Neoplastic Disease of the Spine and Spinal Cord
Puneet S. Pawha
Gerard M. Reddy
Gordon Sze
Of all areas of spinal pathology, it may be in the field of spinal tumors that magnetic resonance imaging (MRI) has had the most impact. Almost immediately after its inception, even with the poor quality of early scans, the potential of MR in the evaluation of suspected neoplasms of the cord was recognized (1). Today, MR is always considered the essential procedure of choice for the workup of all spinal tumors (2), in the absence of contraindications to its use.
Spinal tumors should virtually always initially be assessed by the radiologist asking “within what compartment is the lesion situated?” that is, is it extradural, intradural, extramedullary, or intramedullary. This classification scheme has stood the test of time, over decades of radiologic diagnosis of spinal mass lesions, as well as over the entirety of the dramatic evolution of imaging methodology up to and including the current state-of-the art MRI techniques. The key to answering this essential question in the diagnostic approach to spinal lesions lies in inspection of the edge of the lesion at its interface with the subarachnoid space. In extradural lesions, the subarachnoid space is at the interface of the mass lesion and the spinal cord (Fig. 21.1). In intradural extramedullary masses, the subarachnoid space is widened and caps the lesion (Fig. 21.2). In intramedullary masses, the lesion is part of the expanded spinal cord; hence, the subarachnoid space around the lesion–spinal cord complex is narrowed (Fig. 21.3).
That said, there are cases in which the categorization of mass lesions into a single compartment either cannot be accomplished or is less helpful than in most cases. First, a given lesion may reside in two compartments simultaneously. The most common diagnosis in the scenario of a lesion occupying two compartments is a neurofibroma extending into both the extradural and the intradural extramedullary spaces (Fig. 21.4). Second, two lesions with identical pathology may occur in different compartments. For example, metastases obviously may occur in any of the three compartments, including the intramedullary space. Third, a lesion that is plaquelike, instead of spherical, will not necessarily demonstrate the above-described effects on the appearance of the cerebrospinal fluid (CSF) column.
The typical example would be a plaquelike meningioma that, although an intradural extramedullary lesion, would not necessarily widen the CSF space. Nevertheless, this classification scheme serves well as the basis for localization and ultimately the appropriate differential diagnosis of spinal tumors.
The typical example would be a plaquelike meningioma that, although an intradural extramedullary lesion, would not necessarily widen the CSF space. Nevertheless, this classification scheme serves well as the basis for localization and ultimately the appropriate differential diagnosis of spinal tumors.
In the extradural space, numerous primary bone tumors can occur. However, with few exceptions, such as hemangioma, most of the primary bone tumors are comparatively unusual. Secondary tumors, including metastases, are far more common in the extradural space.
In the intradural extramedullary space, primary tumors, such as neurofibroma and meningioma, are relatively common. Secondary tumors or leptomeningeal metastases were formerly considered quite rare. This entity is now seen more frequently because of several factors. First, clinicians have a higher awareness of leptomeningeal tumor and greater suspicion of it under the appropriate clinical circumstances. Second, laboratory tests are more precise in identifying tumor cells in the CSF. Third, as patients live longer with their tumors, they have more opportunity to develop ancillary complications of their disease, such as leptomeningeal tumor. Therefore, the increase seen in leptomeningeal tumor is due to both better diagnosis and an actual increase in its incidence.
Finally, in the intramedullary space, primary tumors are far more common than secondary tumors or metastases. Metastases to the cord itself are comparatively unusual.
Extradural Tumors
Technique
When evaluating spinal tumors involving the epidural space with MR, it is important to keep two goals in mind: (a) the detection of vertebral body lesions, even if there is no suspicion of epidural impingement; and (b) the delineation of possible nerve root or spinal cord compression.
Unenhanced MR scans are generally superb at delineating extradural tumors, whether they are primary or secondary (1,2,3). Vertebral body lesions are usually well visualized as low-intensity lesions surrounded by the higher intensity of normal fat-containing marrow on T1-weighted images. Before the development of MR, bone scans were considered the most sensitive means of detecting suspected tumors of the vertebral body. MR, however, has been found to be more sensitive to marrow abnormalities (2). For example, active lesions that might not be visible on a bone scan are generally detectable on MR (2).
![]() FIGURE 21.4 Neurofibromas occupying multiple compartments in sagittal T2 (A, B) and axial postcontrast images (C). |
The standard MR parameters for the detection of vertebral body lesions generally consist of short–repetition time (TR)/short–echo time (TE) (T1-weighted) spin-echo (SE) sequences (2). These acquisitions are very sensitive to the high intensity of normal fat-containing marrow and show morphology in great detail. Tumors usually are of low signal intensity on T1-weighted sequences and of high signal intensity on long–TR/TE (T2-weighted) sequences. Normal marrow, in contrast, generally is of high signal intensity on T1-weighted scans and of less signal intensity on T2-weighted scans (Fig. 21.5). Fast spin-echo (FSE)-type sequences must be used with caution because the persistent high signal of surrounding fat on T2-weighted images may decrease visualization of a tumor (Fig. 21.5). Fat-suppressed T2-weighted imaging, on the other hand, is often useful in depicting marrow lesions.
In occasional cases, uninvolved marrow appears to be low in signal, especially in young patients, in whom the vertebral body marrow does not contain much fat or in patients with anemia of chronic disease, who are hypothesized to have altered metabolism of iron. In these cases, the low-intensity lesions are not highlighted by the surrounding high-intensity marrow, and it may be difficult to detect tumor on unenhanced T1-weighted images. In these instances, T2-weighted sequences, short–inversion-time inversion recovery (STIR) sequences, or gradient-echo (GRE) sequences may help for further evaluation.
Impingement on the thecal sac can occur by extension of a vertebral body tumor or by neoplastic growth in the epidural space itself. Good-quality MR scans of the spine are generally very precise in showing impingement on the thecal sac. T1- and T2-weighted sequences are both excellent for depiction of epidural disease, particularly when lesions are visualized in two orthogonal planes (Fig. 21.6). However, in some cases, particularly when disease is extensive, T2 GRE or balanced steady-state free precession acquisitions can also help. By producing a myelographic effect with the CSF of high signal, they can better delineate regions of impingement.
