mediastinal blood pool Lesions < 2 cm Uptake ≤ surrounding background | 0: No uptake 1: Minimal uptake (<background) 2: Equivocal (=background) | 1: No uptake 2: Uptake ≤ mediastinal blood pool 3: Uptake > mediastinal blood pool but ≤ liver | |
Positive | Lesions ≥ 2 cm Uptake > mediastinal blood pool Lesions < 2 cm Uptake > surround background | 3: Moderate uptake (>background) 4: Intense uptake (much > background) | Focal uptake > average Liver with anatomic correlate | 4: Moderately > liver 5: Markedly > liver |
Notes | Extranodal disease | 1. Only abnormal sites at baseline evaluated 2. Activity in spleen and marrow only positive if focal and clearly discernable 3. Symmetric foci in mediastinum and hilum only positive if rest of scan positive 4. New foci only positive if remainder of scan positive and anatomic correlate |
The evaluation of posttherapy PET for lymphoma is based on visual qualitative assessment of tumor uptake (Table 7.3), and this seems to differentiate progressers from nonprogressers well. Whether a semiquantitative-based analysis using SUV is needed in the posttherapy setting has not been studied in a large number of patients. An SUV-based analysis may be more useful, however, in the interim assessment setting and is discussed further below.
Multiple consensus reports using PET for response assessment (interim and posttherapy) in lymphoma and other tumors stress the importance of obtaining high-quality, standardized PET and CT scans on dedicated PET systems. Detailed discussions on obtaining such scans are found in reports by the National Cancer Institute [161] and the Netherlands multicenter trial group [162] and reviewed in the revised IWC [160] and PET Response Criteria in Solid Tumors (PERCIST) [163].
The use of [18F]FDG PET for routinely monitoring the response of low-grade follicular and other indolent lymphomas is debatable. Low-grade follicular and indolent lymphomas are currently considered incurable by many; therefore, primary endpoints for these lymphomas are usually PFS and overall survival (OS). These tumors also tend to have lower levels of [18F]FDG uptake compared to their aggressive counterparts. In the revised IWC criteria, [18F]FDG PET scans at baseline and posttherapy for follicular and indolent lymphomas are only recommended in clinical trials when overall or complete response rates are the primary study endpoints and only if the pretreatment scan is “positive” [156]. We believe that since [18F]FDG PET/CT is more accurate than CT, it is quite reasonable to perform [18F]FDG PET/CT in place of CT in such patients.
Interim PET and Risk-Adapted Therapy
Theory and Biologic Basis
Chemotherapy of tumors begins to affect tumor metabolism soon after it is started. In 1993, PET was used to sequentially assess breast cancer response to chemohormonotherapy, and decreases in the amount of [18F]FDG uptake in responding tumors were seen by 8 days into treatment [164]. The clear predictive value of PET in the posttherapy setting for patients with lymphoma has led investigators to evaluate its usefulness earlier during the course of chemotherapy, and numerous studies have upheld PET’s prognostic value in the interim therapy setting [165–171]. Event-free survival (EFS) is consistently lower in patients with positive interim PET scans (0–46%) compared to those with negative interim scans (62–98%), despite variability in the study designs (prospective versus retrospective, chemotherapy cycles, qualitative versus quantitative PET assessments), tumor histologies, and treatments employed. This body of work, including a landmark study by Gallamini et al. [22] (discussed below), is the premise for risk-adapted therapy for patients with lymphoma.
Importantly, Gallamini et al. [22] showed that in 190 patients with advanced HL, the results of early interim PET after 2 cycles of ABVD chemotherapy were more prognostic than the widely used and validated IPS. As previously discussed, the IPS for HL and IPI for NHL are both currently used to guide management decisions. Both indices are based solely on pretreatment parameters and do not consider the inherent “chemosensitivity” of the tumor or the rate of tumor kill which are both related to outcome, but PET can assess both of these factors [150].
The conceptual framework for PET providing an early readout on “chemosensitivity” is nicely discussed by Kasamon, Jones, and Wahl, and two underlying points are summarized [150, 172]. First, chemotherapy is expected to kill the same percentage of cells during each cycle (first-order kinetics) [173]. For example, 11 cycles of chemotherapy are needed for cure with 1010–1011 cancer cells. If each cycle kills one log of tumor cells, all cells are clonogenic, and there is no tumor regrowth between treatments. Second, due to the lower limit of resolution for current scanners, PET can only detect the initial logs of cell kill. A negative scan after therapy either means cure or residual microscopic disease. A true-negative interim therapy (or soon posttherapy) scan implies that the rate of kill is fast enough to achieve cure by the end of therapy, whereas a positive interim therapy scan does not [150, 172].
