Fig. 14.1
Overview of the factors influencing small HC volumes and the consequence of HC volume changes in MDD: there is evidence that genetic polymorphisms and early life stress may contribute to HC volumes prior to onset of illness. Repeated episodes of illness may further contribute to loss of HC volume via stress toxicity, reduced neurotrophic factors, and excess in glutamatergic neurotransmission on hippocampal neurons. The underlying molecular basis is an ongoing matter of research. Speculatively, the changes in the HC may then contribute to treatment resistance or chronicity and may further increase the vulnerability for the disease. Consistent with this are studies reporting that small HC volumes predict poor short- and long-term responsiveness to treatment and a higher vulnerability. A more chronic course of disease and relapses seem to have further effects on neural connectivity and might result in further structural changes (Reprinted courtesy of Molecular Psychiatry, with permission (MacQueen and Frodl 2011))
14.3 Functional Imaging in MDD
fMRI studies examining neural responses to emotional stimuli in patients with major depression (MDD) indicated increased responses in the amygdala, anterior cingulate cortex (ACC), fusiform gyrus, putamen, and prefrontal cortical regions (Fu et al. 2004; Surguladze et al. 2005; Frodl et al. 2007a, b). Since many researchers assume that the depressive syndrome might arise from abnormal interactions between brain regions, functional neuroimaging studies have examined the connectivity of the neural network. With respect to connectivity a study in 15 unmedicated patients with major depression and 15 healthy volunteers found decreased correlations between ACC and limbic regions, which is consistent with the hypothesis that decreased cortical regulation of limbic activation in response to negative stimuli may be present in depression (Anand et al. 2005). Again the amygdala was negatively coupled with the ACC but also positively coupled bilaterally with medial temporal and ventral occipital regions in 19 unmedicated patients with major depression and 19 healthy volunteers (Chen et al. 2008). Studies on functional connectivity in patients with MDD receiving antidepressant medication achieved varied results. The results indicated that a neural network consisting of the cingulate region, prefrontal cortical regions, amygdala, and subcortical regions may play key roles in MDD: compared to healthy controls, patients with depression showed increased functional connectivity between the amygdala, hippocampus, and caudate–putamen regions during emotion processing (Hamilton and Gotlib 2008) but significantly reduced amygdala–prefrontal connectivity (Dannlowski et al. 2009). Uncoupling of the prefrontal cortex and gyrus cinguli was found in 14 patients with MDD and 14 healthy controls during a verbal working memory task (Vasic et al. 2008). Resting-state fMRI showed that subgenual cingulate and thalamic functional connectivity was significantly increased in 20 patients with MDD than in 20 healthy controls (Greicius et al. 2009). In 25 drug-free patients with MDD compared to 15 healthy controls, dorsal anterior cingulate cortex, precuneus, and cerebellum activity showed less connectivity with the OFC in patients than in controls, while functional connectivity between the OFC and the right dorsolateral prefrontal cortex (DLPFC), right inferior frontal operculum, and left motor areas was increased in patients than in healthy controls (Frodl et al. 2010a, b, c).
Interest is growing in the use of resting-state fMRI, which does not require the use of a task and which has become a popular means of complementing the results of task-based fMRI studies. Resting-state fMRI allows for the examination of large-scale neural systems that exhibit spontaneous synchronous fluctuations during goal-directed and non-goal-directed behavior (Castellanos and Proal 2012). These low-frequency (<0.1 Hz) spontaneous fluctuations in blood oxygenated level dependent (BOLD) signal correlate with interactions between adjacent and nonadjacent brain areas that form spatially distributed networks of brain function (Raichle et al. 2001). Thus, functional connectivity is the observed correlation in spontaneous neural activity between brain areas at rest (Deco and Corbetta 2011). Several resting-state studies have found increased resting-state functional connectivity in the cognitive control network (Zhou et al. 2010; Sheline et al. 2010), increased default mode network (DMN) connectivity (Zhou et al. 2010; Grimm et al. 2009; Sheline et al. 2010), as well as increased functional connectivity in the affective network (Sheline et al. 2010).