MR can also be useful in patients with compression fractures of the vertebral bodies to differentiate between benign osteoporotic collapse and pathologic collapse due to neoplastic replacement. In the chronic situation, nonneoplastic involvement is typified by preservation of normal bone marrow signal, absence of edema, and no associated paravertebral soft tissue. Tumor causing compression fractures are usually lower signal intensity on T1-weighted images (Figs. 21.5 and 21.7). Unfortunately, in more acute cases, the distinction between the two causes is more difficult, in that both may display altered signal because of marrow edema in nonneoplastic causes (Fig. 21.8) and because of tumor with edema in neoplasms. Benign acute vertebral compression fractures can also show paravertebral edema and hemorrhage, which further complicates the imaging
distinction from metastatic tumor. In some cases of acute benign osteoporotic collapse, incomplete signal loss is seen. An even band of normal high-signal marrow with smooth margins may remain adjacent to a band of lower signal in the same vertebral body. This appearance is suggestive of a nonneoplastic deformity (Fig. 21.7). Linear fluid signal adjacent to the fractured endplate is suggestive of a benign fracture, although it does not exclude a neoplastic process. In a series of 87 patients, this finding was present in 21 of 52 benign fractures (40%) but only in 2 of 35 neoplastic fractures (6%) (4).
distinction from metastatic tumor. In some cases of acute benign osteoporotic collapse, incomplete signal loss is seen. An even band of normal high-signal marrow with smooth margins may remain adjacent to a band of lower signal in the same vertebral body. This appearance is suggestive of a nonneoplastic deformity (Fig. 21.7). Linear fluid signal adjacent to the fractured endplate is suggestive of a benign fracture, although it does not exclude a neoplastic process. In a series of 87 patients, this finding was present in 21 of 52 benign fractures (40%) but only in 2 of 35 neoplastic fractures (6%) (4).
There has been considerable debate about the use of diffusion-weighted imaging (DWI) to differentiate between benign and pathologic compression fractures. A number of studies have been published using a variety of techniques, including steady-state free procession, line scan imaging, and quantification of apparent diffusion coefficient values (5,6). Because the results have been mixed and some false positives have been demonstrated, the utility of DWI in making this important distinction has been controversial (7). A few studies have, however, shown that the use of apparent diffusion coefficient (ADC) values can aid in making this differentiation, reducing the incidence of false positives (6,8).
When gadolinium compounds are given for spinal lesions, enhancement is extremely variable (3). Some tumors enhance mildly and remain hypointense to normal marrow; others enhance markedly and become hyperintense; still others enhance moderately and become isointense to normal marrow. This variability of enhancement often occurs even in different metastatic lesions within the same patient.
Because low-intensity lesions tend to enhance after the administration of gadolinium, they often become isointense with surrounding marrow and are less easily detectable (3,9). In fact, gadolinium can even obscure some lesions. If the detection of vertebral body lesions is a consideration, a noncontrast scan is often sufficient. If postcontrast imaging is performed for vertebral column lesions, it should be combined with fat suppression. One study has shown fat-saturated T1-weighted fluid-attenuated inversion recovery (FLAIR) imaging to increase conspicuity of extradural enhancing lesions when compared with fat-saturated T1-weighted FSE imaging (10).
Although not usually essential in the detection of vertebral lesions, gadolinium contrast agents can be particularly useful as an adjunct when epidural tumors are considered (3,9). Contrast may be helpful in (a) more specifically characterizing possible epidural tumor, (b) indicating regions of more active tumor for biopsy, and (c) outlining areas of cord compression, when necessary. In specific cases, it also may be helpful in (a) differentiating diffuse marrow involvement with tumor from marrow that is abnormal in signal for nonneoplastic reasons, and (b) in evaluating response to therapy.
Contrast can further help to characterize suspected epidural tumors (3,9). For example, although disc herniation usually is easily differentiated from epidural tumors on noncontrast MR scans, occasionally an epidural mass is seen adjacent to a narrowed disc. In these cases, the etiology of the mass may be in question. Gadolinium enhancement may prove helpful in this specific clinical situation. Although discs and disc fragments generally do not enhance on immediate postcontrast acquisitions, tumor does. Therefore, these cases may benefit from the administration of gadolinium because enhancing tumor can be differentiated from nonenhancing disc material.
Gadolinium-enhanced imaging can help to highlight areas of cord compression (3). Occasionally, especially in patients who have diffuse metastatic disease and congenitally narrow spinal canals, exact localization of areas of cord compression may be difficult on unenhanced T1-weighted MR scans. This is particularly true in the cervical and thoracic regions, where a paucity of fat in the spinal canal prevents outlining of epidural lesions. Because of the extent of the vertebral body involvement, placement of the axial scans also is difficult. After the administration of gadolinium, enhancing tumor surrounding nonenhancing cord is better delineated. Alternatively, high-quality, T2-weighted scans or GRE acquisitions can provide similar information. Finally, for patients in whom spinal cord compression is suspected, it should be remembered that intradural or even intramedullary lesions can mimic extradural compressive lesions; therefore, it is also of use to perform postcontrast imaging in those cases in which no compressive lesion is found.
Primary Extradural Tumors
Vertebral Hemangioma
Vertebral hemangiomas are benign vascular tumors in the spinal column, present in approximately 11% of all patients. In autopsies of 3,829 spines, Schmorl and Junghanns found hemangiomas in 409. Vertebral hemangiomas tend to increase in incidence with age. These lesions are solitary in 66% and multiple in 34% of cases. Sixty percent occur in the thoracic region, 29% in the lumbar region, 6% in the cervical region, and 5% in the sacrum. They are slightly more common in women.