The logical question follows, “Can the prognostic information from an interim therapy PET scan be used to improve therapeutic strategies for patients based on their individual risk, hence risk-adapted therapy,” and this can be considered in two ways. First, should therapy be intensified to improve the chance of cure in a patient with a positive interim PET scan as cure is unlikely with the initial therapy? On the other hand, is it safe to omit or decrease therapy in a patient with a negative interim scan? As alluded to in the section above on treatment for early stage HL, this second option is particularly attractive to reduce the long-term toxicities from therapy in this otherwise curable population.
To date, there are a small number of published trials, but an even larger number of ongoing trials that are investigating these questions (Table 7.4). These trials are reviewed and limitations of the use of interim therapy PET for risk-adapted therapy discussed. Until the results of these trials are available and more mature, interim PET scanning and risk-adapted therapy should be performed in the clinical trial setting [156].
Table 7.4
Ongoing clinical trials investigating [18F]FDG-PET for risk-adapted therapy
University Hospital, Essen, Germany | PET Guided Therapy of Aggressive NHL (PETAL) [180] | Phase 3 | Aggressive NHL | CHOP or R-CHOP × 2 Positive PET, randomized to: CHOP or R-CHOP × 6, or Burkitt’s regimen × 6 |
ECOG 3404 | Response-adapted therapy for aggressive NHL based on early PET scanning [176] | Phase 2 | Advanced DLBCL | R-CHOP × 3, PET scan + R-CHOP × 1 Positive PET: R-ICE × 4 Negative PET: R-CHOP × 2 |
British Columbia Cancer Agency, Vancouver, Canada | Tailoring treatment for B-cell NHL based on PET scan results mid treatment [177] | Phase 2 | Advanced DLBCL | R-CHOP × 4 Positive PET: R-ICE × 4 Negative PET: R-CHOP × 2 |
Johns Hopkins University, Baltimore, MD | R-ICE and high-dose cyclophosphamide with PET/CT for DLBCL [178] | Phase 2 | Aggressive NHL | R-CHOP or equivalent × 3 Positive PET: R-ICE × 2, high-dose cyclophosphamide and rituximab Negative PET: R-CHOP or equivalent × 3 |
Hospices Civils de Lyon, Lyon, France | Randomized phase 2 study of 2 associations of rituximab and chemotherapy, with a PET-driven strategy* [179] | Phase 2 | DLBCL | Arm A: R-ACVBP × 4, PET after 2 and 4 cycles PET 2−, 4−: high-dose MTX, R-ICE PET 2+, 4−: high-dose MTX, Z-BEAM, ASCT PET 4+: salvage Arm B: R-CHOP × 4, PET after 2 and 4 cycles PET 2−, 4−: R-CHOP × 4 PET 2+,4−: high-dose MTX, Z-BEAM, ASCT PET 4+: salvage |
University of Cologne, Germany (GHSG), HD16 | HD16 for early stages: treatment optimization trial in the first-line treatment of early HL: treatment stratification by means of [18F]FDG PET [185] | Phase 3 | Early HL | Arm 1: ABVD × 2, PET scan, IFRT Arm 2: ABVD × 2 Positive PET: IFRT Negative PET: observation |
EORTC–GELA-IIL, H10 | Randomized intergroup trial on early [18F]FDG PET scan guided treatment adaptation versus standard combined modality treatment in patients with supradiaphragmatic early HL [186] | Phase 3 | Early HL | Standard Arm 1: favorable disease: ABVD × 3 Unfavorable disease: ABVD × 4 PET after 2 cycles of ABVD IFRT Experimental arm 2: ABVD × 2 Negative PET: standard treatment above, no RT Positive PET: escalated BEACOPP, IFRT |
UK NCRI Lymphoma Group | Randomized phase 3 trial to determine the role of [18F]FDG PET imaging in clinical stages 1/2A HL (RAPID) [188] | Phase 3 | Early HL | ABVD × 3 Response to therapy: PET Positive PET: ABVD × 1, IFRT Negative PET: randomized Arm 1: IFRT Arm 2: observation |
UK NCRI Lymphoma Group | Randomized phase 3 trial to assess response-adapted therapy using [18F]FDG PET imaging in patients with newly diagnosed advanced HL [189] | Phase 3 | Advanced HL | ABVD × 2 Negative PET: Arm 1: ABVD × 4 Arm 2: AVD × 4 Positive PET: Arm 1: BEACOPP × 4 Arm 2: escalated BEACOPP × 3 Not randomized regarding PET-adapted therapy |
