Development phase
Desired outcome
Basic research
Target identification
Discovery/candidate optimization/prototype development
Molecule or prototype that has suitable properties to interact with the pharmacological target in vivo
Preclinical development
Demonstrate proof of principle in animal models
Early clinical development
Pharmacokinetics, pharmacodynamics, proof of concept, dose selection
Late clinical development
Demonstration that the product is efficacious and safe
The objective in preclinical research is to demonstrate that the investigational product performs as desired. For a cholesterol ester transfer protein (CETP) inhibitor, this would mean that it increased HDL and lowered LDL. For a rheumatoid arthritis treatment, it may mean improvement of inflammatory biomarkers or improvement at the level of the joint. The criteria used to satisfy proof of principle and the animal model(s) selected can have a significant influence on subsequent steps. Whenever possible, it is recommended that the criteria used for proof of principle in animal models be similar to the criteria that will be used in phase II trials in humans. Once proof of principle has been demonstrated, distribution and metabolism of the pharmaceutical product is known, and appropriate toxicology experiments have been conducted, an investigational new drug application to test the drug in humans can be considered. The investigational new drug application is a major milestone in the drug development process which requires careful documentation of years of preclinical work. Guidance documents from health authorities such as FDA and EMA can be found on their websites and are helpful in the preparation of regulatory submissions.
The phase I or first in human studies are usually conducted in specialized facilities where the study subjects are closely monitored. Initially a single dose is administered to a single subject. Once a specified number of study subjects have completed a single dose and no clinically significant side effects are observed, the dose of the study drug can be increased. These studies are referred to as single ascending dose studies. The objective is to determine the dose range where efficacy is observed and side effects are minimal and within an acceptable rate. Next multiple dose studies are performed. In these studies, subjects receive multiple doses of the study medication. These are usually one daily dose for oral medications (dependent of the half-life) in order to better delineate the therapeutic dose range. For monoclonal antibodies, the rate of administration may be less frequent such as twice weekly, weekly, or monthly. Pharmacokinetic and pharmacodynamic assessments are performed as part of most phase I studies. The objective at the end of phase I is to have sufficient information regarding the dose range that will be required to demonstrate proof of concept in humans.
During phase II several dosing regimens will be assessed using a placebo-controlled or an active comparator experimental design. The objectives are to prove that the pharmaceutical product achieves the desired clinical effect (proof of concept in humans) and to determine the optimal dose or doses to be carried forward to larger phase III trials. In the design of the phase II trial, at least one dose should be higher than the anticipated optimal dose and at least one dose should be lower than the minimally effective dose such that the optimal dose or doses become apparent as a result of the study. In reality, this is seldom the case. Due to the limited number of subjects in these studies, surrogate endpoints are heavily relied upon to determine dose selection. Well-conducted imaging studies can add significant value during early clinical development [5]. This is because imaging studies can often accurately measure changes in pathophysiological processes, thereby providing valuable information for either efficacy or safety.
Phase III clinical trials require substantial strategic, technical, operational, and financial resources. The objective is to demonstrate that the new product in its studied route and frequency of administration provides a clinically meaningful benefit compared to the risks involved for the study population that has been investigated. The concept of benefit to risk ratio is paramount. Historically sponsors focused on demonstrating benefit while collecting adverse events in a routine fashion during phase III trials. Today, that strategy is unlikely to be successful in many therapeutic areas. Safety must be actively assessed by identifying potential safety risks and designing studies to evaluate the risk relative to placebo or active comparators. A recent example is the serotonin 2b antagonist lorcaserin for weight loss where echocardiography was performed to assess cardiac valvular function [6].
In retrospect, identification of a clinical target appears simple. We will use the example of hypercholesterolemia to demonstrate this concept. Basic research identified the key physiological steps in the pathway for cholesterol synthesis. This revealed several potential steps in which the synthesis of cholesterol could be inhibited to lower serum total and LDL cholesterol. Pharmaceutical developers targeted the HMG-CoA reductase enzyme and the products known as statins emerged.