The vast majority of vertebral hemangiomas are discovered incidentally. Rarely, they may be symptomatic. Symptomatic lesions tend to occur in the thoracic region. In one study, 13 of 14 cases (93%) of symptomatic vertebral hemangiomas were located in the thoracic region, specifically between T3 and T9 (11).
Initially, symptoms include localized pain and tenderness, often associated with muscle spasm. Radiculopathy may result from impingement on a nerve root. Myelopathic symptoms, such as motor and/or sensory abnormalities, can be seen with cord compression. Cord compression may occur secondary to (a) mechanical compression of the spinal cord by the expansion of the tumor within the vertebral body and/or posterior elements, (b) extension of tumor into the epidural space, (c) epidural hematoma secondary to the hemangioma, and (d) rarely, compression fracture of the involved vertebral body. Compression fractures in hemangiomas are unusual because the involved vertebrae usually have thickened vertical trabeculae, which tend to protect against axial collapse.
Pathology
Grossly, these lesions are characterized by their dark red color (11). Histologically, they consist of vascular structures within bony sinuses lined by endothelium and filled with blood. This angiomatoid tumor can destroy some bony trabeculae, resulting in compensatory thickening of the remaining vertical trabeculae.
Imaging
The thickened vertical trabeculae of hemangiomas cause parallel linear densities producing a “jail bar” or “corduroy cloth” appearance in the vertebral body on plain films. This appearance can also be seen on sagitally or coronally reformatted computed tomography (CT), and even sometimes on sagittal MR images. Extension into the posterior elements can occur. On axial CT, the remaining thickened trabeculae give a typical spotted appearance to the vertebral bodies. This appearance can also sometimes be appreciated on axial MR images, particularly on T2-weighted sequence (Fig. 21.9).
MR is extremely sensitive in the detection of hemangiomas. On both T1-weighted and T2-weighted images, these lesions tend to have increased signal intensity (Fig. 21.9). This high signal largely reflects the adipose tissue in these lesions rather than a hemorrhagic component. Occasionally, hemangiomas within the bony confines can have a paucity of adipose tissue and even appear diffusely hypointense making their identification more difficult. These lesions may be more aggressive and can enhance markedly with contrast.
MR is able to show paravertebral and epidural extension of tumor. Extraosseous components tend to lack adipose tissue
and to appear isointense on T1-weighted images. MR readily defines spinal cord and/or thecal sac compression or displacement. In those occasional cases in which multiple vertebral hemangiomas are present and lack the typical T1 hyperintense signal, it may be impossible to distinguish these benign lesions from metastases without the benefit of other clinical information or other imaging studies.
and to appear isointense on T1-weighted images. MR readily defines spinal cord and/or thecal sac compression or displacement. In those occasional cases in which multiple vertebral hemangiomas are present and lack the typical T1 hyperintense signal, it may be impossible to distinguish these benign lesions from metastases without the benefit of other clinical information or other imaging studies.
Treatment
Asymptomatic lesions are left untreated. Symptomatic lesions can be treated with surgical decompressive laminectomy. Preoperative angiography can be useful to identify and occlude feeding arteries. Radiation therapy may also be used, either preoperatively or by itself. Percutaneous therapeutic techniques have also been described, including direct injection of ethanol into the lesion.
Osteochondroma
Although osteochondromas are the most common benign bone tumors (35.8%), only 3% of solitary and 7% of multiple osteochondromas occur within the spine (12). Nevertheless, because osteochondromas are much more common than many other bone tumors, they actually are encountered in the spine with regularity. Osteochondromas of the vertebral column are nearly always confined to the posterior elements, with a predilection for the spinous processes. Involvement of the vertebral body is unusual but has been reported (12). Most cases occur in the thoracic or lumbar region; the cervical spine is only rarely the primary site (12,13).
Three-fourths of osteochondromas occur in patients younger than 20 years. There is a slight male predilection. Any bone preformed in cartilage can give rise to this lesion. These lesions occur in two different patterns—solitary and multiple. Solitary lesions have no known genetic component, whereas multiple lesions are seen in multiple hereditary exostosis.
Signs and symptoms of osteochondromas are nonspecific. Frequently, pain is present; there may or may not be associated swelling or a palpable soft tissue mass. The lesions are usually large by the time they are symptomatic and come to medical attention (14).
Neurologic symptoms are rare, occurring in less than 0.1% of patients. There is, however, a greater incidence of spinal cord symptoms in the teenage years, implying that growth spurts of the osteochondroma could compromise a marginally narrowed canal. Thoracolumbar lesions can present with bowel or bladder dysfunction and lower-extremity weakness, but cervical lesions have a varied presentation (12,13). In addition to myelopathy, the presence of a mass may be noted. Dysphagia and even sudden death from partial transection of the cervical cord from an osteochondroma of the dens have been reported. If lesions bridge multiple vertebrae, fusion and restricted motion can result, most evident in the cervical region.
Pathology
Imaging
Plain films show a pedunculated or sessile lesion with its cortex in direct contiguity with the cortex of the adjacent bone. These lesions generally originate from the posterior elements.
CT accurately delineates the exact site of attachment of the lesion to the adjacent bone, the presence of the cartilaginous cap, and any compromise of the spinal canal (14). In addition, CT can be helpful in distinguishing between benign osteochondromas and malignant degeneration into an osteosarcoma or chondrosarcoma. The incidence of malignant degeneration is 1% in cases of solitary osteochondroma and 5% to 25% in cases of multiple hereditary exostoses; rapid growth of the tumor should prompt suspicion of malignancy.