Gruppo Italiano Terapie Innovative Nei Linfomi, Italy | Multicenter clinical study with early treatment intensification in patients with high-risk HL, identified as [18F]FDG PET scan positive after 2 cycles of ABVD [182] | Phase 2 | Advanced HL | ABVD × 2 Positive PET: escalated BEACOPP Negative PET: ABVD |
Intergruppo Italiano Linfomi Onlus | Early salvage with high-dose chemotherapy and ASCT in advanced HL with positive PET after 2 courses of ABVD and comparison with RT versus no RT in PET-negative patients [183] | Phase 3 | Advanced HL | Arm 1: ABVD × 2 Negative PET: ABVD × 4 Positive PET: high-dose salvage therapy PET—after ABVD × 6: no RT Arm 2: ABVD × 2 Negative PET: ABVD × 4 Positive PET: high-dose salvage therapy PET—after ABVD × 6: RT Not randomized regarding PET-adapted therapy |
University of Cologne, Germany (GHSG), HD18 | HD18 for advanced stages in HL [184] | Phase 3 | Advanced HL | Escalated BEACOPP × 2 Positive PET: BEACOPP × 6 (8 total) ± addition of rituximab Negative PET: BEACOPP × 2 (4 total) |
Clinical Trials of Risk-Adapted Therapy for Aggressive NHL
Kasamon et al. [174] published a phase II study in 59 patients with aggressive NHL, hypothesizing that suboptimal response on interim PET (PET positive) was sign of chemoresistance and that intensifying therapy in this group of patients would improve their outcome. After 2–3 cycles, R-CHOP chemotherapy was continued for a negative interim PET (tumor uptake ≤ mediastinal blood pool) or intensified to high-dose therapy and autologous stem cell transplant (ASCT) for a positive interim scan (tumor uptake > mediastinal blood pool). On an intent-to-treat basis and at median follow-up time of 34 months, 2-year EFS was 67% (95% CI 53–86%) in patients with positive interim PET versus 89% (95% CI 77–100%) for negative interim PET. The IPI was not associated with the interim PET result. The outcome of the PET-positive group compared very favorably to historical controls where <20% PFS was expected [174].
Moskowitz et al. [175] also performed a phase II study of risk-adapted therapy in advanced DLBCL, but with a slightly different strategy. The treatment scheme was dose-dense R-CHOP followed by PET after 4 cycles. PET-negative patients received ICE alone (ifosfamide, carboplatin, etoposide) for 3 more cycles. All PET-positive patients underwent biopsy, contrary to Kasamon’s study, and received 2 cycles of ICE. If the biopsy was negative, an additional cycle of ICE was given, but if positive, therapy was intensified to R-ICE, high-dose therapy, and ASCT. Interim PET scans were positive in 38 of 97 patients. Five of these 38 patients had a positive biopsy for tumor and proceeded to ASCT. The remaining biopsies showed inflammation. At a median follow-up of 44 months, EFS was not significantly different between interim PET-negative patients and PET-positive–biopsy-negative patients. Three of the five patients who underwent ASCT were alive and progression free at the time of publication.
Possible explanations offered for the high “false-positive” rate on PET were dose-dense initial therapy, increased inflammation due to greater use of rituximab than in prior studies, interim scan occurring relatively soon, a median of 12 days after last therapy (although longer than minimal recommendation of 10 days [160, 161]), and consolidation to ICE for all patients [175]. The authors concluded and recommended that biopsy be performed to confirm interim-positive PET scan findings prior to treatment intensification to ASCT [175]. It is also possible that false-negative biopsies occurred. It should be noted there are little data on the prognostic value of biopsy in this setting.
