Reference
Number of patients
RPA class I
RPA class II
RPA class III
Gaspar et al. (1997)
1,200
7.1
4.2
2.3
Lutterbach et al. (2002)
916
8.2
4.9
1.8 (IIIA 3.2)
Nieder et al. (2000)
528
10.5
3.5
2.0
Agboola et al. (1998)
125 (resected brain met.)
14.8
9.9
6.0
Tendulkar et al. (2006)
271 (resected single brain met.)
21.4
9.0
8.9
Lorenzoni et al. (2004)
110 (RS)
27.6
10.7
2.8
Sneed et al. (2002)
268 (RS only)
14.0
8.2
5.3
301 (RS + WBRT)
15.2
7.0
5.5
Table 2
Overview of results with the graded prognostic assessment (GPA) score
Study | Median survival class I | Median survival class II | Median survival class III | Median survival class IV |
---|---|---|---|---|
Sperduto et al. (2008a) 1,960 patients who participated in clinical trials | 11.0 | 8.9 | 3.8 | 2.6 |
Nieder et al. (2009) 232 patients treated outside of clinical trials | 10.3 | 5.6 | 3.5 | 1.9 |
Nieder et al. (2008) 64 patients treated with surgery and WBRT | 18.9 | 9.8 | 5.5 | 3.7 |
Sperduto et al. (2008b) 140 patients treated outside of clinical trialsa | 21.7 | 17.5 | 5.9 | 3.0 |
Focal treatment such as SRS improves the local control observed with WBRT. In a small randomized study, patients with two to four brain metastases (all ≤25 mm diameter) either received WBRT alone (30 Gy in 12 fractions) or WBRT plus SRS (Kondziolka et al. 1999). The rate of local failure at 1 year was 100 % after WBRT alone but only 8 % in patients who had boost SRS. Median survival was 7.5 vs. 11 months for patients who received WBRT vs. WBRT plus SRS (p = 0.22). A randomized study by the RTOG enrolled 333 patients with one to three brain metastases (Andrews et al. 2004). WBRT dose was 37.5 Gy in 15 fractions in both groups. SRS boost dose was adjusted to lesion size (15 Gy in lesions larger than 3 cm, 24 Gy in those up to 2 cm, and 18 Gy in others). Median survival was significantly better after SRS boost in patients with single brain metastasis. By post hoc multivariate analysis, survival was also improved in RPA class I patients. SRS-treated patients were more likely to have a stable or improved performance status at 6 months (43 vs. 27 %, p = 0.03). Central imaging review showed higher response rates at 3 months and better 1-year control of the SRS-treated lesions, p = 0.01. The risk of developing a local recurrence was 43 % greater with WBRT alone.
The risk of serious toxicity after WBRT appears rather low, even if prospective studies have demonstrated variable degrees of neurocognitive deficits during extended follow-up (Aoyama et al. 2007; Chang et al. 2009). Furthermore one must acknowledge that any type of cancer treatment might cause measurable neurocognitive decline, including SRS alone (Rugo and Ahles 2003; Heflin et al. 2005; Chang et al. 2009) and that some post-radiation symptoms might be caused by certain drugs rather than radiation itself (Nieder et al. 1999; Klein et al. 2002).