14.4 Diffusion Imaging in MDD
Structural neuroimaging studies in MDD show volume reductions, and functional imaging studies indicate dysconnectivity in limbic and frontal brain regions. Whether white matter fiber bundles between limbic and frontal brain regions are altered can be investigated with diffusion tensor imaging (DTI). Recent DTI studies have suggested that there is a strong correlation between depression and reduced fractional anisotropy (FA) with the nature of this relationship representing a topic of great interest. A study comparing 13 patients with late-life depression to matched healthy controls found a reduction in FA in both the frontal and temporal lobes of depressed patients (Nobuhara et al. 2006). In addition, an inverse relationship was established between FA values and symptom severity (Nobuhara et al. 2006). Another recent study conducted in MDD patients using whole-brain DTI analysis found reduced FA in the left striatum, right cingulate cortex, and posterior body of the corpus callosum, areas of the brain believed to play an important role in emotional regulation (Kieseppä et al. 2010). Importantly, reductions in FA have also been associated with early life adversity in the form of disrupted maternal–infant attachment and correlate with an increased risk of both anxiety and depression (Coplan et al. 2010). A study comparing 12 maternally deprived adult male macaques to nine normally reared controls found significant reductions in FA in the anterior limb of the internal capsule in the maternally deprived macaques (Coplan et al. 2010). This is another brain region important in emotional regulation and is involved in the medial and the basolateral limbic circuits (Coplan et al. 2010). Thus, disruption of this region may alter functional connectivity between the frontal and temporal lobes conferring an increased risk of MDD (Coplan et al. 2010). Another study demonstrating the microstructural implications of early life adversity found significantly reduced FA within the genu of the corpus callosum among those exposed to high levels of early life stress (Paul et al. 2008). DTI represents the forefront of neuroimaging techniques in the characterization of microstructural alterations occurring in the brain both as an antecedent to and as a consequence of depression (Frodl et al. 2012b). These findings appear to be heterogeneous, and therefore, we conducted a meta-analysis over seven available studies including 188 patients with MDD and 221 healthy control patients with depression which showed decreased white matter FA values in the superior longitudinal fasciculus (SLF) and increased FA values in the fronto-occipital fasciculus (FOF) compared to controls. In conclusion, the meta-analysis revealed a significant reduction in FA values in the left SLF, which may ultimately play an important role in the pathology of depression. More research in larger samples is needed to particularly track changes during the disease course using DTI.
14.5 Monitoring Treatment Effects
Functional magnetic resonance imaging (fMRI) is becoming established as a method of visualizing the action of drugs on animal and human brain; in this context it is called pharmacoMRI or phMRI. Longitudinal functional imaging studies (without a comparison treatment arm) that investigated changes in the brains of patients with MDD after treatment with an antidepressant medication have found different results. Only a few studies have tried to elucidate medication effects on the structure of the brain. Increases in hippocampal volume following treatment with the antidepressants sertraline and paroxetine have been reported in patients with post-traumatic stress disorder (PTSD) (Vermetten et al. 2003; Bremner and Vermetten 2004). In MDD, the results are less clear, with some studies failing to find an association between antidepressant therapy and hippocampal volumes (Vythilingam et al. 2004). One study, however, found a trend toward increases in hippocampal volumes in patients who had declines in cortisol levels under pharmacotherapy with either amitriptyline or paroxetine (Colla et al. 2007). In a longitudinal 3-year follow-up study, it was found that continuous antidepressant treatment was associated with an increase in hippocampal volumes (Frodl et al. 2008a, b, c, d). In another longitudinal 8-year follow-up study, 31 remitted former inpatients with moderate to severe MDD showed no differences in hippocampal volume compared to 37 healthy comparison subjects (Hviid et al. 2010). However, a more recent study in 15 young patients with MDD has shown an association between antidepressant treatments and increased posterior hippocampal volumes correlating with improved performance in neuropsychological tests (Schermuly et al. 2011). Moreover, the antidepressant duloxetine increased gray matter volume in the left inferior frontal cortex, right fusiform gyrus, and right cerebellar areas after a 6-week therapy with 60 mg duloxetine in 15 patients with MDD and panic disorder compared to healthy controls (Lai and Hsu 2011). Studies in larger samples with controlled designs will be necessary to elucidate the effect of antidepressants and psychotherapy on brain structure.