Osteochondromas have a heterogeneous appearance on MR. The cartilaginous portions of this lesion are of increased signal intensity on the T2-weighted images, whereas the osteoid or
calcified portions demonstrate low signal intensity. Both MRI and CT can effectively depict the “corticomedullary continuity” with the parent bone, that is characteristic of this tumor (Fig. 21.10). Because a large portion of the tumor is bony, the extent of thecal sac impingement may be easier to delineate on T2-weighted or GRE sequences rather than T1-weighted sequences, in which the low-intensity regions may blend into CSF. As with other imaging modalities, the rapid growth of the lesion is an ominous sign. Factors favoring benignity include cortical margins that are contiguous with the adjacent bone, well-defined lobular surfaces, lack of adjacent bone involvement, and a thin cartilaginous cap (usually less than 1 cm).
calcified portions demonstrate low signal intensity. Both MRI and CT can effectively depict the “corticomedullary continuity” with the parent bone, that is characteristic of this tumor (Fig. 21.10). Because a large portion of the tumor is bony, the extent of thecal sac impingement may be easier to delineate on T2-weighted or GRE sequences rather than T1-weighted sequences, in which the low-intensity regions may blend into CSF. As with other imaging modalities, the rapid growth of the lesion is an ominous sign. Factors favoring benignity include cortical margins that are contiguous with the adjacent bone, well-defined lobular surfaces, lack of adjacent bone involvement, and a thin cartilaginous cap (usually less than 1 cm).
Treatment
Because these lesions are benign, no treatment is required unless they are large and compress adjacent structures. In this instance, surgery can be performed and is usually curative, with only a 5% recurrence rate. Obviously, tumors with malignant degeneration require additional therapy.
Osteoid Osteoma
Osteoid osteoma comprises 11% to 12% of all benign bone tumors (15). It has been reported in virtually every bone, with a 0% to 25% incidence in the spine (average, approximately 10%) (16). The most common locations in the spine are the lumbar region (59%), followed by the cervical (27%), thoracic (12%), and sacral (2%) regions (16). The posterior elements are involved in 75% of cases. Thirty-three percent of cases involve the laminae, 19% affect the articular facets, and 15% affect the pedicles (16). The vertebral body is affected in only 7% (16).
Osteoid osteomas are more common in males than females by a 2:1 to 4:1 ratio. This tumor is rare after age 30 years; 87% of the cases occur before this age. In MacLellan and Wilson’s review of the literature in 1967, of 36 documented cases of spinal osteoid osteoma, the average age was 16.7 years, and 72% of the patients were between 10 and 25 years old.
Localized pain at the site of the lesion is highly characteristic; only rarely are they asymptomatic (1.6%) (13). Classically, the pain is worse at night and relieved by aspirin. Although it may be intermittent at first, it soon becomes constant and severe. Radicular pain can occur if the lesion encroaches on the neural foramina, which is seen in 50% of patients.
There can be a significant delay in evaluation and diagnosis until the symptoms increase in severity. The average delay from initial symptoms to diagnosis in the series of Jackson et al. (17) was 11.3 months. In the absence of trauma, back pain is very unusual in a child and should prompt evaluation.
Focal tenderness is the most common sign of osteoid osteoma and is seen in 69% of patients (16). Scoliosis is also frequently seen resulting from muscle spasm and consequent pelvic tilt. Scoliosis was seen in 29 of 36 cases in one literature review.
Pathology
Grossly, this tumor has a central, vascular nidus that is reddish gray. Histologically, the tumor contains multinucleated giant cells. The nidus consists of very vascular fibrous connective tissue with surrounding osteoid matrix,
and may contain irregular calcification (Fig. 21.11). The size of the nidus is by definition less than 1.5 cm (if greater than 1.5 cm, the lesion would be classified as an osteoblastoma), with an average size of 0.9 cm. The nidus is surrounded by sclerotic bony reaction. The extent of sclerosis is extremely variable but tends to be less in spinal lesions.
and may contain irregular calcification (Fig. 21.11). The size of the nidus is by definition less than 1.5 cm (if greater than 1.5 cm, the lesion would be classified as an osteoblastoma), with an average size of 0.9 cm. The nidus is surrounded by sclerotic bony reaction. The extent of sclerosis is extremely variable but tends to be less in spinal lesions.
Imaging
The appearance of osteoid osteoma in the spine resembles its appearance elsewhere in the skeleton. Plain films, which demonstrate the classic findings in 66% to 75% of cases, show a lucent nidus (Fig. 21.12). Frequently, a small amount of calcification can be seen within the nidus. Surrounding bony sclerosis can be seen but its extent is variable. If there is extensive bony sclerosis, the exact location of the nidus can be difficult to discern on plain films, and further imaging is required to localize the nidus.
If the initial plain films are negative and clinical suspicion is still high for an osteoid osteoma, nuclear medicine bone scans are generally recommended. Osteoid osteomas are focally “hot” on bone scan (Fig. 21.12). Once the level of the lesion is localized with the bone scan, then further cross-sectional imaging with either CT or MR can be performed to confirm the precise location of the nidus preoperatively.
Frequently, the nidus can be seen only on cross-sectional imaging. CT shows a small, rounded area of low attenuation with or without calcification (Figs. 21.12 and 21.13). Surrounding sclerosis is evident and can be extensive.
On MR, osteoid osteomas have a heterogeneous appearance. The calcification within the nidus and the surrounding bony sclerosis are of low signal intensity on T1-weighted and T2-weighted images. The noncalcified portions of the nidus itself demonstrate increased signal intensity on the T2-weighted images. The administration of gadolinium, like that of iodinated contrast material, causes intense enhancement within the highly vascular nidus. This enhancement may help not only to localize the nidus, but also to differentiate it from a nonenhancing lytic lesion such as Brodie’s abscess, which can also demonstrate similar surrounding edema and sclerosis. MR also may show an associated reactive soft-tissue mass. This soft-tissue swelling usually demonstrates inhomogeneous signal on T1-weighted images and increased signal on T2-weighted images (Fig. 21.12). Adjacent bone marrow may also demonstrate these signal changes. Both the soft tissue and the reactive marrow often enhance with contrast. Although CT has traditionally been the primary modality for evaluation of suspected osteoid osteomas due to its sensitivity to bone detail, MR is being used with increasing frequency. In one case report, MR was able to localize the nidus of a lesion that could not be defined on any other modality. Dynamic postcontrast MRI of osteoid osteomas was described with good results in a series of 11 patients. The nidus demonstrated arterial phase peak enhancement in 9 of 11 (82%) patients and was equally or more conspicuous compared with thin-section CT in all cases (18).