A more recent target that was selected for the treatment of hypercholesterolemia is the cholesterol ester transfer protein. CETP transfers cholesterol from HDL cholesterol to very low-density or to low-density lipoproteins (VLDL or LDL). Inhibition of this process results in higher HDL levels and reduces LDL levels. Torcetrapib was the first molecule of CETP inhibitors that demonstrated a dose-dependent increase in HDL and a decrease in LDL with and without an added statin [7]. In the phase III trial, there was a 58 % increase in deaths among patients taking torcetrapib and atorvastatin versus those taking atorvastatin alone [8]. Some scientists believe that the increased mortality observed with torcetrapib was secondary to unintended increases in blood pressure [9]. These scientists and their organizations have continued to develop their CETP inhibitors by evaluating their prospective compounds for changes in blood pressure. Due to current limitations in the understanding of lipid physiology, there is uncertainty as to whether CETP will be a viable target for pharmaceutical intervention.
At the conclusion of a phase III program, there is a large amount of data that is available pertaining to the pharmaceutical product. Analysis of this data can be valuable in determining the potential for use of this drug in additional indications. Bevacizumab (Avastin®, Genentech, San Francisco, CA, USA) which is a monoclonal antibody to vascular endothelial growth factor will be used as an example of closely related additional indications. In February 2004 the FDA approved Avastin for use in combination with intravenous 5-FU-based chemotherapy as a treatment for first-line metastatic colorectal cancer. In June 2006, the FDA approved Avastin in combination with intravenous 5-FU-based chemotherapy for patients with metastatic colorectal cancer who have been previously treated for their cancer (or second-line metastatic colorectal cancer). Investigation of additional tumor types followed such that in October 2006, the FDA approved Avastin in combination with carboplatin and paclitaxel for the first-line treatment of patients with unresectable, locally advanced, recurrent, or metastatic non-squamous, non-small cell lung cancer. Subsequently approval for glioblastoma and metastatic renal cell carcinoma followed.
At times, the emerging data may indicate potential application for a very different patient population. Zoledronic acid will be discussed as a representative example of this situation.
Zoledronic acid is a bisphosphonate drug that works by inhibiting osteoclast-mediated bone resorption. It was first approved by the FDA in 2001 for the treatment of hypercalcemia of malignancy at a dose of 4 mg per infusion with retreatment permitted after 7 days. In 2002 zoledronic acid was approved for patients with multiple myeloma and patients with documented bone metastases from solid tumors at a dose of 4 mg per infusion every 3–4 weeks. During its development for oncology uses, it became apparent that zoledronic acid would also be useful in patients with metabolic bone disease. A development program for metabolic bone diseases was initiated. In 2007 it was approved first as a single 5 mg infusion for the treatment of Paget’s disease of bone. Studies were performed to support additional indications within metabolic bone disease. It was approved as a 5 mg once-yearly intravenous treatment for osteoporosis in postmenopausal women. In 2008, zoledronic acid at a dose of 5 mg annually was approved for the prevention of fractures following a hip fracture and for the treatment of osteoporosis in men. In 2009, it was approved for the treatment and prevention of glucocorticoid-induced osteoporosis in patients expected to be on glucocorticoids for at least 12 months and for the prevention of osteoporosis as a single 5 mg dose that is effective for 2 years.
Marketing authorization is based on all the discovery, preclinical, and clinical studies performed to date in clinical trials through phase III. Phase IV studies are designed to provide additional data that are of value to patients and healthcare practitioners that were not collected during the phase III studies. These must be conducted within the current prescribing instructions. They may investigate specific populations, compare dosing regimens, monitor a safety parameter, or investigate a new efficacy endpoint. It is common for health authorities to make marketing authorization contingent upon the conduct of additional post-marketing studies to assess potential safety concerns. In conjunction with use of the pharmaceutical product outside of the clinical trial setting, it is also common for safety issues to arise. These safety issues will need to be evaluated using the clinical trial data as well as various epidemiological sources. Imaging studies within the phase IV environment are relatively common and add value by objectively measuring the impact of the pharmacological intervention on either efficacy or safety parameters.