Local control of a limited number (mostly one to three) of brain metastases can effectively be achieved by surgical resection or SRS with or without adjuvant WBRT (Table 3). Recent data suggest that local control can also be achieved with SRS in patients with more numerous metastases, for example, ten or more (Yamamoto et al. 2014). The number of patients dying from uncontrolled brain metastases despite intensive local treatment ranges from 20 to 30 %. In general, SRS doses have varied with lesion size although it is counterintuitive to treat larger tumors with lower doses of radiation. While small lesions typically receive minimum doses of 20–24 Gy to the margin of the lesion, those that measure between 2 and 3 cm are treated with 18–20 Gy and those that measure between 3 and 4 cm with 15–16 Gy and sometimes with doses as low as 12 Gy, based on location. A retrospective analysis of 375 lesions suggests that 1-year local control after 18 Gy or less is in the range of 45–49 % as opposed to 85 % after 24 Gy (Vogelbaum et al. 2006). In the Japanese SRS study of 132 patients treated with lower SRS doses, discussed in greater detail below, only 4 patients (3 %) developed radionecrosis (Aoyama et al. 2006). Prognosis of SRS patients might be estimated either by RPA classes, DS-GPA, or the score index for radiosurgery (SIR) (Weltman et al. 2001; Lorenzoni et al. 2004). The most favorable SIR group contains patients with age ≤50 years, Karnofsky performance status (KPS) >70 %, no evidence of systemic disease at the time of SRS, limited number of brain lesions, and largest SRS-treated lesion <13 ml. After many years of controversy about the role of combining WBRT with SRS and considerable variation in practice, comparable to the discussion around WBRT after surgical resection of brain metastases, four randomized trials and a meta-analysis have attempted to address the issue (Aoyama et al. 2006; Chang et al. 2009; Kocher et al. 2011; Sahgal et al. 2015; Brown et al. 2015). The Japanese prospective randomized multicenter phase III study of SRS alone vs. SRS and WBRT (Aoyama et al. 2006) was designed with the primary endpoint of survival, with an overly generous expected difference of 30 %. The trial included adult patients with Karnofsky performance score >60 % and a maximum of four brain metastases, none exceeding 3 cm diameter. WBRT was given in 10 fractions of 3 Gy. SRS dose varied with size of the lesion (up to 2 cm, 22–25 Gy; >2 cm, 18–20 Gy margin dose) and was reduced by 30 % if WBRT was given. The combined arm contained 65 patients, the SRS arm 67 patients. Almost 50 % of patients had a single lesion. Median survival was 7.5 months after SRS plus WBRT and 8 months after SRS alone. One-year survival in the combined treatment arm was actually relatively increased by 36 %, but this did not reach statistical significance due to low patient numbers (38.5 vs. 28.4 %, p > 0.05). After SRS alone, 2 patients developed serious late complications (radionecrosis and grade 4 seizures, respectively). After SRS plus WBRT, 3 patients developed a radionecrosis, and 3 showed signs of leukoencephalopathy. The trial revealed statistically significant differences in local control. The rate of actuarial failure at 1 year was 47 % after combined treatment but significantly greater at 76 % after SRS alone (relative increase of 62 %; p < 0.001). New lesions developed in 42 vs. 64 % (of SRS alone patients) (p = 0.003). WBRT reduced the risk of failure at the site of SRS from 27 to 11 % after 1 year (p = 0.002).
Table 3
Results of surgery and stereotactic radiosurgery (SRS) for brain metastases
Reference | n (patients and lesions) | Prescribed dose (median; range [Gy])a | Median OS | 1-year PFS (%) |
---|---|---|---|---|
Patchell et al. (1990) | 25/25 | Surgery | 9.5 | 80 |
Patchell et al. (1998) | 49/49 | Surgery | 11.0 | 82 |
Pirzkall et al. (1998) | 236/311 | 20; 10–30 | 5.5 | 89 |
Cho et al. (1998) | 73/136 | 17.5; 6–50 | 7.8 | 80 |
Kocher et al. (1998) | 106/157 | 20; 12–25 | 8.0 | 85 |
Sneed et al. (1999) | 62/118b 43/117c | 18; 15–22 17.5; 15–22 | 11.3 11.1 | 80 86 |
Varlotto et al. (2003) | 137/208 | 16; 12–25 | Not given | 90 |
Andrews et al. (2004) | 164/269d | Not given; 15–24 | 6.5 | 82 |
Bhatnagar et al. (2007) | 205/4–18 lesions eache | 16; 12–20 | 8.0 | 71 |
A recent reanalysis of this trial has further fueled the survival debate. Based on a handful of retrospective reviews, substantially underpowered prospective trials, and a meta-analysis based on these underpowered trials, it has been widely concluded that omission of WBRT does not decrease overall survival (OS), primarily because salvage therapies are effective, and systemic progression is the key competing cause of mortality (Sahgal et al. 2015). This assertion may perhaps be true, but diligent review of the available data would caution against jumping to such a conclusion on the basis of the relative weakness of the supporting data, as well as the recent emergence of contradictory data from the aforementioned Japanese trial. An analysis of three pieces of data in the literature should induce a degree of interpretive caution. As early as 1998, Pirzkall et al. reported a single-institution 236-patient retrospective experience of SRS with or without WBRT, demonstrating a trend for superior survival (OS) in favor of WBRT (1- and 2-year OS of 30 vs. 19 and 14 vs. 8 %), but much more impressive was the recognition that in patients without extracranial disease, i.e., in those in whom systemic progression as a competing cause of mortality is largely diminished, the median survival was impressively different at 15.4 vs. 8.3 months, in favor of WBRT (reaching only borderline significance because of the small numbers). This allows one to posit the very reasonable hypothesis that a certain proportion of patients with brain metastases are destined to succumb to intracranial progression (after all we see such compartmental progression as a cause of death in other organs such as the lungs, liver, etc.) and enhanced control of intracranial progression will lengthen their survival.
