1. Confirmation of the presence of an intrauterine pregnancy
2. Evaluation of a suspected ectopic pregnancy
3. Defining the cause of vaginal bleeding
4. Evaluation of pelvic pain
5. Estimation of gestational (menstrual) age
6. Diagnosis or evaluation of multiple gestations
7. Confirmation of fetal cardiac activity
8. Imaging as an adjunct to chorionic villus sampling, embryo transfer
9. Localization and removal of an intrauterine device
10. Assessing for certain fetal anomalies, such as anencephaly, in high-risk patients
11. Evaluation of maternal pelvic masses and/or uterine abnormalities
12. Measuring the nuchal translucency (NT) when part of a screening program for fetal aneuploidy
13. Evaluation of a suspected hydatidiform mole
Recently, several studies encouraged the early diagnosis of major anomalies after demonstrating the association of increased fetal nuchal translucency (NT) and structural defects [2–4]. The development of high-frequency and high-resolution transvaginal ultrasound transducers, along with substantial improvement of the technology, has resulted in the visualization of fetal anatomic structures in greater detail earlier in gestation [5–16]. This helps to shift the prenatal diagnosis from the standard second-trimester anatomy scan into the first trimester, and also gives the opportunity for pregnancy termination in appropriate cases of anomalies identified earlier in gestation. On the other hand, the absence of major fetal structural malformations in the first trimester can reassure patients and reduce anxiety.
While there are some anomalies that will not be evident at a first-trimester anatomy evaluation, due to the natural history of fetal malformations, and a second-trimester anatomical survey remains the “gold standard,” we will consider some patients who might benefit from first-trimester anatomy ultrasound. Diagnostic performance of first-trimester ultrasound in detecting major fetal structural abnormalities has been described as 29–78.8 %, with an overall detection rate of 50 % [17–19]. The highest detection rate of 88–100 % has been reported in acrania, holoprosencephaly, hypoplastic left heart syndrome, omphalocele, megacystis, and hydrops [18]. Scanning in the first trimester may be performed either transabdominally or transvaginally. It was demonstrated that the transvaginal approach is significantly better in visualizing the cranium, spine, stomach, kidneys, bladder, and limbs. Complete fetal anatomy surveys were achieved in 64 % of transabdominal scans and 82 % of transvaginal scans at 13–14 weeks of gestation [20]. Using both transabdominal and transvaginal ultrasonography, noncardiac anatomy was seen in 75 % of fetuses with a crown-rump length of 45–54 mm and in 96 % with a crown-rump length (CRL) of more than 65 mm [21] (Figs. 3.1, 3.2, 3.3, and 3.4).