Imaging as a Biomarker
Advances in imaging technology have enabled scientists to detect events at the cellular level. Hardware and software manufacturers have increased the resolution of their products such that detection sensitivity and resolution have improved markedly. It is clear that imaging technologies have revolutionized the practice of medicine over the past few decades. This has contributed to improved diagnostics as well as improved monitoring of response to therapy. The result is a quantifiable improvement in the quality of care in most therapeutic areas resulting in improvements in both quality and duration of life. The percentage of the population living into their 70s, 80s, and beyond is among the most rapidly expanding segments of the population in many countries.
Many individuals who work in drug development view imaging endpoints as a biomarker analogous to C-reactive protein (CRP) for inflammation. A biomarker is a characteristic that is objectively measured and evaluated as an indicator of normal or pathological biological processes [10]. Biomarker programs within clinical development organizations typically assess serum markers, DNA, and RNA for association with the disease process. In an autoimmune inflammatory disease such as rheumatoid arthritis, CRP may be used to assess disease activity. Changes in CRP can be used to select doses of a therapeutic agent used to treat rheumatoid arthritis.
In contrast, imaging is the measurement of an in vivo biological process. For rheumatoid arthritis this would involve imaging of specific joints. While CRP is a systemic biomarker, imaging can provide additional information by demonstrating changes at specific anatomical locations (e.g., joints impacted by arthritis). Imaging is performed on the organ of scientific interest such as the heart, bone, or joint, while many other biomarkers are derived from the serum or circulating cells. During the development of a biomarker strategy, several biomarkers can be used together to derive a model predictive of a particular disease. Imaging may be considered as the net effect of both local and circulating factors on the disease process. As such, it is highly clinically relevant for many diseases.
A key property of imaging modalities is that they can provide critical data regarding disease progression. It is important to be able to detect progression of disease when patients are asymptomatic because often a disease process becomes less amenable to medical treatment as it becomes more advanced. From the perspective of drug development, demonstration of an improvement in patient signs and symptoms, while clinically relevant and necessary, is often not sufficient to gain regulatory approval. In diseases such as arthritis where pain is a key symptom, agents that reduce pain can be approved on this basis. From a payer perspective these agents will be considered alongside aspirin, acetaminophen, and NSAIDs. Pharmacological therapies which modify the disease process are much more highly valued. In order to gain approval for a product that modifies the disease, it is also necessary to show either an improvement or a reduction in the rate of disease progression which often requires the use of imaging.
The potential that imaging technologies have within clinical development has barely scratched the surface. While there are many reasons for this gap between potential and realized benefit, it is important to focus on the key elements required for success. The consensus opinion among imaging researchers and healthcare regulators is that the potential for the use of imaging in clinical trials for drug development hinges on appropriate use of standard principles. With appropriately rigorous trial conduct, the clinical trials will be more robust, require fewer subjects, and will be more likely to provide conclusive results that will allow for effective decision making. Simply incorporating imaging endpoints into a clinical trial does not guarantee that useful data will be generated. Rather it is the how the imaging component is designed and implemented that is critical to the generation of high-quality data that will facilitate interpretation of the trial results. As will be discussed next, there is a trend to collect key data to determine whether the drug candidate will be likely to garner regulatory approval and, if so, whether it will be competitive in the marketplace earlier in the development process than may have occurred in the past.
Key Elements for Product Approval
Advancing a product from the laboratory into the clinic or from early to late development while necessary for product approval will turn out to represent a failed investment if the product does not get approved. Therefore, it is important for all individuals working in drug development to have a sound understanding of the elements that are required for marketing authorization of pharmaceutical products globally. Whether one is working to register a new chemical entity, a new biologic agent, or a new medical device, the same principles apply. Demonstration that a product is effective followed by a listing of the adverse events experienced during the clinical trials will rarely be sufficient for approval with a few exceptions. These exceptions include diseases with high unmet need where no treatments are available.