fMRI showed evidence that altered brain function normalizes during effective treatment against depression. In 19 patients with major depressive disorder (MDD) treated with fluoxetine for 8 weeks, BOLD responses decreased significantly not only in the basal ganglia and thalamus regions but also in the amygdala, ACC, insula, precentral and postcentral gyrus, and inferior parietal lobule (Fu et al. 2004). Moreover, in this sample, treatment with fluoxetine was associated with a significant increase in functional coupling between the amygdala and subgenual ACC (Chen et al. 2008). Effects on amygdala activation were also found in other studies; for example, exaggerated left amygdala activation in 11 patients with major depression during a face-matching paradigm decreased to a normal activation level following treatment with the SSRI sertraline (Sheline et al. 2001). This effect may have been a direct effect of the antidepressant: after 21 days of treatment with escitalopram, 13 healthy volunteers without depression showed less activation in the amygdala when shown fearful faces than when shown control shapes (Arce et al. 2008). Only one earlier study in 12 patients with MDD found significantly increased activation in the left insular cortex after 2 weeks of treatment with venlafaxine and in the left anterior cingulate cortex after 8 weeks of treatment (Davidson et al. 2003). Thus, the majority of studies of SSRIs found decreased BOLD responses in cortical and subcortical brain regions. Patients receiving mirtazapine showed a significantly different pattern of changes: these patients showed more BOLD responses after 4 weeks’ treatment than before in the left and right MCC and the left and right supplementary motor area (SMA), which indicates that mirtazapine had some stimulating effects in these brain areas (Frodl et al. 2011). A recent pharmacological MRI study in 45 healthy male volunteers who were randomly allocated to receive mirtazapine or placebo in a double-blind fashion found increased activation in the right orbitofrontal cortex after a single oral dose of mirtazapine (Vollm et al. 2006).
14.6 Predicting Therapy Response
Interestingly, the neural correlates of emotional processing in MDD may have predictive value in determining which patients will respond to treatment. The first evidence came from positron emission tomography (PET) which suggests that the subgenual ACC (sgACC) may be relevant in determining biomarkers for treatment response. Differential metabolism in the sgACC predicts response to antidepressant treatment (Mayberg et al. 1997; Wu et al. 1999), an observation which has led to the utilization of this area as a target for deep brain stimulation in chronically treatment-resistant depression (Mayberg et al. 2005). Other regions are also interesting with respect to treatment response. Decreased metabolism in the insular cortex was found by Mayberg et al. (1999) and Kennedy et al. (2001) to be associated with response to treatment in patients with MDD. In addition, such functional alterations are not limited to the use of psychotropic medications for the treatment of MDD. Response to cognitive behavioral therapy (CBT) has been linked to metabolic increases in the hippocampus and poster cingulate (pCC) (BA 24) and decreases in the dorsal (BA 9/46), ventral (BA 47/11), and medial (BA 9/10/11) frontal cortices (Goldapple et al. 2004). Moreover, amygdala hyperactivation and ACC hypoactivation during fMRI predicted response to CBT (Siegle et al. 2006; Fu et al. 2004).
Moreover, decreases in glucose metabolism in ventral regions of the PFC (Brody et al. 1999; Kennedy et al. 2001) and increases in the temporal cortex (Buchsbaum et al. 1997; Brody et al. 2001) have been previously associated with the response to selective serotonin reuptake inhibitors (SSRIs). Pre- vs. posttreatment changes in the ventrolateral prefrontal and temporal cortex, posterior cingulate (BA 29), and putamen have also been reported with non-SSRI antidepressant pharmacotherapy (Davies et al. 2003; Martin et al. 2001). Furthermore, the caudate nucleus is discussed to be a trait marker of depression vulnerability, and caudate activation is elevated even in recovered depressed patients (Norbury et al. 2010). Before treatment, patients with MDD responding better to pharmacological treatment showed greater activation in the dorsomedial PFC (dmPFC), posterior cingulate cortex (pCC), and superior frontal gyrus (SFG) when viewing of negative emotional pictures compared with the resting condition. Activations in the caudate nucleus and insula contrasted for emotional compared to neutral stimuli were also associated with successful treatment (Samson et al. 2011). In a meta-analysis a difference in the activation of a limbic–cortical path of the hippocampus–OFC–ACC–lateral PFC network was found between responders and nonresponders to paroxetine and a combination of various medications. The pathway OFC–ACC–lateral PFC was also characteristic for responders treated with various antidepressants (Seminowicz et al. 2004). Indeed good OFC connectivity observed before treatment was shown to be associated with response to antidepressant (Lisiecka et al. 2011).