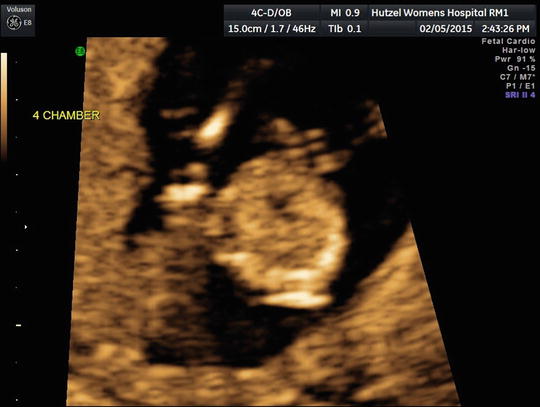
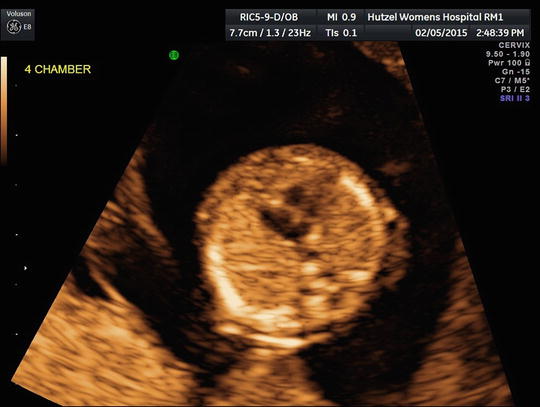
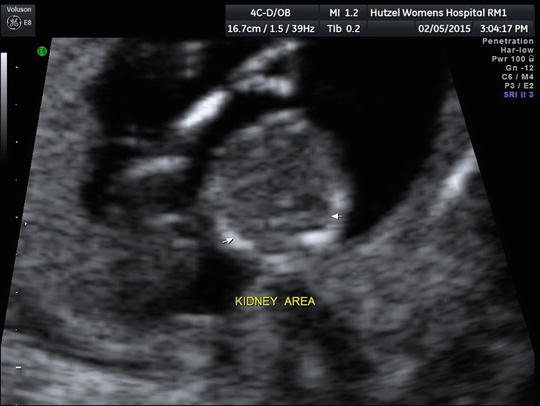
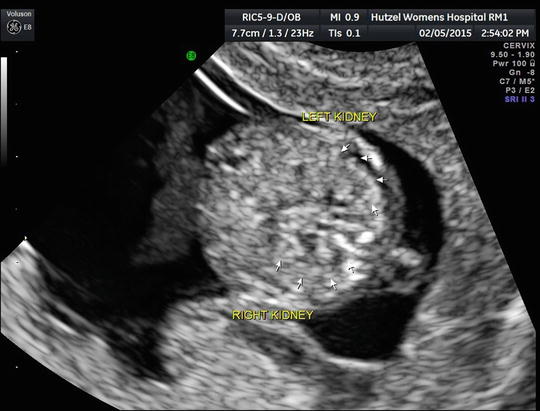
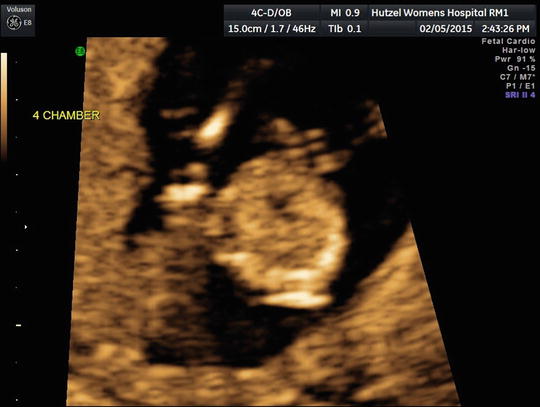
Fig. 3.1
A four-chamber view of the fetal heart at 13 weeks of gestation. Transabdominal approach
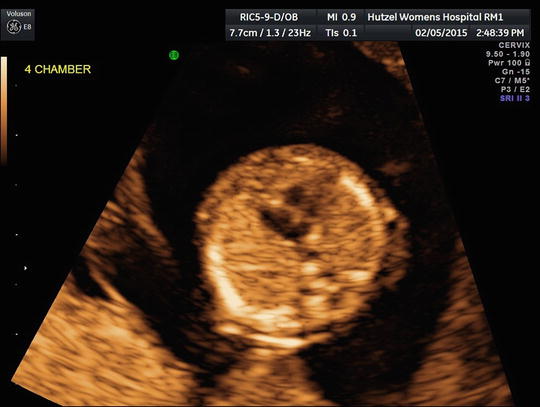
Fig. 3.2
A four-chamber view of the fetal heart at 13 weeks of gestation. Transvaginal approach
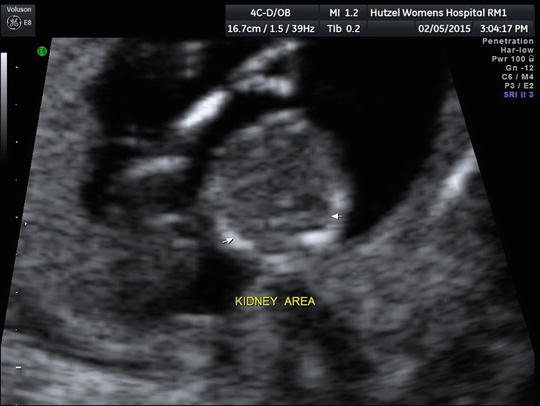
Fig. 3.3
Kidney area of the fetus at 13 weeks of gestation. Transabdominal approach
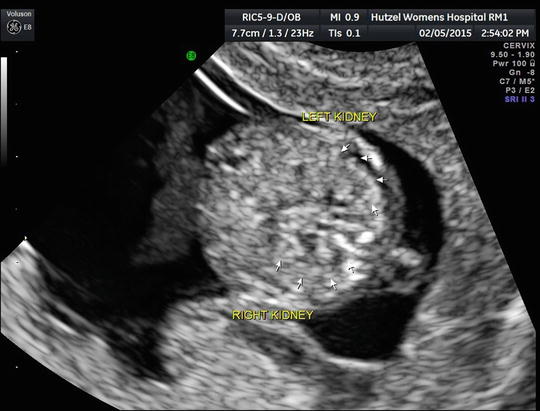
Fig. 3.4
Kidney area of the fetus at 13 weeks of gestation. Transvaginal approach
Maternal Comorbidities
Fetal anomalies may have various etiologies, such as genetic, environmental, or multifactorial. Various maternal conditions and/or their treatment are known to be associated with structural anomalies or restricted growth. Sonographic measurements of fetal ultrasound parameters are the basis for accurate determination of gestational age and detection of fetal growth abnormalities. Crown-rump length between 7 and 12 weeks is the most accurate parameter for first-trimester dating. First-trimester growth charts and predictive equations based on CRL instead of menstrual dating are more accurate [22]. Gestational age assessment is very important in the diagnosis of fetal conditions that involve early growth abnormalities due to conditions such as maternal hypertension, autoimmune disease, and preeclampsia. Clinical application of fetal biometry in abnormal growth is also important in cases of small- and large-for-gestational-age fetuses, chromosomal aberrations, and skeletal dysplasias.
Pregestational Diabetes
Maternal pregestational diabetes is a well-known risk factor for congenital anomalies. The types of congenital anomalies in diabetic pregnancies differ from those of nondiabetic pregnancies.
The overall incidence of congenital malformations in diabetic pregnancies has been reported to be 6–13 %, which is twofold to fourfold greater than that of the general population [23–25]. A higher proportion of central nervous system (CNS) abnormalities (anencephaly, encephalocele, meningomyelocele, spina bifida, and holoprosencephaly); cardiac anomalies (transposition of the great