The key measure for health authorities in 2010 is the benefit to risk ratio (BRR) for the specific indication and specific population. For example, one may get approval for a product for hypertension, colon cancer, or rheumatoid arthritis. It may be approved for first-line therapy, or it may be approved for use after first- or second-line therapy. How the BRR of the product compares to other available alternatives will determine whether approval is for first-line, second-line, or salvage therapy. It is also a function of whether or not the sponsor has developed the product in a manner that highlights its benefits in specific populations, or relative to other products. These can be defined by means of demographic, disease, or genetic characteristics.
Approval truly boils down to whether the sponsor can genuinely demonstrate and communicate a sound understanding of how their product works. This requires building upon the foundation of the mechanism of action (MOA) to select a patient population that should benefit based on the MOA. The next step is to validate the working hypothesis by demonstrating efficacy in the target population. Finally, one must show a comparable or better overall BRR compared to other available treatments for the specific population to be used as first-line therapy.
These changes in regulatory attitude are part of the change in medicine towards individualization of therapy. While this is being led in oncology through the increased use of tumor antigens to determine prognosis and treatment, it is occurring in many other disease areas through the use of patient characteristics including laboratory and imaging variables [11]. Defining the patient population(s) that will benefit is a key part of any clinical development program.
How have these changes in health authority decision making impacted clinical development programs? These changes have resulted in the selection of molecules with very specific actions that maximize their effect on the desired pharmacological target, while minimizing unintended off target effects. Monoclonal antibodies have experienced a marked increase and represent an increasing percentage of new products reaching the market [12].
Earlier Decision Making
Unfortunately, pharmaceutical product development will have more products that do not make it to market relative to those that succeed. A product that does not make it to market generates no revenues. Within organizations there is often a desire by team members to continue projects despite extremely low probabilities of ever recovering the associated expenses. As the competitive landscape intensifies, successful organizations will be those who are able to generate scientifically robust and clinically relevant data early in the development process and those who make evidence-based decisions on therapeutic agents within the development portfolio [13].
During phase I studies individuals with the targeted disease are being studied earlier and more extensively than they were historically. This is because while healthy volunteers can be used to determine the basic pharmacokinetics, effects on vital signs, and routine laboratory parameters, they are not informative in providing data relative to the pharmacodynamics of the disease process. Phase I studies provide the opportunity to explore the effects of the drug on the pathophysiology of the disease. Proof of principle is often established in phase I. A good understanding of the impact of the drug on the various components of the disease guides the doses to be taken forward into phase II.
Phase II studies will prove that the scientific concepts leading to clinical improvement in the target disease have been met. They will hone in on the population(s) that will derive the greatest BRR, as well as the dosing regimen to be used in phase III. Increasingly, active comparators are being included in phase II studies so that sponsors can determine where (e.g., first versus second line) in clinical practice their therapy will be used.
Inevitably, at the end of phase II, there remain unanswered questions regarding the product and its potential to modify the targeted disease. Could these data have been collected earlier in the development process? In many cases, the answer is yes. Phase III studies are becoming increasingly larger and often represent major investments even for the large multinational companies. While it is relatively easy to make a good decision when the information quality is excellent, as the ambiguity of the data increases, the probability of making an investment error in the hundreds of millions of dollars for the initiation of a large phase III program increases. The take home message is to invest in understanding the MOA of the product and the impact of the drug on the pathophysiology of the targeted disease population by the end of phase II such that informed investment decisions can be made. Companies that do not do this are unlikely to remain viable in their current form.
Opportunities for Imaging
From a regulatory perspective, health authorities are looking for a logical sequence of events that give them a high probability that the product will perform as suggested by the sponsor in their marketing application. Health authorities do not want surprises. They are charged with protecting the public health which means that the public needs to be fully aware of any actual or potential safety issues. A thorough development plan will utilize early data such as the MOA and drug distribution to outline potential efficacy and safety effects. Potential safety issues if clinically significant will need to be assessed promptly. The use of imaging to evaluate potential safety issues is increasing. The observation of some fractures during a phase II study in a drug with a theoretical risk of impacting bone metabolism can be assessed by adding a bone mineral density sub-study into phase III. The risk of heart valve dysfunction can be addressed with echocardiography. The risk of direct CNS effects can be mitigated by demonstrating the absence of drug in the CNS using PET. Studies addressing specific safety concerns may be best performed during phase II since the presence or even the probability of certain safety issues can have a major impact on the approval of a product and may therefore influence the decision of whether to proceed into phase III.