Finally, a recent reanalysis of the randomized Japanese JROSG-99 trial, using the validated graded prognostic assessment (GPA) stratification model and applied to all non-small cell lung cancer patients on the trial, reveals a median survival of 16.7 versus 10.6 months in favor of the WBRT + SRS arm (vs. SRS alone, p = 0.03) for the favorable (GPA = 2.5–4) subgroup, without demonstrating an advantage for the inferior prognosis group, providing further support that intracranial control matters and one accepts a lower rate at the potential peril of diminishing overall survival (Aoyama et al. 2006).
A European phase III trial (EORTC 22952-26001) included 359 patients, 199 underwent SRS, and 160 underwent surgery (Kocher et al. 2011). In the SRS group, 100 patients were allocated to observation, and 99 were allocated to WBRT. After surgery, 79 patients were allocated to observation, and 81 were allocated to adjuvant WBRT. The median time to WHO performance status more than 2 was 10.0 months (95 % CI, 8.1–11.7 months) after observation and 9.5 months (95 % CI, 7.8–11.9 months) after WBRT (p = 0.7). Overall survival was similar in the two arms (median, 10.9 vs. 10.7 months, p = 0.9). WBRT reduced the 2-year relapse rate both at initial sites (surgery, 59–27 %, p < 0.001; SRS, 31–19 %, p = 0.04) and at new sites (surgery, 42–23 %, p = 0.008; SRS, 48–33 %, p = 0.02). Salvage therapies were used more frequently after observation than after WBRT. Intracranial progression caused death in 44 % of patients in the observation arm and in 28 % of patients in the WBRT arm.
The randomized trial from the M.D. Anderson Cancer Center re-emphasized patient selection issues as critical for overall survival. In this trial, patients with one to three newly diagnosed brain metastases were randomly assigned to SRS plus WBRT or SRS alone, and over an almost 7-year time frame, 58 patients were recruited and stratified by RPA class, number of brain metastases, and histology (Chang et al. 2009). The primary endpoint was neurocognitive function: measured as a 5-point drop compared with baseline in Hopkins Verbal Learning Test-Revised (HVLT-R) total recall at 4 months. An interim analysis showed that there was a high probability (96 %) that patients assigned to receive SRS plus WBRT were more likely to show a decline in learning and memory function at 4 months than patients assigned to receive SRS alone. Further, at 4 months there were four deaths (13 %) in the group that received SRS alone, and eight deaths (29 %) in the group that received SRS plus WBRT, and 73 % of patients in the SRS plus WBRT group were free from CNS recurrence at 1 year, compared with 27 % of patients who received SRS alone (p = 0.0003). These differences in early death bring into question the generalizability of the HVLT-R score results; it is well known that a general disease-related decline due to progression, especially in the preterminal phase, will cause a significant drop in neurocognitive function, and its attribution to a single component, such as WBRT, can be misleading. Early deaths in neuro-oncology are almost invariably consequential to systemic progression of disease in this setting. In fact, there were several differences in patient characteristics between the two cohorts which could explain both the early deaths and the differences in 4-month HVLT-R scores. When the constellation of prognostic factors is evaluated collectively, the SRS alone group, compared to SRS plus WBRT, had far more favorable characteristics, such as more female patients (60 vs. 39 %), fewer patients with multiple brain metastases (40 vs. 46 %), lower intracranial disease burden (1.4 vs. 2.3 cc), superior RPA (23 vs. 11 % RPA Class 1) and GPA (10 vs. 3.5 % GPA score 3.5) distribution, fewer patients with liver metastases (7 vs. 18 %), etc.; the small patient numbers precluded any of these factors from individually reaching statistical significance, but taken collectively, the prognostic variables were substantially skewed in favor of the SRS group. As would be expected from the use of WBRT, the 1-year local tumor control rate was 67 % for patients in the SRS group but considerably superior at 100 % for patients in the SRS plus WBRT group, and additionally, the 1-year distant brain tumor control rate was 45 % for patients in the SRS group and 73 % for patients in the SRS plus WBRT group. The 1-year freedom from CNS recurrence was 27 % (95 % CI 14–51) for SRS alone and 73 % (46–100) for SRS plus WBRT. This trial therefore emphasizes three crucial points when evaluating brain metastases data:
- 1.