vessels, ventricular septal defect [VSD], single ventricle, and hypoplastic left ventricle); and kidney anomalies [26–31] are reported. The detection rate for CNS anomalies in the first trimester has been reported to be as high as 100 % in cases of anencephaly and encephalocele and only 18 % in cases of spina bifida [18, 32], because the typical findings of “lemon sign” and “banana sign” do not appear until the end of the first trimester [33, 34].
Obesity is a well-known risk factor for and comorbidity of diabetes. Moreover, several studies reported that women with pregestational diabetes and BMI higher than 28 kg/m2 have a threefold increase in the risk of congenital anomalies, and the risk further increases proportionally with BMI [35–37]. The potential role of first-trimester anatomy ultrasound in the obese gravida is discussed further below.
Rates of fetal malformation appear to be similar for type 1 and type 2 diabetes [38]. It is well known that the poorer the glycemic control is periconceptionally or early in pregnancy, the greater the risk is for congenital anomalies [39, 40]. Lack of proper glycemic control during pregnancy is associated with profound fetal anomalies. Maternal hyperglycemia at the time of fertilization (defined as a glycosylated hemoglobin [HbA1c] > 7.5 %) has been associated with a ninefold increase in congenital fetal anomalies and a fourfold increase in spontaneous abortion [41, 42].
Women with pregestational diabetes are advised to plan their pregnancy and optimize the glycemic control before pregnancy. The Canadian Diabetes Association (CDA) and American Diabetes Association (ADA) recommend HbA1c level for pregnancy to be ≤ 7 % before conception is attempted to decrease the risk of congenital malformations [43, 44]. They also encourage the women to take 4–5 mg of folic acid daily, although the evidence suggests that folic acid is more protective against spina bifida than anencephaly and encephalocele [45]. However, unplanned pregnancies occur in 50 % of all pregnancies and the majority of women do not seek prenatal care until after embryogenesis (4–8 weeks of gestation). Thus, we should consider the evaluation of anatomy using first-trimester ultrasound in a pregnancy complicated by pregestational diabetes. Although certain anomalies of the central nervous system may not be detected between 11 and 14 postmenstrual weeks, there have been case reports demonstrating the detection of congenital and major anomalies of the central nervous system using transvaginal ultrasonography in the first trimester [46].