In summary, imaging studies can be very useful in understanding how the pharmacological product modifies disease progression. This can lead to better decisions regarding the dosing regimen, the probability of clinically significant safety issues, and, ultimately, whether to progress further in developing the product. In many therapeutic areas imaging studies are required for initial approval or approval of specific indications.
Detection of Disease Progression
Many diseases remain asymptomatic until they are relatively advanced. Examples include atherosclerosis, osteoporosis, and certain malignancies. In many diseases including rheumatoid arthritis, patient symptoms both at the level of the joint and systemically may not correlate with disease progression obtained through imaging studies. Imaging may be considered more objective than documentation of clinical symptoms. This is not surprising since reporting of clinical symptomatology is dependent upon several factors which are difficult to precisely control in the context of a clinical trial.
One major challenge facing pharmaceutical companies is that in certain therapeutic areas, there are already high-quality medicines available to treat the disease. This may mean performing a head-to-head trial against existing options or comparing the existing therapy to a combination of the new and existing therapy. In both situations, the difference in therapeutic effect will be less than a comparison of the new agent to placebo. Since imaging is in essence “a sharper scalpel,” the use of this instrument to demonstrate a relative improvement in either efficacy or safety can greatly modify the use of the product. An increase in progression free survival relative to existing standards of care in oncology is one example. For all late stage clinical development programs, imaging endpoints should be considered in evaluating the BRR relative to existing therapeutic options.
Do the Results of the Imaging Study Answer the Key Scientific Question?
Unfortunately the answer is often not to the degree that is necessary for health authorities. Why is this so? Suleiman and Gorovets in April 2010 presented their observation that the FDA desires scientifically robust evidence. However, many of the imaging trials lacked standardization, calibration, and reproducibility. They compared the standards for drug quality and purity required for chemistry manufacturing control and stated that similar rigor should be applied to imaging [14]. They note that several studies did not have sufficient power to detect a difference between treatment groups due to the large variability observed for the imaging parameter. This becomes particularly relevant when conducting non-inferiority studies for efficacy. It is also pertinent for safety studies because results which show no difference between a drug in development and placebo may not be considered sufficiently robust if there are no data on within subject variability of the imaging parameter to enable determination of study power. If a clinically meaningful difference is not detectable using the imaging technology due to inherent limitations in the technology employed in the study, or due to poor implementation of imaging standards, this will be considered an irrelevant study and will not diminish a safety risk.
Imaging studies are used to measure one or more variables associated with disease progression. This requires attention to detail for each of the steps involved in the process analogous to the manufacturing of marketed drugs. Lack of attention to critical details will result in a study that has similar value to a batch of a pharmaceutical product that does not meet desired product specifications.
Key Considerations for Successful Imaging Studies
Creating a Successful Imaging Team
The clinician(s) within the clinical development team are usually responsible for the task of clinical trial design with input from their statistical colleagues. The key component of a successful imaging trial is the recognition that specialized skills beyond those of the lead clinician and the core development team will be required. These imaging professionals, whether they are within the organization or external, should be involved early in the process of trial design. As a team leader, it is helpful to map out the key questions that will need to be addressed and to seek input from individuals with the appropriate knowledge and experience to provide constructive input. Table 6.2 outlines some key questions that will need to be answered in order to develop the imaging component of a clinical trial and the skills that are required to answer these questions. For illustrative purposes a proposed study evaluating a novel agent for rheumatoid arthritis will be discussed. Management would like to know if this compound will modify disease at the level of the joints prior to investing in a phase III program which will cost in excess of 500 million US dollars.