Local control as well as distant control in the brain is significantly improved by WBRT as an adjunct to focal therapies.
- 2.
Patient selection variables can significantly skew neurocognitive and survival outcomes, and small trials are unlikely to statistically pick up these differences in patient prognostic variables.
- 3.
Early decline in some neurocognitive functions, such as memory recall as measured by HVLT-R, can be impacted by several variables, including WBRT, and the early decline is suggestive of an “early-responding” cell population.
2 Reirradiation: Whole-Brain Radiotherapy
The key issues guiding clinicians in the first-line setting remain important in selecting appropriate management options for patients who relapse after brain irradiation (Table 4). However, few prospective clinical studies formally addressing the role of reirradiation for brain metastases have been published. Salvage WBRT after previous SRS is a common treatment option with survival results indistinguishable from those of first-line WBRT, i.e., usually 3–6 months median survival (Khuntia et al. 2006). A repeat course of WBRT is less commonly employed due to concerns about lack of efficacy and the potential for neurocognitive deficits. Historical experience with WBRT dates back to a retrospective study by Shehata et al. (1974) and another study by Kurup et al. (1980), which will not be reviewed in greater detail. Both are limited by the fact that they date back to the pre-CT era and few systemic treatment options existed at that time. Thus, rapid progression of systemic disease was an even bigger problem than it is now. The first study extending into the CT era, but pre-dating the advent of SRS salvage for recurrence, was reported in 1988 (Hazuka and Kinzie 1988). It included 44 patients (34 % with non-small cell and 20 % with small cell lung cancer), all of whom had previously received WBRT for brain metastases. The reasons for retreatment with WBRT (and in a small number of patients, large-volume partial brain reirradiation) were the appearance of new intracranial lesions (47 %), new lesions plus progression of pre-existing metastases (10 %), and local progression of pre-existing metastases only (43 %). The median interval between initial WBRT and reirradiation was 8 months, with a minimum of 8 weeks. The median initial dose was 30 Gy in 10 fractions of 3 Gy, and the median retreatment dose was 25 Gy (range 6–36 Gy, dose per fraction 2–4 Gy). Median survival after repeat WBRT was only 8 weeks. Partial neurological improvement was observed in 27 % of patients. Two patients most likely died as a direct consequence of brain necrosis (brain necropsy result). Both were treated to rather high cumulative doses, especially if one calculates biologically equivalent doses. In one case, WBRT to 32 Gy in 8 fractions of 4 Gy was followed by WBRT to 30 Gy in 10 fractions of 3 Gy (necrosis after 20 weeks from reirradiation). In the other case, WBRT to 30 Gy in 10 fractions of 3 Gy was followed by partial brain RT to 33 Gy in 10 fractions of 3 Gy (necrosis after 11 weeks from reirradiation). The reirradiation tolerance of the human brain is reviewed in detail in other chapters of this book.
Table 4
Key questions when selecting between the different treatment options for recurrent brain metastases
Is the patient’s performance status after initiation of steroid treatment at a level that justifies initiation of radiation therapy? |
Do laboratory tests point to advanced extracranial disease status and poor tolerability/efficacy of the planned therapy? |
Are extracranial disease sites absent or controlled, and if so, does one expect continued extracranial disease control? |
Will systemic treatment be offered or are there no more options left? |
Will brain control impact on the survival of the patient or is treatment focused on palliation of symptoms? |
Will surgical intervention lead to rapid symptom improvement or effective local control, if comorbidity and other factors allow for consideration of invasive measures? Could the same goals be achieved without surgery? |
Might the cumulative radiation dose to critical normal tissue structures result in serious toxicity in patients with expect prolonged survival? |
What would be the functional consequence of treatment-induced injury? |
How did the lesion(s) respond to initial radiotherapy and how long is the interval? |
Limited, but more recent experience with 2 courses of WBRT in 72 patients, the majority with primary lung cancers suggests that 31 % of patients experienced a partial clinical response after reirradiation (Sadikov et al. 2007). In responders, the mean duration of response was 5.1 months. The median survival after reirradiation was 4.1 months. One patient was reported as having memory impairment and pituitary insufficiency after 5 months of progression-free survival. However, assessment of toxicity in this and other similar series is hampered by their retrospective nature and the poor performance status of most patients. The most frequent dose used for the initial radiotherapy was 20 Gy in 5 fractions. The most common reirradiation schema were 25 Gy in 10 fractions, 20 Gy in 10 fractions, and 15 Gy in 5 fractions. Median interval between the two courses of brain radiation was 9.6 months, with a minimum 8 weeks. The typical patient had a performance status of 1 or 2. Patients with better performance status experienced significantly longer survival after reirradiation, comparable to the study by Aktan et al. (2015; median 2.2 months if KPS ≤70; 5.3 months for all 34 patients). In initial nonresponders, median survival was only 0.9 months after reirradiation, implying that this might be a crucial variable to consider. Surprisingly, the interval between the two courses had no impact on survival.