Treatment
Osteoid osteomas can be conservatively treated with medication (nonsteroidal anti-inflammatory drugs) because some may regress spontaneously after several years. Definitive treatment requires complete removal of the nidus. Traditionally, this meant surgical excision; however, percutaneous ablation (radiofrequency, laser, and other techniques) has emerged as a primary modality of treatment in both appendicular and axial skeletal locations. This treatment option has demonstrated good outcomes, with the benefit of decreased hospitalization and recovery time.
Osteoblastoma
Osteoblastomas are uncommon, benign bone tumors accounting for 1% of all primary bone neoplasms (19). Although they have been described in almost every bone, there is a particular predilection for the spine, which accounts for 25% to 50% of
cases (19). In the Mayo Clinic series, 39 of the 123 tumors were located in the spine. The lumbar spine is most often involved, followed by the thoracic and cervical spine (19). Osteoblastomas most often occur in the posterior elements. In 1988, Myles and MacRae reported 10 cases of spinal osteoblastoma in children and found that 9 were located in the posterior elements (19). In 14% of cases, the lesions were located in the vertebral body (19). In 24% of cases, both the vertebral body and posterior elements were involved. Epidural extension of tumor could be seen (19).
cases (19). In the Mayo Clinic series, 39 of the 123 tumors were located in the spine. The lumbar spine is most often involved, followed by the thoracic and cervical spine (19). Osteoblastomas most often occur in the posterior elements. In 1988, Myles and MacRae reported 10 cases of spinal osteoblastoma in children and found that 9 were located in the posterior elements (19). In 14% of cases, the lesions were located in the vertebral body (19). In 24% of cases, both the vertebral body and posterior elements were involved. Epidural extension of tumor could be seen (19).
![]() FIGURE 21.13 Osteoid osteoma. Axial (A) and parasagittal (B) computed tomography images demonstrate a small lucent nidus (arrowhead) in the left lamina with surrounding bony sclerosis. |
Osteoblastomas are more common in men (19,20). In the Mayo Clinic series, 87 of 123 cases were found in men. Ninety percent of the cases occur before age 30 years. The lesion usually presents in patients within the second or third decade of life (19,20).
Osteoblastomas most often present with pain and local tenderness (19). Scoliosis or torticollis may result. The symptoms may occur before the lesion becomes evident on plain films. Frequently there is a delay in diagnosis (average 9.3 to 12.3 months) (19,20).
Pathology
Grossly, these masses are soft, hemorrhagic, and very vascular and friable. Because of mineralized osteoid, they often have a granular texture. They also contain fibrovascular stroma (19,20).
Histologically, osteoblastomas appear similar to both osteoid osteoma and osteosarcoma. Osteoid osteoma and osteoblastoma are differentiated by their size. Osteoblastomas are larger than 1.5 cm, whereas osteoid osteomas are smaller than 1.5 cm. The average size of the nidus in one study was 2.4 cm. In another study, the size ranged up to 10 cm. In addition, osteoblastomas may lack the identifiable central nidus and have less surrounding sclerosis than osteoid osteoma.
Imaging
On plain films and CT, these lesions tend to be expansile, with surrounding thinned cortex. In the spine, the lesions usually involve the posterior elements (Fig. 21.14) (19,20). The tumor may have a lucent or an ossified center (20). The margins are often but not always well defined. There can be dense sclerotic reaction associated with these lesions (20,21). CT also shows associated soft tissue masses and epidural extension (19).
MR readily shows the lesion and any associated soft tissue mass. Impingement on the thecal sac can be detected. These lesions are inhomogeneous if areas of hemorrhage or calcification are present (Fig. 21.14). A thin rim of signal void as a result of the bony shell may be visible. On T2-weighted images, osteoblastomas demonstrate high signal intensity, possibly corresponding to regions of vascularized stroma. Irregular linear areas of signal void may be seen, corresponding to osseous trabeculae (Fig. 21.11). Osteoblastomas demonstrate enhancement after administration of contrast on T1-weighted images (Fig. 21.14).
Treatment
The goal of treatment is total excision of the lesion (19). If the lesion is removed completely, there is usually complete disappearance of symptoms with relatively little chance for recurrence, although recurrence is more common in lesions involving the spine (19,20). Preoperative embolization is often used to decrease intraoperative bleeding in hypervascular tumors. Long-term follow-up is essential because recurrences may occur as long as 9 years after surgery. Radiation therapy may be given for incompletely removed recurrent lesions (19). Rarely, malignant degeneration has been reported.
Aneurysmal Bone Cyst
Aneurysmal bone cysts (ABCs) are benign disorders of bone with an unknown etiology. These tumors represent 1.4% to 2.3% of primary bone neoplasms (15). Generally, these lesions arise de novo; however, they can be associated with other lesions (32%), such as giant cell tumor, chondroblastoma, chondromyxoid fibroma, fibrous dysplasia, and nonossifying fibroma. There is either a slight female predilection or no sex predilection. The patients are usually in their first two decades, with 66% to 78% of the cases occurring in patients less than 20 years of age (15). In a study reviewing 81 cases of ABC in the spine, the average age was 16.6 years, and most occurred between 10 and 25 years of age (22). Giant cell tumors, which may appear similar, are usually seen in patients older than 30 years of age (23).
Although these lesions have been found in almost every bone, the spine is involved frequently. In the Mayo Clinic series of 134 cases of ABCs, 27 were located in the spine, including 5 in the sacrum. In other studies, between 3% and 20% of cases involve the spine. The neural arch is the most frequently affected site. Sixty percent of ABCs involve the posterior elements; 40% arise in the vertebral bodies (21). Forty-four percent occur in the lumbosacral region, 34% in the thoracic spine, and 22% in the cervical spine. They can cross the intervertebral disc space and involve an adjacent vertebral body. About 22% of lesions extend into the paraspinal soft tissues. There may or may not be an associated soft tissue mass.