Table 6.2
Key elements in the design of imaging parameters within clinical trials
Key questions | Relevant parameters/examples | Skills required |
---|---|---|
Endpoint(s) to be measured and selection of imaging modality | Joint space narrowing and bony erosions by X-ray are the regulatory standard for approval in phase III. Synovitis, tenosynovitis, bone marrow edema and bursitis can be detected by MRI or ultrasound | Rate of change over time in the population of interest. Determination of the change that is most likely to be impacted by the treatment over the intended duration of the trial |
Availability of validated metrics for the selected endpoint | Scoring systems | Knowledge regarding test validation and regulatory standards in imaging |
validations conducted | ||
Imaging hardware | Acceptable hardware for imaging of key endpoints | Ability to detect changes in the selected parameters. Differences between available hardware and impact on imaging endpoints |
Image acquisition | Protocol for image acquisition | Performance characteristics of imaging devices |
With or without contrast | Experience with the pros and cons of different acquisition protocols | |
Image type and desired resolution | ||
Precision (reproducibility) | Difference between two measurements from the same patient on the same day | Knowledge of the conduct, analysis, and interpretation of reproducibility studies |
Accuracy | Comparison to gold standard (phantom) | Determination of whether phantoms are required for this study |
Image analysis | Hardware and software | Knowledge of the clinical relevance of differences in hardware and software |
Image interpretation | Read methodologies | See Chap. 5 |
Data management | Identification of key imaging metrics for both operational purposes and statistical analysis | Experience in the therapeutic area with the specific imaging technology and operational experience |
True potential for detecting change | Following selection of the patient population, imaging modality, and image acquisition protocol an estimate of the change that will be demonstrated in the control group and in the treatment groups | Experienced individuals in translating potential for detecting change into an accurate estimate of expected change between groups in the clinical trial |
Imaging Endpoints
In responding to management’s request, there is a multitude of information that must be acquired and processed by the team in order to develop reasonable designs for their phase II study. This includes all of the parameters listed in Table 6.2. Generally, the first aspects of trial design that need to be agreed upon are the key endpoints and the associated imaging technology through which they will be measured.
In rheumatoid arthritis only a small percentage of patients will demonstrate progression of joint damage over a 12-month time period using radiographs. The size of a study using this imaging technology will require 300–500 patients per group [15]. MRI and ultrasound can detect changes in the joint that cannot be detected with standard radiographs. Therefore, changes can be detected at earlier time points and in a greater percentage of patients enabling a smaller sample size.
Another consideration is that as the imaging technology becomes more sensitive to detecting smaller changes, one must determine which specific imaging changes are temporary and reversible and which specific changes represent disease progression. This is part of the evolving advances leading to improvements in standards of care. In drug development, one must also have a firm understanding of what changes are predicted to be improved based on the mechanism of action of the molecule under development, over what time period, and in what patient population? The answers to these questions will be important in the design of the clinical trial program.
Invariably, there will be different answers to these questions based on which literature source is referenced and whose clinical opinion is sought. There will be variability in the imaging data reported depending on the patient population studied, the acquisition method, hardware, software, and reading methodologies. Professionals skilled from a clinical perspective in conducting research in this area can be helpful. Likewise, experienced musculoskeletal imaging professionals especially those who have conducted clinical trials with similar endpoints will provide significant value. Ultimately, one will be required to estimate the incidence of imaging changes, the rate of change over time, and the effect of the pharmacological intervention in the population under investigation in order to design and adequately power the studies.
Say that a preliminary decision is made to proceed with MRI as the imaging modality and the anatomical areas for evaluation include the hands, wrist, and feet joints. Are there scoring systems that are recognized for MRI in rheumatoid arthritis? Are these scoring systems validated and if so by what methods? Are they acceptable to health authorities? In addition to the professionals mentioned previously, individuals experienced in validation and with the evolving regulatory position on imaging endpoints will provide significant value to the team.