In another retrospective series of 52 patients, a slightly better clinical response rate (42 %) as well as better median overall survival (almost 5 months) was reported (Cooper et al. 1990). The major difference and potential explanation were that patients were offered reirradiation only if they maintained good general condition for at least 4 months after initial WBRT (median 30 Gy in 10 fractions of 3 Gy), excluding nonresponders, and patients experiencing early decline. The most common reirradiation regimen was 25 Gy in 10 fractions of 2.5 Gy.
Another series, published in 1996 by Wong et al. (86 reirradiated patients including 18 with partial brain fields), included an equal number of lung and breast cancer patients (31 each). The median dose of initial WBRT was 30 Gy, usually given in 10 fractions. The median interval to reirradiation was 7.6 months, with a minimum of 6 weeks. The median dose of reirradiation was 20 Gy, with a maximum 30.6 Gy. Complete or partial symptomatic neurological improvement was observed in 27 and 43 % of patients, respectively. The median response duration was 2.8 months. Median survival was 4 months. The only significant prognostic factor for survival was the absence of extracranial metastases. Scharp et al. (2014) analyzed 134 patients, of whom 60 were treated with initial prophylactic WBRT (87 % had lung cancer). The median interval was 13 months (minimum 3) and the median doses 30 plus 20 Gy, both in 2-Gy fractions. Median survival was 2.8 months, and clinical improvement was observed in 39 % of patients. Significantly shorter survival was seen in patients with small cell lung cancer, KPS <70, or progressive primary tumor. A series of 49 patients was reported from Guo et al. (2014). Median interval was 11.5 months (minimum 1.5 months), median initial dose 30 Gy, and median repeat dose 20 Gy. Median KPS was 70. Improved symptoms were reported in 27 %, and median survival was 3 months. Comparable results were reported by Ozgen et al. (2013); median survival in 28 patients was 3 months and symptomatic response rate 39 %.
Minniti et al. (2014) combined reirradiation (25 Gy, 10 fractions) with concurrent temozolomide (75 mg/m2). They treated 27 patients whose median age was 54 years. Minimum KPS was 60. Eighteen patients had lung cancer. Median survival was 6.2 months. Seventeen patients (63 %) had improved symptoms. Severe toxicity was not observed. Survival was significantly longer in patients with stable or absent extracranial disease. Survival was slightly better than in other studies, but interstudy comparison is hampered by the heterogeneity of the different study populations. Without randomized trials, the role of temozolomide is difficult to define.
Overall, the studies reviewed here reported median survival of 2–6.2 months (median 4.0) and improvement of symptoms in 27–70 % of patients (median 35 %). Shorter survival was seen in patients with KPS <70, progressive primary tumor, or extracranial metastases.
Helical tomotherapy can also be utilized in patients who develop multiple brain metastases in spite of previous WBRT (Sterzing et al. 2009). Both patients treated with this technique had previously received 40 Gy in 20 fractions of 2 Gy. The whole-brain reirradiation dose was limited to 15 Gy, while the enhancing lesions plus a 2-mm margin received 30 Gy in 10 fractions of 3 Gy. In the first case, 8 metastases from breast cancer were present 18 months after first-line WBRT. With a follow-up of 12 months, local control was achieved. In the second case, 11 metastases from non-small cell lung cancer were present 18 months after initial WBRT. With a follow-up of 6 months, local control was achieved. No serious toxicity was recorded. In Fig. 1, we show an example of a patient with multiple recurrent brain metastases from breast cancer treated with tomotherapy. The patient had received two prior courses of WBRT, initially 30 Gy in 10 fractions and then 25 Gy in 10 fractions both achieving complete responses; five subsequent individual recurrences were treated with two courses of SRS, also resulting in complete response; the tomotherapy IMRT plan was utilized for 9 new lesions, and the dose was 30 Gy in 15 fractions; most of the normal brain was kept below 10 Gy, and the patient has sustained local control more than 8 months after this course of therapy and for over 42 months since initial presentation with brain metastases. The case illustrates that with modern and advanced radiotherapy techniques, innovative salvage options become possible, and anecdotally, in selected patients, local control and durable survival are achieved. Figures 2, 3, and 4 provide treatment details regarding three other patients at one of the authors’ institutions, utilizing other unique radiotherapy approaches.