While infants of diabetic mothers are at risk for a wide variety of malformations, one syndrome is strongly associated with diabetes. Caudal regression syndrome (Figs. 3.5 and 3.6) is a condition associated with hypoplastic lower extremities, caudal vertebrae, sacrum, neural tube, and urogenital organs [27, 30, 42]. Sirenomelia (the Mermaid syndrome) has been described as a severe and lethal form of caudal regression sequence and characterized by a single lower extremity, absent sacrum, urogenital anomalies, and imperforate anus. The prevalence of sirenomelia has been reported to be 1–3 per 100,000. These malformations occur before the ninth pregnancy week, which has important implications in the prevention of malformations in diabetic pregnancies. The detection of sirenomelia has been described as early as 9 weeks of gestation [47].
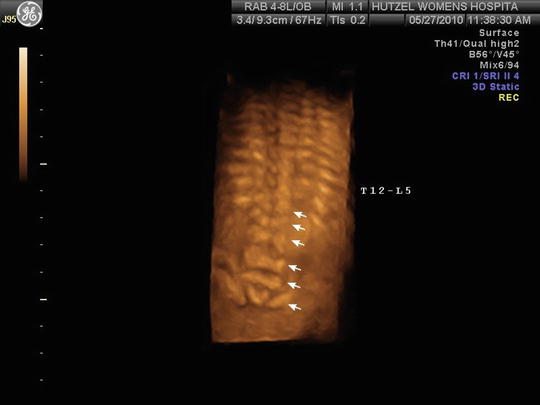
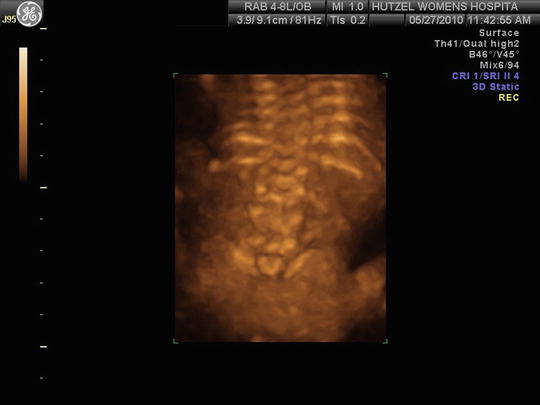
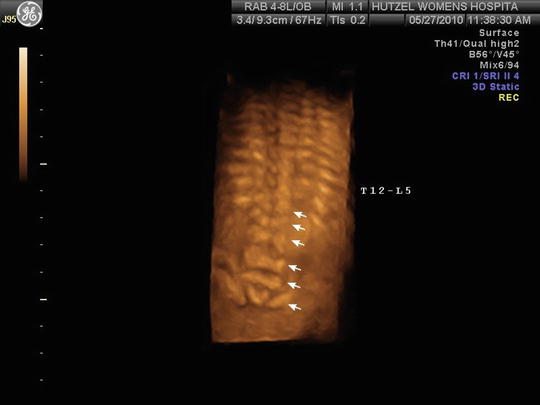
Fig. 3.5
Sacral agenesis in patient with pregestational diabetes
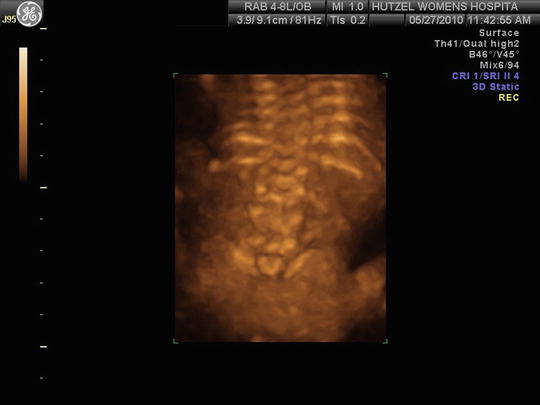
Fig. 3.6
Sacral agenesis in patient with pregestational diabetes
Obesity
Obesity in pregnancy, defined as maternal prepregnancy body mass index (BMI) of 30 kg/m2 and extreme obesity with BMI > 40 kg/m2, are now recognized as a major syndrome in the Western world [48–50]. In the USA, more than 35.8 % of women meet obesity criteria, and its prevalence is increasing steadily among women older than 20 years old, assuming epidemic proportions [51]. It is also associated with an increased risk of congenital anomalies such as cleft palate, neural tube defect and cardiac malformations, hydrocephaly, anal atresia, hypospadias, cystic kidney, pes equinovarus, omphalocele, and diaphragmatic hernia [52–55]. Thus, the ability to adequately visualize these structures at midtrimester prenatal ultrasound examination has significant clinical implications. Antenatal sonographic detection of congenital anomalies is difficult in obese patients. A patient’s body mass index significantly affects the ability of the sonographer to achieve a complete anatomical survey. As maternal BMI increases, the rate of completion of anatomic surveys decreases and the number of scans required increases [56]. The detection rate of anomalous fetuses with either standard or targeted ultrasonography decreased by at least 20 % in obese women compared to those with normal BMI [57].