Responders may, therefore, be more likely to exhibit compensatory functional hyperactivation during fMRI tasks, and thus, responders might be likely to be identified by functional MRI. However, this link and the underlying neurochemical changes of functional hyperactivation require further exploration.
14.7 Conclusion
Improved MRI techniques and fMRI tasks that make possible the study of altered brain function in depression will likely be able to provide useful information on therapy effects and may, in the future, be able to predict therapy response. More longitudinal research during the disease course is needed to achieve these aims.
References
Ahdidan J, Hviid LB, Chakravarty MM, Ravnkilde B, Rosenberg R, Rodell A, Stodkilde-Jorgensen H, Videbech P (2011) Longitudinal MR study of brain structure and hippocampus volume in major depressive disorder. Acta Psychiatr Scand 123(3):211–219PubMed
Anand A, Li Y, Wang Y, Wu J, Gao S, Bukhari L, Mathews VP, Kalnin A, Lowe MJ (2005) Activity and connectivity of brain mood regulating circuit in depression: a functional magnetic resonance study. Biol Psychiatry 57(10):1079–1088PubMed
Arce E, Simmons AN, Lovero KL, Stein MB, Paulus MP (2008) Escitalopram effects on insula and amygdala BOLD activation during emotional processing. Psychopharmacology (Berl) 196(4):661–672
Baare WF, Vinberg M, Knudsen GM, Paulson OB, Langkilde AR, Jernigan TL, Kessing LV (2010) Hippocampal volume changes in healthy subjects at risk of unipolar depression. J Psychiatr Res 44(10):655–662PubMed
Bartley AJ, Jones DW, Weinberger DR (1997) Genetic variability of human brain size and cortical gyral patterns. Brain 120(Pt 2):257–269PubMed