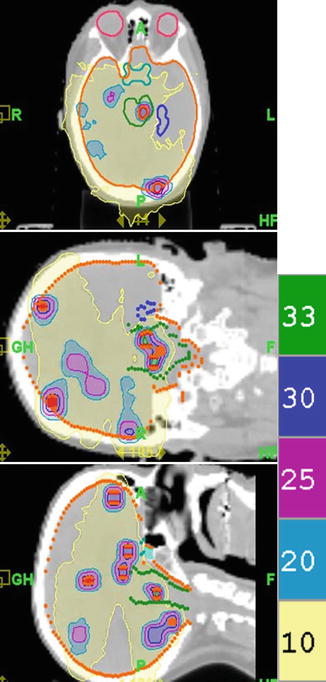
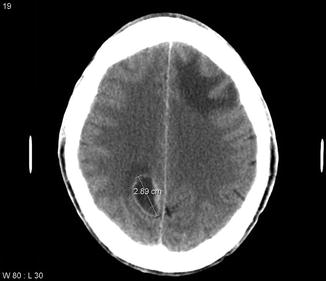
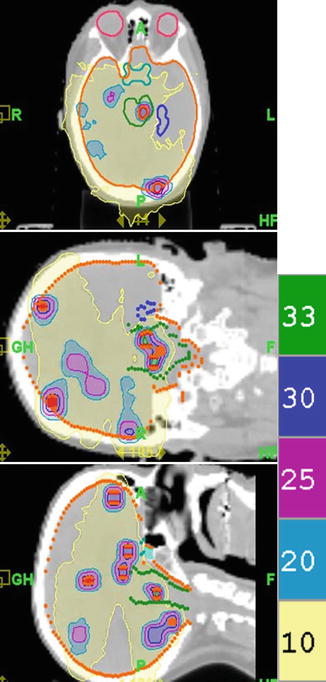
Fig. 1
An example of a patient with multiple recurrent brain metastases from breast cancer treated with tomotherapy. The patient had received two prior courses of WBRT, initially 30 Gy in 10 fractions and then 25 Gy in 10 fractions both achieving complete responses; five subsequent individual recurrences were treated with two courses of radiosurgery, also resulting in complete response; the tomotherapy IMRT plan was utilized for nine new lesions, and the dose was 30 Gy in 15 fractions; most of the normal brain was kept below 10 Gy, and the patient has sustained local control more than 8 months after this course of therapy and for over 42 months since initial presentation with brain metastases
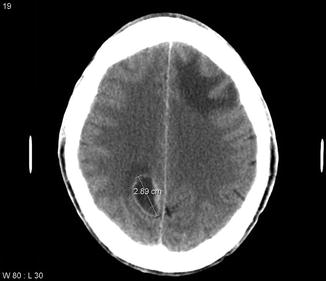
Fig. 2
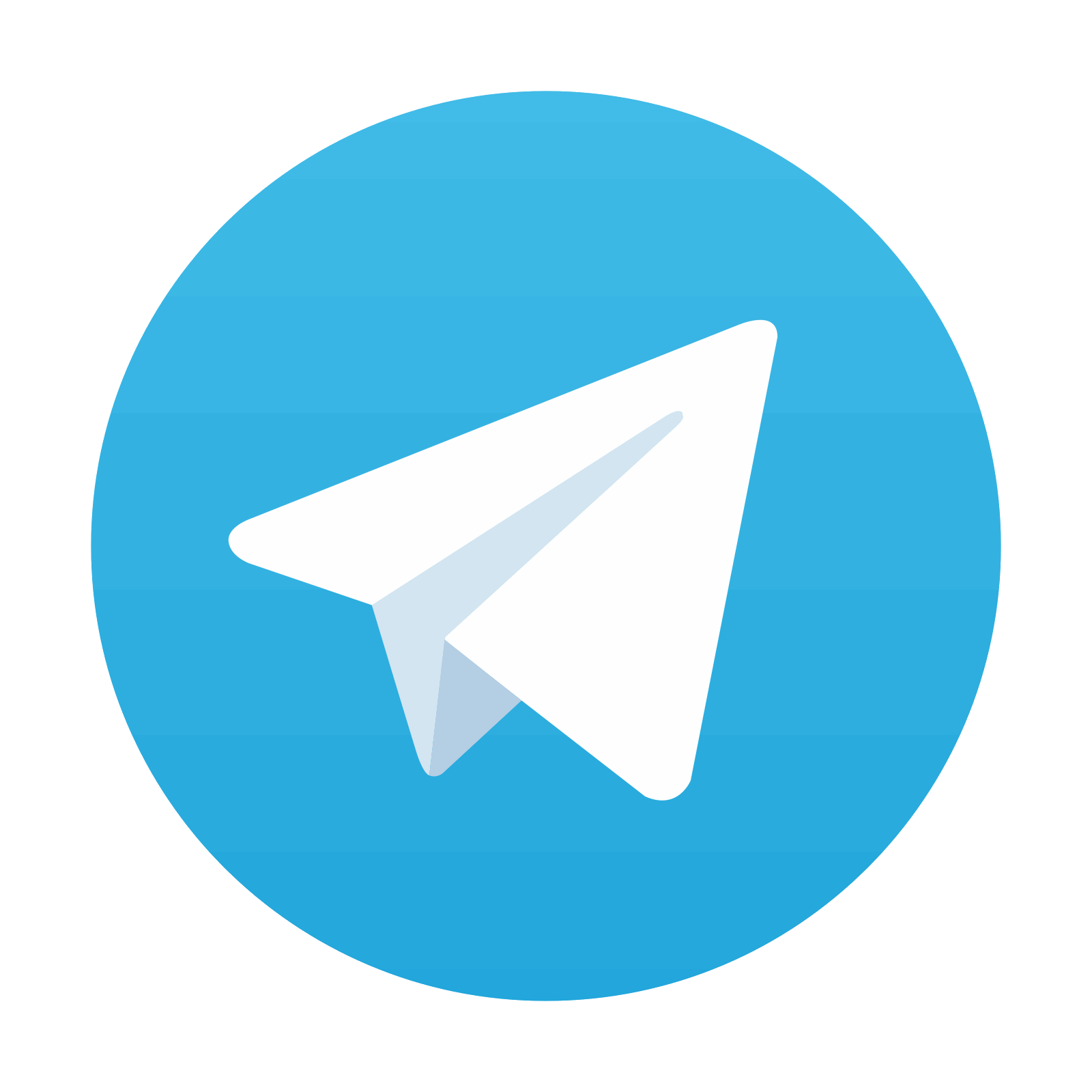
An illustrative case from one of the authors’ institutions (Nordland Hospital Bodø, Norway). A 63-year-old male patient was diagnosed with squamous cell lung cancer stage III B in December 2007. He received systemic platinum-based chemotherapy and thoracic radiotherapy. In November 2008, the patient collapsed, and a computed tomography (CT) scan of the brain revealed four brain metastases, maximum diameter 3.1 cm. No extracranial metastases were detected; all laboratory tests were unremarkable. The intrathoracic status was judged to be ongoing partial remission. The patients Karnofsky performance status (KPS) at that time was 70. Whole-brain radiotherapy (WBRT) was administered (10 fractions of 3 Gy). Three months later, CT scans of the brain showed partial remission of all four lesions. However, another 3 months later, all 4 lesions had increased in size. No additional new brain metastases were detected. The patient was referred for salvage treatment. When considering the key questions presented in Table 4, the following statements could be made.
Is the patient’s performance status after initiation of steroid treatment at a level that justifies initiation of radiation therapy? Yes, the KPS at the time of progression was 70.
Do laboratory tests point to advanced extracranial disease status and poor tolerability/efficacy of the planned therapy? No, the only abnormal finding was slight anemia.
Are extracranial disease sites absent or controlled, and if so, does one expect continued extracranial disease control? No extracranial metastases were detected, but the primary tumor had increased slightly (less than 25 %, no clinical symptoms).