Treatment strategies of ongoing clinical trials are shown in Table 7.4. These trials primarily focus on intensification of therapy if the interim scan is positive and exploring findings of the previous risk-adapted trials. For example, the effects of ICE are being addressed in the ECOG trial 3404 [176] and a similar study in Vancouver [177]. A follow-up to Kasamon’s study is being performed at our institution with intensification of therapy to R-ICE and high-dose cyclophosphamide [178], and others continue to investigate intensification to ASCT [179].
The demonstration of true benefit of therapy intensification for those with a positive interim PET scan is limited by comparison to historical controls and the lack of randomization to standard versus experimental therapy in the above phase II trials. An ongoing study in Germany, Positron Emission Tomography Guided Therapy for Aggressive Non-Hodgkin’s Lymphomas (PETAL), attempts to address this [180]. In this study, patients with positive PET scans are randomized to either 6 additional cycles of R-CHOP or a more intense Burkitt’s lymphoma regimen. For those randomized to R-CHOP, 2 additional cycles are given (total 8 cycles) compared to those who have a negative interim PET scan (total 6 cycles). The results of these studies are awaited and should provide further insight into the usefulness of PET in the interim setting for aggressive NHL.
Clinical Trials of Risk-Adapted Therapy for Hodgkin’s Lymphoma
Risk-adapted therapy is also a major focus of research trials for patients with HL. For those with early, unfavorable, or advanced disease, the approach is generally similar to NHL, intensification of therapy based on a positive interim scan. For those with early stage disease, therapy deintensification after a negative interim PET is undergoing ongoing evaluation in attempt to reduce the risk of late term complications.
Dann et al. [181] performed a prospective study of risk-adapted BEACOPP therapy in 108 patients with intermediate- and high-risk HL. Treatment was adapted based on pretreatment risk factors (standard BEACOPP for intermediate-risk disease or escalated BEACOPP for high-risk (IPS ≥ 3) disease) and then escalated or de-escalated based on interim functional imaging with gallium-67 or PET after 2 cycles. With this schema, 5-year EFS and OS were not different between the intermediate- and high-risk groups at a median of 47 months of follow-up. In the high-risk group (n = 39), 31 (79%) had therapy de-escalated for negative interim scintigraphy, and the negative predictive value of interim PET was 93%. The authors suggested that it may be feasible to de-escalate therapy in a high-risk group based on negative interim PET. Only 4 of 19 patients (21%) with a positive interim PET scan had progressed at last follow-up (median 47 months). Several additional ongoing trials of risk-adapted therapy for advanced stage HL, mostly therapy intensification with positive interim PET [182–184], are listed in Table 7.4.
Three phase III studies investigating the omission of radiation therapy for early stage HL based on a negative interim PET scan are ongoing [185–187]. The studies are well designed in that patients are randomized and standard arms will be available for comparison. The preliminary reports from the EORTC–GELA group H10 and RAPID trial have demonstrated the feasibility, including patient willingness to undergo randomization and reasonable turnaround time of centralized PET review, of performing large-scale multicenter risk-adapted therapy trials based on PET [187–189]. The outcomes of both studies are awaited, as are the results of the GHSG H16 trial. It will undoubtedly be several years before these trials are completed and the data mature, but they should help to address the promising question of the use of PET for risk-adapted therapy in patients with NHL and HL.
Interpretation of Interim Therapy PET Scans
To date, most risk-adapted therapy trials have employed qualitative analyses for interpreting the interim PET scan as positive or negative. One of the major challenges and concerns has been that several visual scales have been used for interim PET interpretation. These include the International Harmonization Project in Lymphoma criteria by Juweid et al. [160] (which was developed and evaluated for the posttherapy setting), ECOG [190], and London criteria [189]. We have employed a 5-point visual scale for interpreting interim PET scans that is similar to the aforementioned criteria [174]. The criteria are compared in Table 7.3 and typically involve comparison of tumor to background tissue uptake. The consequence of qualitative assessments is a risk of interobserver variability in scan interpretation and difficulty in comparing results across clinical trials.