Imaging Hardware
Once the imaging modality has been identified, the question of which equipment to use for this clinical trial arises. Manufacturers of MRI scanners improve their products over time. Published literature may be based on single-center studies with scanners that are not currently used by many imaging facilities. Knowledge regarding scanners from different manufacturers and even models within the same manufacturer are relevant. Scanners in use at sites that will be considered for the study will need to be determined. Biomedical engineers can explain these differences. Discussion between the biomedical engineer and clinician will be helpful in making decisions regarding the tradeoff between hardware consistency and models available at potential sites. Manufacturers are usually very willing to have their engineers go through the specifications and performance characteristics of their products. Most will explain to you why their products are superior to those of their competitors. This process can be confusing as it can be difficult to determine how these differences in technical parameters will impact the images acquired for the study. It can also be quite challenging to determine the magnitude of the impact that these differences will have on the imaging endpoints proposed for the study. CROs with professionals experienced in imaging often have staff members who are familiar with manufacturer upgrades and understand the differences including the impact that these differences will have within your trial. Consulting them will save time and get you the information needed in a timely fashion.
Image Acquisition
For purposes of discussion, image acquisition includes all of the steps from when a patient enters the imaging suite until the images are digitally stored. Ideally image acquisition should be identical for all scans in the study. In reality, numerous factors that vary over time limit us to approximating this goal. A standard acquisition protocol must be developed. This includes all of the variables that may impact image metrics. These include patient positioning, slice thickness, image type (e.g., T1 or T2), and use of contrast. Having the same technician perform the scans is the most important variable. Incorporated into a particular technician’s routine is not only positioning but also many other factors involved in their management of the patient through the process. It is good practice to speak with site technicians regarding whether the proposed acquisition protocol is easily understood and reasonable to conduct in their facility.
Precision
Reproducibility refers to the difference obtained between two scans, obtained with the same scanner, by the same technician on the same patient; see Chap. 2 for comparison of precision and accuracy. In some publications this is also referred to as the precision of the measurement. Typically a scan is performed using the study protocol. The patient is instructed to get off the scanner table and a few minutes later the process of performing the second scan is initiated. The difference between the two scans represents the intra-subject variability. This should be performed on a group of patients with different degrees of disease to assess the intra-patient variability across the disease spectrum. These studies are usually performed at 1–3 sites. Good technicians will be able to point out sources of variability within the acquisition protocol. This will serve as the basis for site training that is required to qualify imaging personnel at the site and ongoing training and monitoring procedure to minimize variability between sites [16].
When conducting clinical trials, consistency is extremely important. Even the same scanner will generate different results over time. How does one detect and manage these changes? Also when we get a reading from a scanner, how close to the truth is it? Accuracy is the term used to describe how close a measurement comes to a “gold standard.” Phantoms can be utilized to describe accuracy and to monitor consistency. If phantoms do not exist for the anatomical area under investigation, you may want to consider having one built. This will be a costly procedure, so it is best to discuss this with an experienced imaging professional. Typically phantoms are imaged at regular intervals during a clinical trial in order to detect variation in machine performance over time. Minor changes in machine performance can be managed by applying correction factors to the study images generated, but more significant changes may make some of the images unsuitable for reading. Images which do not meet the predefined study quality standards will require a repeat image for reading. If this does not occur within a specific time period as defined in the protocol, there will be no usable data for this patient. Since imaging endpoints are typically calculated as the change from baseline, the baseline and final images are the most critical. Statistical analysis will commonly be performed using a last observation carried forward methodology. Therefore scans which are missing will tend to reduce the change detected with the effect of reducing study power.