Symptomatology varies tremendously based on the size and degree of differentiation of the lesion. A small lesion can be entirely asymptomatic. When symptoms are present, they usually consist of localized pain and/or swelling. Large lesions can impinge on the spinal cord with resultant long-tract symptoms and signs. In one study of 15 patients, compression fractures contributed to symptoms in four cases. In addition, neural foraminal narrowing can result in radiculopathy. Often the symptoms may be longstanding before diagnosis. The average duration of symptoms in one series was 8 months.
Pathology
Grossly, ABCs are clearly delineated by the eggshell-thin cyst of subperiosteal new bone. The interior of the lesion can be solid and vascular or cystic and hemorrhagic. ABCs are composed of large, anastomosing cavernous spaces filled with unclotted blood (23). The linings of these spaces lack normal features of blood vessels and do not contain endothelium, muscle fibers, or elastic laminae (22,24). This benign lesion also has solid portions, which are frequently composed of osteoid material, sometimes intermixed with fibrous tissue (22). Histologically, these lesions can be mistaken for other entities, such as telangiectatic osteosarcoma. Giant cells are present within the trabeculae of this lesion and can lead to confusion with giant cell tumor (22).
Imaging
Plain films of ABCs of the spine show an expansile lytic lesion usually involving the posterior elements (24). An eggshell-thin cortical margin is often seen. Severe lesions can destroy the vertebral body and result in collapse and vertebra plana (22). CT confirms the expansile appearance of the lesion and better defines any soft tissue extension (23,24). The absence of permeative bone destruction decreases the possibility of more aggressive processes. In addition, multiple small fluid levels sometimes can be seen on CT. Frequently, to visualize these fluid levels best, the patient must remain motionless for 10 minutes before scanning to allow the different components of blood to settle out within the cavernous spaces of the tumor (24).
MR exhibits similar findings to those seen on CT. Expansile lesions are noted, often with internal septations and lobulations (Fig. 21.15). A thin, well-defined rim of low signal intensity is often visualized on both short and T2-weighted images. Multiple small fluid–fluid levels and internal septations may be present (24). The fluid can have varying signal intensity based on the presence of intracystic hemorrhage of different ages (24). These vary from high to low signal on short and T2-weighted images. In other cases, the lesions may exhibit uniformly high intensity on T2-weighted images. Paravertebral extension of the mass is well demonstrated on MR. Epidural extension or spinal cord compression is better depicted on MR than on CT. After the administration of contrast, the septations within the lesion generally enhance (25).
Altogether, the MR appearance of an ABC is characterized by involvement of the posterior elements of the spine, a rim of low signal intensity, and multiple fluid levels.
Treatment
Curettage is the initial treatment employed. Recurrence develops in 10% to 20% of patients (15). If the lesion recurs several times, radiation therapy may be used.
Giant Cell Tumor
Giant cell tumors constitute approximately 4% to 5% of all primary bone tumors and 21% of the benign tumors (15). These lesions are most common in adults. There is no sex predilection.
Although these tumors occur most often at the knee, giant cell tumors are not infrequently seen in the spine. In the Mayo Clinic series of 2276 bone tumors, they were the most common benign spinal column tumors, excluding hemangiomas (15). This lesion is the most frequent benign tumor to involve the sacrum (11 of 209 cases) (26). Giant cell tumors less often involve the rest of the spine (2 of 135 cases, 2 of 25 cases, and 3 of 209 cases) (26). Malignancy can rarely develop in giant cell tumors, either primarily or more commonly secondarily, after radiation or surgery. When malignancies do develop, they are usually high-grade sarcomas with a poor prognosis (27).
Pain is the most common symptom and is seen in up to 97% of patients (26). In giant cell tumors of the spine, radiculopathy may result from irritation of adjacent nerve roots. Initially, the pain is intermittent and relieved by rest; however, it eventually becomes persistent (26). An associated soft tissue mass often is present (35 of 135 patients) (28).
Pathology
Although giant cell tumors are characterized by multinucleated giant cells, the presence of giant cells is not specific and may be seen in numerous other lesions, including
chondroblastoma, chondromyxoid fibroma, ABC, and osteosarcoma (26). Most of the tumor is composed of mononuclear round or spindle-shaped fibroblastic mesenchymal cells (26). Jaffe et al. (29) stated that the aggressiveness of this lesion is determined by the stromal cells. Many histologists divide giant cell tumors into three grades according to the degree of malignant features, but the presence of metastatic disease does not always correlate with the grade.
chondroblastoma, chondromyxoid fibroma, ABC, and osteosarcoma (26). Most of the tumor is composed of mononuclear round or spindle-shaped fibroblastic mesenchymal cells (26). Jaffe et al. (29) stated that the aggressiveness of this lesion is determined by the stromal cells. Many histologists divide giant cell tumors into three grades according to the degree of malignant features, but the presence of metastatic disease does not always correlate with the grade.
Imaging
Plain films show a lytic lesion with an expansile appearance. Rarely, the border of this lesion is sclerotic. In the spine, no definite characteristic radiographic appearance is seen. CT can show an associated soft tissue mass (28).
MR is readily able to demonstrate the bony and soft tissue components of the lesion. Unenhanced T1-weighted images can show the extent of the tumor within the bone because the lesion displaces the normal higher signal of fat-containing marrow. The extent of the lesion is also well assessed. On T2-weighted images, giant cell tumors may be inhomogeneous, with areas of decreased and increased signal intensity. Areas of decreased T2 signal are typically related to intralesional blood products (Fig. 21.16). After the administration of contrast, T1-weighted images may help to differentiate enhancing tumor from adjacent normal structures.
Treatment
The usual treatment for this tumor is curettage. If surgery is not optimal because of location or numerous recurrences, then radiation therapy can be used. One long-term study of 18 patients found embolization to be a useful treatment option.