Timor-Tritsch et al. proposed that ultrasound examination, with state-of-the-art equipment and in expert hands, can visualize as many structures at 13–14 weeks as it could at 16 weeks 5–10 years previously, and at 20–22 weeks 15–20 years previously [16]. Hendler et al. found that obesity increased the rate of sub-optimal ultrasound visualization for fetal cardiac and craniospinal structures and recognized that in these cases it may require visualization of these structures after 18–22 weeks using a transabdominal approach [58]. Gupta et al. recently suggested performing first-trimester fetal anatomic survey in addition to a routine second-trimester anatomy scan to improve the detection rate of congenital anomalies in obese patients [59]. Transvaginal sonography bypasses the maternal abdominal adipose tissue and the late first-trimester transvaginal scan may be the only opportunity to visualize the fetal anatomy adequately in the obese pregnant patient [60].
Maternal Conditions Associated with Congenital Heart Defects
Various teratogenic agents and maternal conditions have been implicated as the etiologic agents of congenital heart disease [CHD] (see also Chap. 4). Maternal pregestational diabetes has 2–5 times the risk of CHD. Anomalies, such as transposition of the great arteries, truncus arteriosus, visceral heterotaxy and single ventricle, are more common among offspring of diabetic mothers compared to women without diabetes [28, 61–64]. Ventricular septal defect and transposition of the great arteries are the most common cardiac defects in fetuses of diabetic mothers [61]. Establishing glycemic control before and early in pregnancy improves maternal and fetal outcomes, including reduction of CHD [65–67].
Overall, CHD is the most common congenital anomaly, with an incidence of 6–8 % of all live births, accounting for 30–45 % of all congenital defects [68–70]. Prenatal diagnosis of CHD may be used to optimize care and potentially be lifesaving [71–73]. Fetal echocardiogram at 18–20 weeks gestation is a well-established method for evaluation of fetal cardiac structure and function. With improved technology, it has become feasible to obtain images of the fetal heart as early as 11 weeks gestation [14, 74]. Moreover, there is mounting evidence that an increased NT is associated with major cardiac defects in the fetus and therefore represents an indication for specialized fetal echocardiography [75–77]. A recent meta-analysis showed that the use of the 99th centile (i.e., 3.5 mm) can identify around 30 % of fetuses with CHD, supporting the notion that NT is the strongest predictor of CHD in the first trimester [75]. Abnormal ductus venosus (DV) blood velocity waveform (absent or reverse A-wave) in the first trimester has also been associated with increased risk for adverse perinatal outcome, in particular for chromosomal anomalies and CHD [78, 79] (Figs. 3.3, 3.4, 3.5, 3.6, and 3.7). Abnormal DV blood velocity waveform in the first trimester is an independent predictor of CHD and should constitute an indication for early echocardiography. It has been reported that the use of DV blood velocity assessment increased early detection of CHD by 11 % with respect to the use of NT measurement alone [80]. The combined data from eight studies on euploid fetuses with increased NT (above the 95th centile) demonstrated abnormal DV blood velocity waveform in 87 % of fetuses with cardiac defects, compared with 19 % without cardiac defects [81]. Thus, many groups have suggested the use of DV as a secondary marker to be assessed selectively in fetuses with increased NT [82–84].