Bermingham R, Carballedo A, Lisiecka D, Fagan A, Morris D, Fahey C, Donohoe G, Meaney J, Gill M, Frodl T (2012) Effect of genetic variant in BICC1 on functional and structural brain changes in depression. Neuropsychopharmacology 37(13):2855–2862PubMedCentralPubMed
Bremner JD, Vermetten E (2004) Neuroanatomical changes associated with pharmacotherapy in posttraumatic stress disorder. Ann N Y Acad Sci 1032:154–157PubMed
Brody AL, Saxena S, Silverman DH, Alborzian S, Fairbanks LA, Phelps ME, Huang SC, Wu HM, Maidment K, Baxter LR Jr (1999) Brain metabolic changes in major depressive disorder from pre- to post-treatment with paroxetine. Psychiatry Res 91(3):127–139PubMed
Brody AL, Saxena S, Mandelkern MA, Fairbanks LA, Ho ML, Baxter LR (2001) Brain metabolic changes associated with symptom factor improvement in major depressive disorder. Biol Psychiatry 50(3):171–178PubMed
Buchsbaum MS, Wu J, Siegel BV, Hackett E, Trenary M, Abel L, Reynolds C (1997) Effect of sertraline on regional metabolic rate in patients with affective disorder. Biol Psychiatry 41(1):15–22PubMed
Bueller JA, Aftab M, Sen S, Gomez-Hassan D, Burmeister M, Zubieta JK (2006) BDNF Val66Met allele is associated with reduced hippocampal volume in healthy subjects. Biol Psychiatry 59(9):812–815PubMed
Campbell S, Marriott M, Nahmias C, MacQueen GM (2004) Lower hippocampal volume in patients suffering from depression: a meta-analysis. Am J Psychiatry 161(4):598–607PubMed
Canli T, Qiu M, Omura K, Congdon E, Haas BW, Amin Z, Herrmann MJ, Constable RT, Lesch KP (2006) Neural correlates of epigenesis. Proc Natl Acad Sci U S A 103(43):16033–16038PubMedCentralPubMed
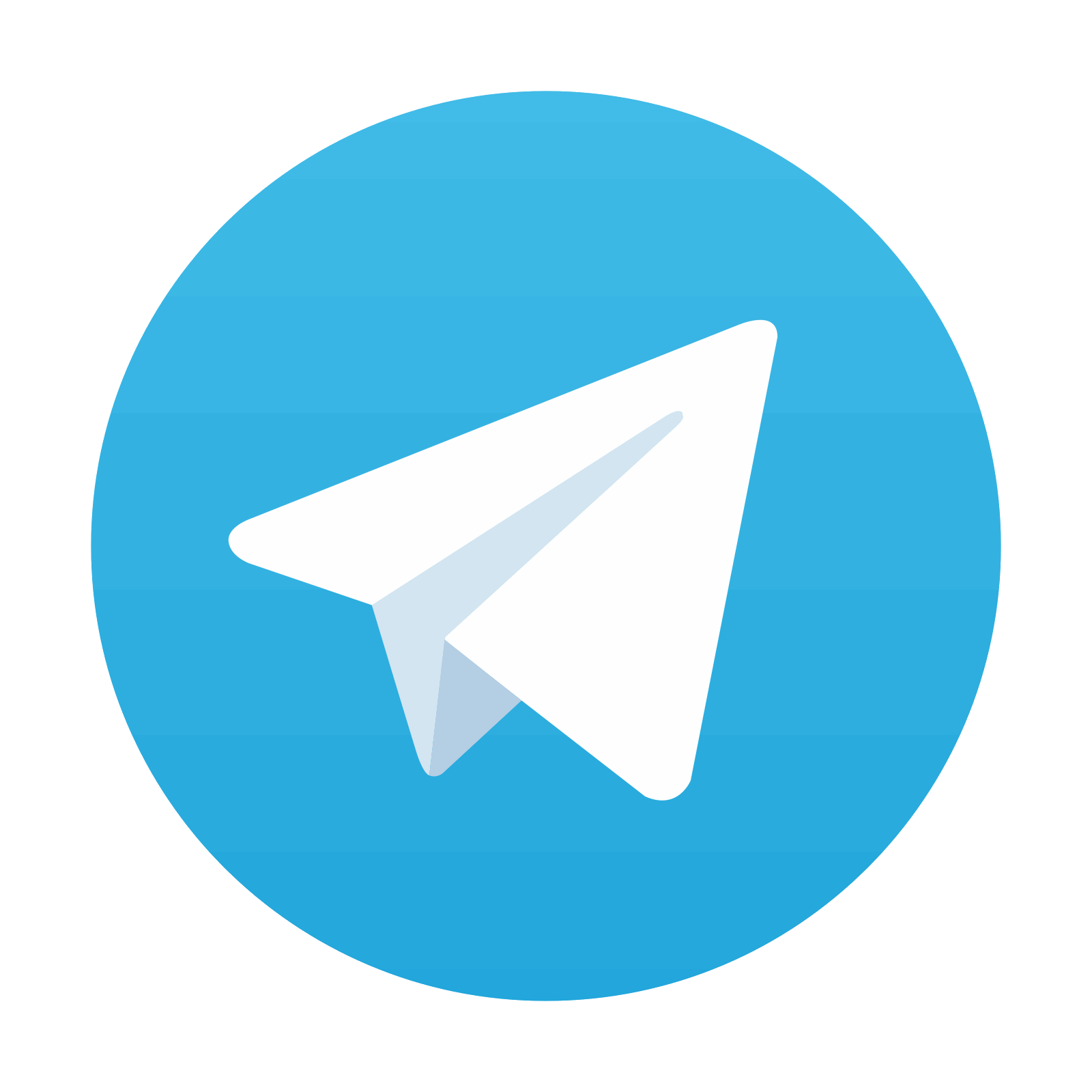
Stay updated, free articles. Join our Telegram channel

Full access? Get Clinical Tree
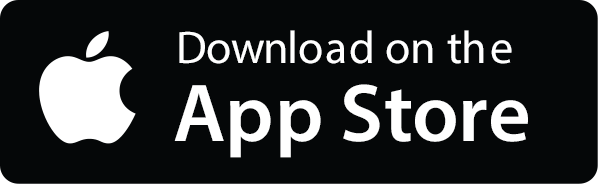
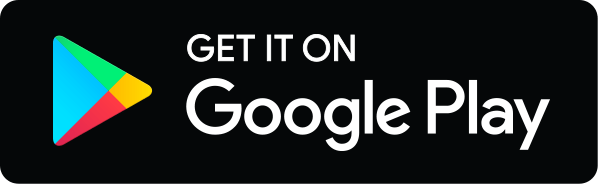