Sacrococcygeal Teratoma
Sacrococcygeal teratomas are rare tumors of childhood. They arise from multipotential cells of Hensen’s node that migrate to lie within the coccyx. As a result, the soft tissue mass may be accompanied by bony abnormalities of the coccyx. The American Academy of Pediatrics grades these tumors into four types. Type 1 tumors are almost always completely external and distort the buttocks. Type 2 tumors have an intrapelvic portion, but most of the tumor is external. Type 3 tumors are predominantly intrapelvic with significant displacement of invasion of surrounding structures. Type 4 tumors have no external portion, and almost all of the tumors are intrapelvic.
Sacrococcygeal teratomas occur in 1 in 35,000 to 40,000 births (28). Most sacrococcygeal teratomas are benign and identified at birth. They are associated with a high frequency of other congenital anomalies, especially anorectal malformations. Females predominate over males in a ratio of 4:1. Malignant lesions tend to be more common in males. Malignancy is also more frequent in lesions with a greater internal component (types 3 and 4), with a long delay in diagnosis and treatment and with more solid and fewer cystic components.
Pathology
Grossly, these tumors can be cystic, solid, or a combination of the two. Histologically, tissues arising from all three germ layers can be found. These tumors often contain squamous or intestinal epithelium, appendages, cartilage, bone, or neuroglial fibers. They tend to be very vascular.
Imaging
Plain films may show a pelvic soft tissue mass. Coccygeal erosion may or may not be present. The mass can lie in a presacral location and displace pelvic structures such as the urinary bladder or bowel loops. The solid portions of this tumor are calcified in 60% of all cases (30). CT is more sensitive than plain film or MRI for demonstration of calcification. Both CT and MRI may demonstrate focal areas of fat, which are highly suggestive of the diagnosis.
MR shows a mass adjacent to the coccyx (Fig. 21.17). Any intrapelvic or external components can be assessed. The lesion may be entirely solid, or it may have cystic components. Although cysts generally appear to be of decreased intensity on T1-weighted images or increased intensity on T2-weighted images, some cysts may have different intensities if they contain hemorrhage. Focal fatty components are hyperintense on both T1- and T2-weighted images. After the administration of gadolinium, solid portions of the tumor enhance.
The diagnosis can also be made prenatally, with either sonography or fetal MRI. A series of 22 patients demonstrated prenatal MRI to be more accurate than ultrasound in characterizing extent of the tumor and compression of adjacent organs (31).
Treatment
The treatment for both benign and malignant sacrococcygeal teratomas involves immediate surgical excision. These tumors have increased malignant potential with age; therefore, early surgery is advocated. The prognosis for benign lesions is excellent, although there may be morbidity secondary to surgical damage of the sacral plexus or to severe blood loss at the time of surgery because of the vascular nature of these tumors (30). If metastases occur, these tumors have an extremely poor prognosis because there is limited response to chemotherapy or radiation therapy.
Eosinophilic Granuloma
Eosinophilic granuloma is a nonneoplastic condition with an unknown etiology. The disease is most common in children. In one series of 28 patients, the disorder was most frequently seen in the 6- to 10-year-old age group. In another series of 46 patients, 38% were less than 10 years of age, and an additional 26% were between 10 and 19 years old (30). Overall, there is a male predilection, with 36 cases found in males and 7 cases in females in one review.
Symptoms are extremely variable, ranging from nonexistent to severe. The most common symptom is localized pain with or without an associated soft tissue mass. Systemic symptoms and signs, such as fever and weight loss, may also be present. The duration of the symptoms can range from days to months.
These lesions can be single or multiple. In a study of 46 patients, 36 had solitary lesions and 10 had multiple lesions.
Overall, the skull, pelvis, vertebrae, ribs, and long bones can be involved. When the lesions are multiple, the ribs and vertebrae are involved more commonly, frequently at numerous levels. With spinal lesions, collapse of the vertebral body can result in spinal cord compression, nerve root impingement, and deformity of the spine (32). Complete or near-complete vertebral body collapse, termed “vertebra plana,” may be seen with eosinophilic granuloma.
Overall, the skull, pelvis, vertebrae, ribs, and long bones can be involved. When the lesions are multiple, the ribs and vertebrae are involved more commonly, frequently at numerous levels. With spinal lesions, collapse of the vertebral body can result in spinal cord compression, nerve root impingement, and deformity of the spine (32). Complete or near-complete vertebral body collapse, termed “vertebra plana,” may be seen with eosinophilic granuloma.
Pathology
Initially, these lesions are cystic and hemorrhagic. The cysts vary from 1 to 4 cm in size and have a yellow to reddish brown appearance. As these lesions evolve, they develop increased amounts of lipid and appear friable and yellow. They heal into gray fibrous lesions, with bone formation in the later stages.
Histologically, these lesions are initially infiltrated by many eosinophils, accompanied by variable numbers of lymphocytes. Finally, in the healing stages, connective tissue is present, which in turn is transformed into bone.
Imaging
Plain films show round or oval, sharply marginated lytic lesions with well-defined borders. There may or may
not be an associated soft tissue mass. CT demonstrates findings similar to the plain films and better delineates any associated soft tissue mass.
not be an associated soft tissue mass. CT demonstrates findings similar to the plain films and better delineates any associated soft tissue mass.
On MR, lesions usually have decreased signal intensity on T1-weighted images and increased signal intensity on T2-weighted images, unless hemorrhage is present. Spinal cord compression is well delineated. When the vertebral body is involved, it is usually affected in its entirety. The weakened vertebral body can collapse, resulting in vertebra plana (Fig. 21.5). A secondary kyphosis may develop. Associated epidural hematoma also can cause spinal cord compression. The lesions may be difficult to differentiate from metastatic disease.
Chordoma
Chordomas constitute 3% to 4% of all primary bony tumors (33). They arise from remnants of the notochord. Because the notochord, which forms the early fetal skeleton, extends from the clivus to the sacrum, chordomas can occur anywhere along the skull base and spine: 50% arise in the sacrum, 35% in the clivus, and 15% in the vertebrae (33).