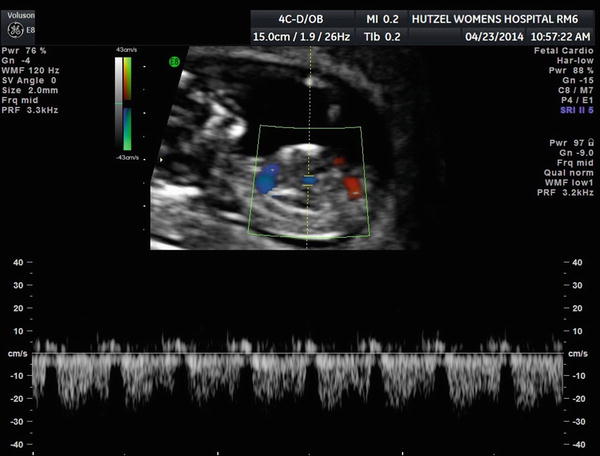
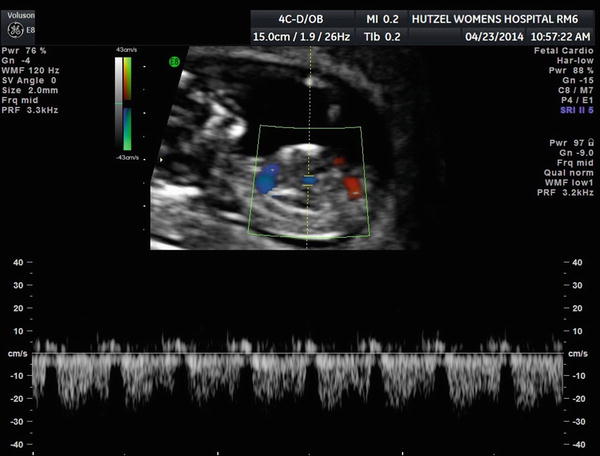
Fig. 3.7
Abnormal ductus venosus (DV) blood velocity waveform at 12 weeks of gestation
Several studies have shown that complete evaluation rate of the heart increased from 45 % at 11 weeks to 90 % between 12 and 14 weeks and 100 % at 15 weeks [12, 13, 85]. The visualization of the four-chamber view and the cross-over of the pulmonary artery and aorta have been reported from 44 % at 10 weeks to 100 % at 13–17 weeks [12]. Transvaginal echocardiography is reported to be superior to the transabdominal approach between 10 and 13 weeks of gestation, both methods are similar at 14 weeks of gestation, and transabdominal echocardiography is more accurate than transvaginal at 15 weeks of gestation [85]. Detection of cardiac anomalies in the first trimester varies by lesion, as noted in Tables 3.2 and 3.3.
1. Tricuspid atresia |
2. Pulmonary atresia (with or without ventricular septal defect [VSD]) |
3. Mitral atresia |
4. Aortic atresia |
5. Hypoplastic left heart syndrome (aortic and mitral atresia or severe stenosis) |
6. Complete transposition |
7. Corrected transposition |
8. Double inlet ventricle |
9. Atrioventricular septal defect (large septal defects) |
10. Truncus arteriosus |
11. Tetralogy of Fallot |
12. Large ventricular septal defects |
13. Complex lesions in the setting of laterality defects |
Developmental lesions |
1. Mild aortic/pulmonary stenosis |
2. Mild mitral/tricuspid valve abnormalities |
3. Coarctation of the aorta |
4. Cardiac tumors |
5. Cardiomyopathies |
Septal defects |
1. Ventricular septal defects |
2. Primum atrial septal defects |
3. Atrioventricular septal defects |
Others |
1. Tetralogy of Fallot with normal size pulmonary arteries |
2. Abnormalities of pulmonary venous return |
Phenylketonuria (PKU) is another metabolic disorder that is associated with CHD. Women with PKU who have elevated phenylalanine levels are at increased risk for offspring with CHD. VSD and coarctation of the aorta are most common in this population [86]. Levels exceeding 15 mg/ml are associated with a 10- to 15-fold increase in CHD [87]. The etiology of CHD is related not only to elevated blood phenylalanine levels, but also to poor protein and vitamin intake during the first trimester [88]. Diet control before conception and during pregnancy has shown reduced risk of CHD [89, 90].