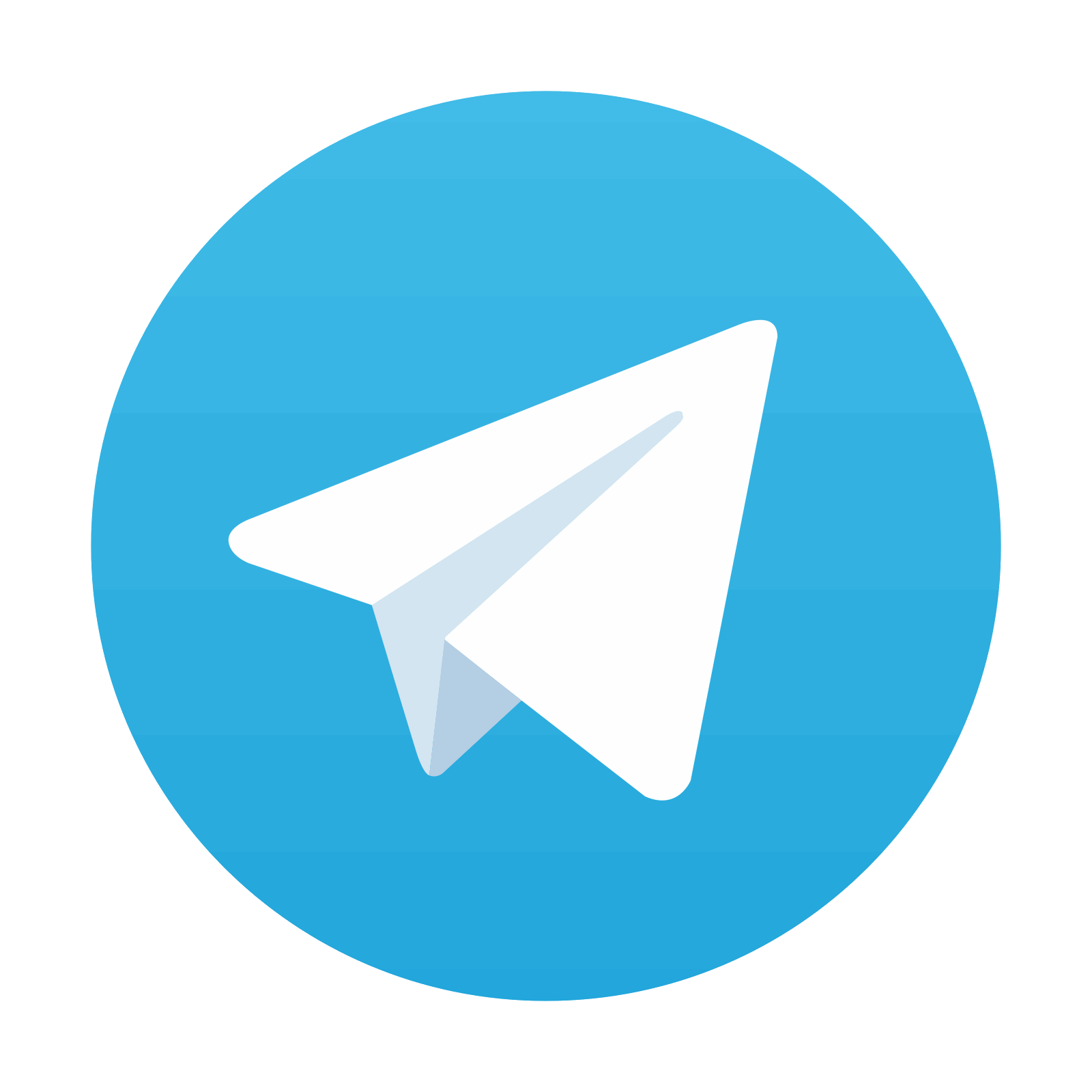
Stay updated, free articles. Join our Telegram channel

Full access? Get Clinical Tree
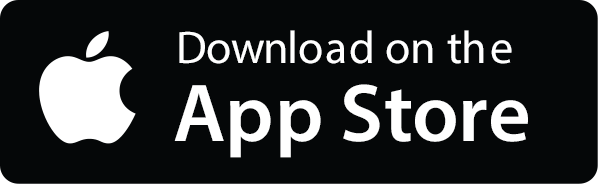
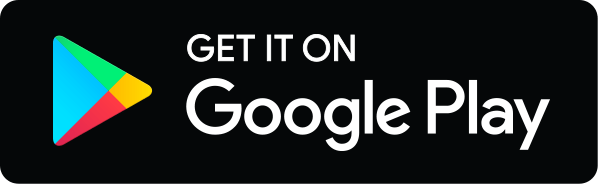