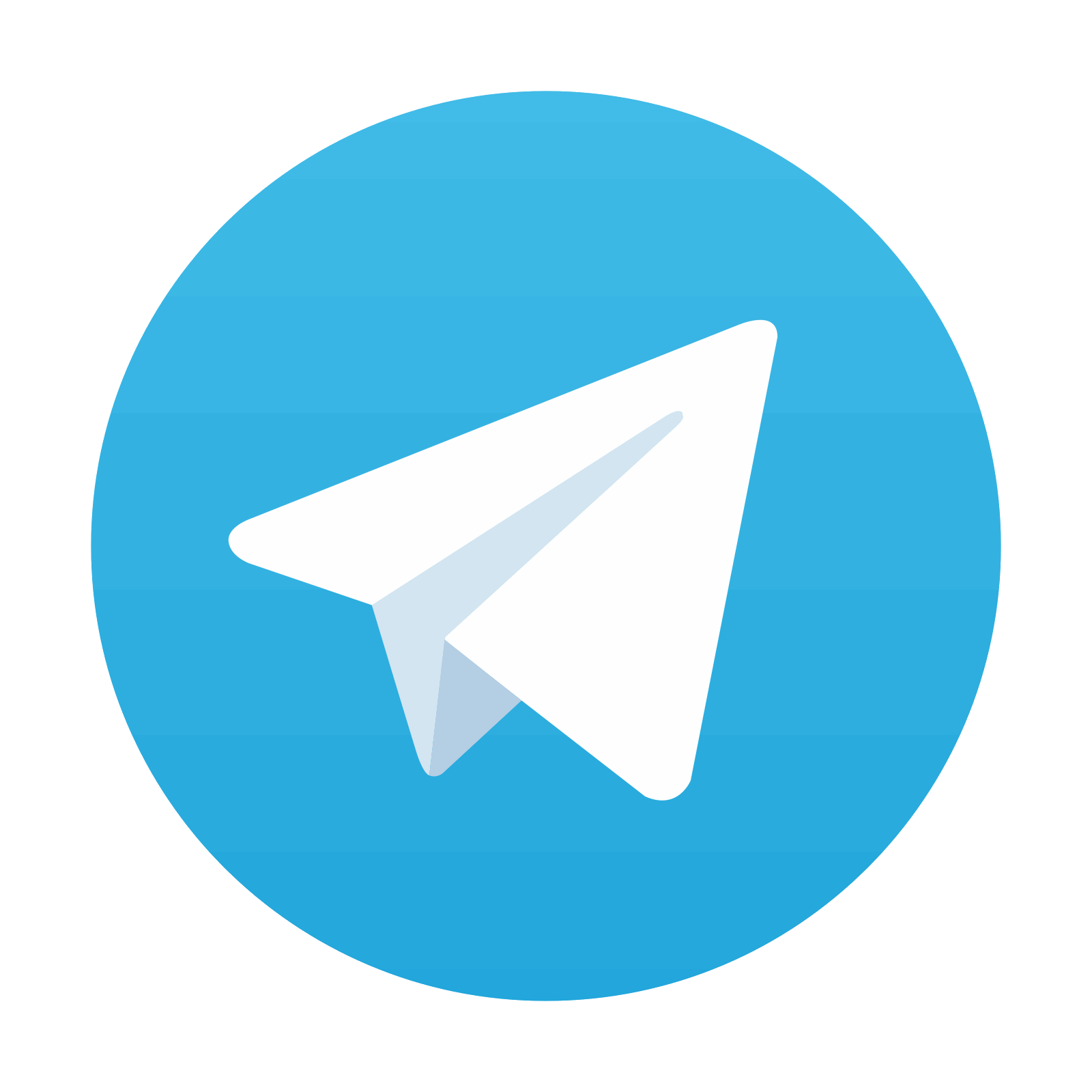
Stay updated, free articles. Join our Telegram channel

Full access? Get Clinical Tree
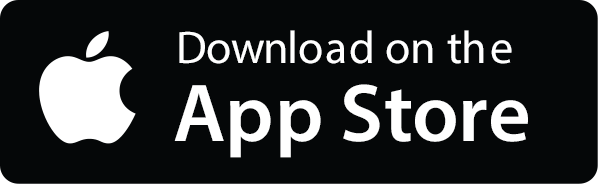
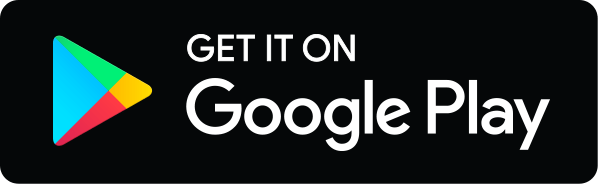