Anticonvulsants, a class of drugs that includes phenytoin, carbamazepine and sodium valproate, are commonly used in the treatment of epilepsy. The incidence of congenital defects is 4–10 %, an approximate twofold to fourfold increase compared to the general population [91–97]. Polytherapy with antiepileptic drugs (AEDs) is associated with a higher malformation rate than monotherapy [98]. Use of certain AEDs during pregnancy increases the risk for specific congenital malformations, such as neural tube defects, cleft lip and palate, and cardiovascular malformations [99–102]. Valproic acid monotherapy, among the different regimens, has the highest risk of congenital abnormalities in offspring [94]. The use of valproate and carbamazepine is strongly associated with NTDs, especially with spina bifida. The prevalence of spina bifida is approximately 1–2 % with valproate exposure and 0.5 % with carbamazepine [103].
Carbamazepine exposure is associated with Tetralogy of Fallot, esophageal atresia, vertebral anomalies, and multiple terminal transverse limb defects [97]. The most common cardiac anomalies reported among offspring exposed to carbamazepine are VSD, Tetralogy of Fallot, patent ductus arteriosus (PDA) and atrial septal defect (ASD) [97, 100, 104, 105]. In light of these results, we should consider first-trimester anatomy ultrasound and fetal echocardiogram for women with epilepsy on AEDs.
Alcohol abuse during pregnancy is associated with health problems to both mother and fetus.
Of the four million pregnancies in the USA each year, 3–5 % of women drink heavily throughout pregnancy [106]. The fetal alcohol syndrome (FAS) is considered to be the most severe manifestation of the adverse effects of alcohol on the fetus. A diagnosis of FAS requires prenatal alcohol exposure and the following characteristics: fetal growth restriction, neurocognitive delays and/or mental retardation, and at least two facial dysmorphic features (short palpebral fissures, thin vermillion border, or smooth philtrum) [107]. FAS occurs in 4–10 % of children born to alcoholic mothers. CHD is reported in 25–50 % of infants with FAS; ASD and VSD are the most common [108–110]. The findings suggest that prenatal alcohol exposure as a potential etiology of CHD may also be considered as an indication for performing first-trimester fetal echocardiogram.
Maternal Vascular Disease
Women with hypertension, renal disease, and vascular disease have a recognized increased risk of preeclampsia, fetal growth restriction, and other adverse pregnancy outcomes. While most of these women will be candidates for low-dose aspirin therapy, identification of a particularly high-risk subset may allow more intensive surveillance and targeted interventions. Abnormal placental vascular development is a basis of common obstetrical disorders such as fetal growth restriction and preeclampsia. Uterine artery Doppler has been investigated as a predictive and diagnostic tool.
It has been reported that pregnancies with an increased risk of developing hypertensive disorders and related complications have an abnormally increased UtA-PI in early pregnancy [111]. The 11- to 14-week period is characterized by an elevated UtA-PI and bilateral notching. As pregnancy progresses, UtA-PI decreases and bilateral notching is less prevalent [112, 113]. A meta-analysis involving 55,974 women has shown that first-trimester uterine artery Doppler is a useful tool for predicting early-onset preeclampsia, as well as other adverse pregnancy outcomes [114]. Aspirin treatment initiated before 16 weeks of pregnancy may reduce the incidence of preeclampsia and its consequences in women with ultrasonographic evidence of abnormal placentation diagnosed by first-trimester uterine artery Doppler studies [115].
Summary
First-trimester ultrasound is already a common part of our obstetric armamentarium. As our patients, their comorbidities, and the sophistication of ultrasound change, so too may we change our approach to prenatal diagnosis. While the information presented here does not reflect current standard of care, we anticipate further evolution of condition- and exposure-based recommendations, including first-trimester anatomy studies and echocardiography in selected populations.
Teaching Points
Ultrasound performed in the first trimester confirms an intrauterine pregnancy, establishes accurate dates, pregnancy failure, and ectopic pregnancy.Stay updated, free articles. Join our Telegram channel
Full access? Get Clinical Tree