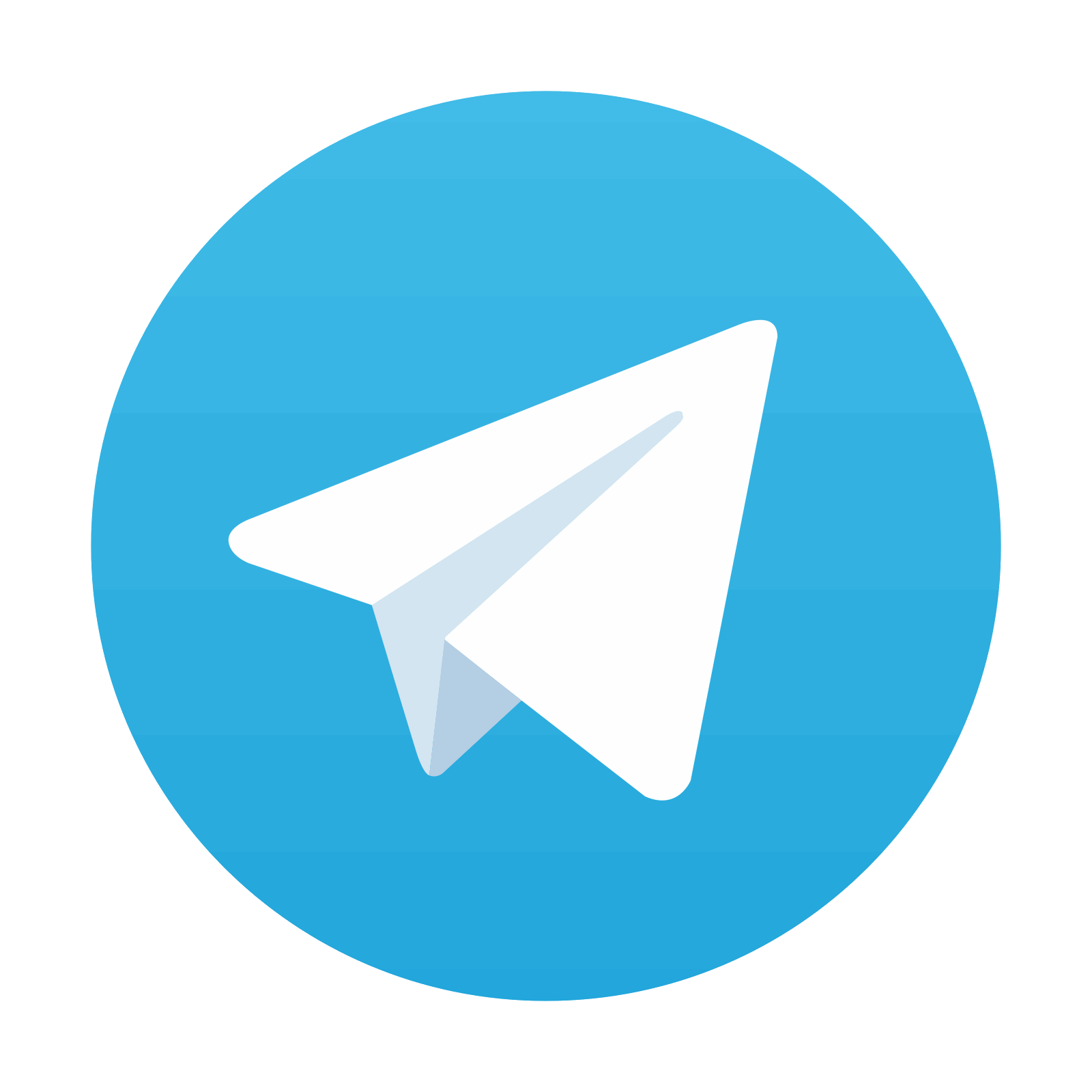
Stay updated, free articles. Join our Telegram channel

Full access? Get Clinical Tree
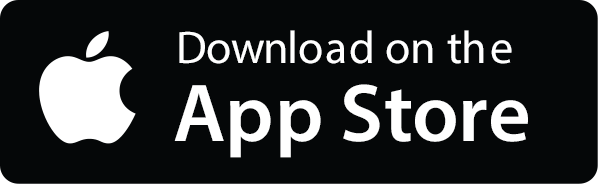
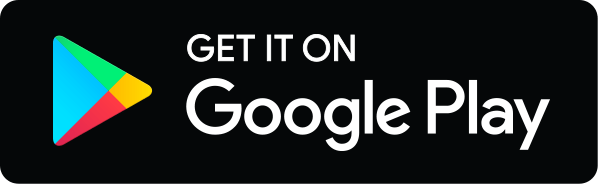