In the spine, the areas most commonly involved are the cervical, lumbar, and, finally, the thoracic spine, in descending order of frequency. There is a definite male predominance, with roughly a 2:1 male-to-female ratio. Of 155 cases from the Mayo Clinic, 103 were male and 52 were female. In this same study, the age range was from 8 to 83 years, with an average age at diagnosis of 48 years.
Pain is the most common symptom in spinal chordomas, and is usually localized to the site of origin. As they grow, vertebral chordomas can show signs and symptoms of cord compression. In one series of 46 cases, the average duration of symptoms to the time of diagnosis was almost 1 year.
Although chordomas often invade adjacent structures, they metastasize less frequently. However, chordomas arising in the vertebral bodies are more malignant than their counterparts in the sacrum or the clivus. Although metastases have been reported in 10% to 15% of all cases, metastases occur in 80% of the vertebral body cases.
Pathology
Grossly, chordomas may be soft or firm. They often appear lobulated. Histologically, these lesions are composed of large, vacuolated physaliferous cells. The cells are usually arranged in cords and contain abundant glycogen. Fibrous septae subdivide the tumors into lobules.
Imaging
Plain films show bony destruction with areas of amorphous calcification in 50% to 70% of the cases. In one study of 16 cases, 7 patients exhibited involvement of two or more adjacent vertebral bodies and the intervening disc, a finding generally associated with infectious etiologies and unusual in neoplasms. In addition, paravertebral masses can be seen.
CT can better demonstrate the calcification and paravertebral soft tissue masses. CT after intravenous contrast or after myelography can show an associated epidural component. Chordomas may show areas of hypodensity on CT, likely reflecting mucinous content or cystic degeneration (34).
MR is inferior to CT in showing bony destruction or calcification (35). MR, however, is superior in outlining epidural disease and the true extent of disease involving the bone (Figs. 21.18 and 21.19). These lesions may involve neural foramina, mimicking nerve sheath tumors (34). Seventy-five percent of chordomas are isointense to cord on T1-weighted images and 25% are hypointense. The lesions are high signal on T2-weighted images (35). Seventy percent of cases show internal septations and a surrounding capsule of low signal intensity. Areas of hemorrhage and cystic change are readily demonstrated if present. After the administration of contrast, prominent enhancement is typically seen.
Treatment
Treatment consists of surgical resection with radiation therapy. The prognosis is poor, with a 5-year survival rate of 10%. In one study, an average survival of 3 years after the time of diagnosis was seen. Although metastases are not common, local recurrence is the major problem, usually within 2 to 4 years after initial surgery and radiation therapy.
Neuroblastoma, Ganglioneuroma, and Ganglioneuroblastoma
Neuroblastoma, ganglioneuroma and ganglioneuroblastoma are considered together because they all arise from the same primitive cells—neuroblasts, which are of neural crest origin (36). These tumors, however, vary significantly in degree of cell maturity and behavior. The neural crest cells embryologically form the adrenal medulla and the paravertebral sympathetic chain. Therefore, neuroblastomas (the most common of the three) can originate in the adrenal medulla (36% to 40%) or in the paravertebral sympathetic chain. The adrenal medulla and upper abdominal parasympathetic chain are the primary sites of 65% of neuroblastomas (36) Ganglioneuroma is the most well differentiated lesion and is composed almost entirely of mature ganglia cells.
Neuroblastoma is a disease of infancy and childhood that occurs in 1 in every 10,000 births. Children less than 5 years of age are affected most often (36). Excluding central nervous system (CNS) tumors, neuroblastoma is the most common solid tumor of children (36). There is a slight male predilection in some studies (36). Both ganglioneuroma and ganglioneuroblastoma tend to present later than neuroblastomas and are most often seen in the 5- to 8-year-old age group.
Because the tumors often originate in a paraspinal location, they can extend through the neural foramina to impinge on the thecal sac. Epidural extension occurred in 17 of 129 cases of neuroblastoma in a study from the Hospital for Sick Children. One percent to 4% of patients present with spinal cord compression. Punt et al. (37) reviewed the records of 21 children with neuroblastoma who presented with spinal cord compression. Four children had spinal cord compression at birth (37).
Involvement of the spine occurs most frequently in the thoracic and lumbar regions and is rare in the cervical area. In Punt et al.’s (37) series of 21 cases with cord compression, 9 occurred in the thoracic region, 5 in the thoracolumbar, 6 in the lumbosacral, and only 1 in the cervical region.
Symptomatology can vary tremendously according to the location and extent of disease. With intraspinal involvement, the most common presenting symptoms include local pain and spinal cord dysfunction (36). The patient may notice a paraspinal mass or may have signs of spinal cord compression. In one series of 11 cases, impaired motor and sphincter function were seen in 8, weakness of the lower extremities in 6, and weakness of the arms in 2. Cord compression is also common in terminal stages of the disease because of the frequent occurrence of osseous metastases. Actual brain and spinal cord parenchymal metastases from neuroblastoma are rare, although leptomeningeal tumor spread is not unusual.
Pathology
Histologically, neuroblastomas are composed of small, round cells with hyperchromatic dense nuclei, which can be confused with Ewing sarcoma, rhabdomyosarcoma, lymphoma, and Wilms tumor (36). Neuroblastoma is composed of primarily primitive cells and lacks elements characteristic of further maturation, such as increased size of nuclei, increased amount of cytoplasm, and production of fibrillar elements (36). The tumor is frequently hemorrhagic, and calcifications are seen in 10% of cases.
Ganglioneuroblastoma is a mixture of immature neuroblastoma and more mature elements, whereas ganglioneuroma is composed of primarily mature cells. The nuclei are large, and there is more cytoplasm present within the cells than in neuroblastoma. As the axonal processes develop, more mature fibrillary structures
are seen. Calcifications are seen in 20% of cases (36). Neuroblastomas can differentiate into ganglioneuroma. In fact, neuroblastoma metastases can have ganglioneuroma elements within them.
are seen. Calcifications are seen in 20% of cases (36). Neuroblastomas can differentiate into ganglioneuroma. In fact, neuroblastoma metastases can have ganglioneuroma elements within them.
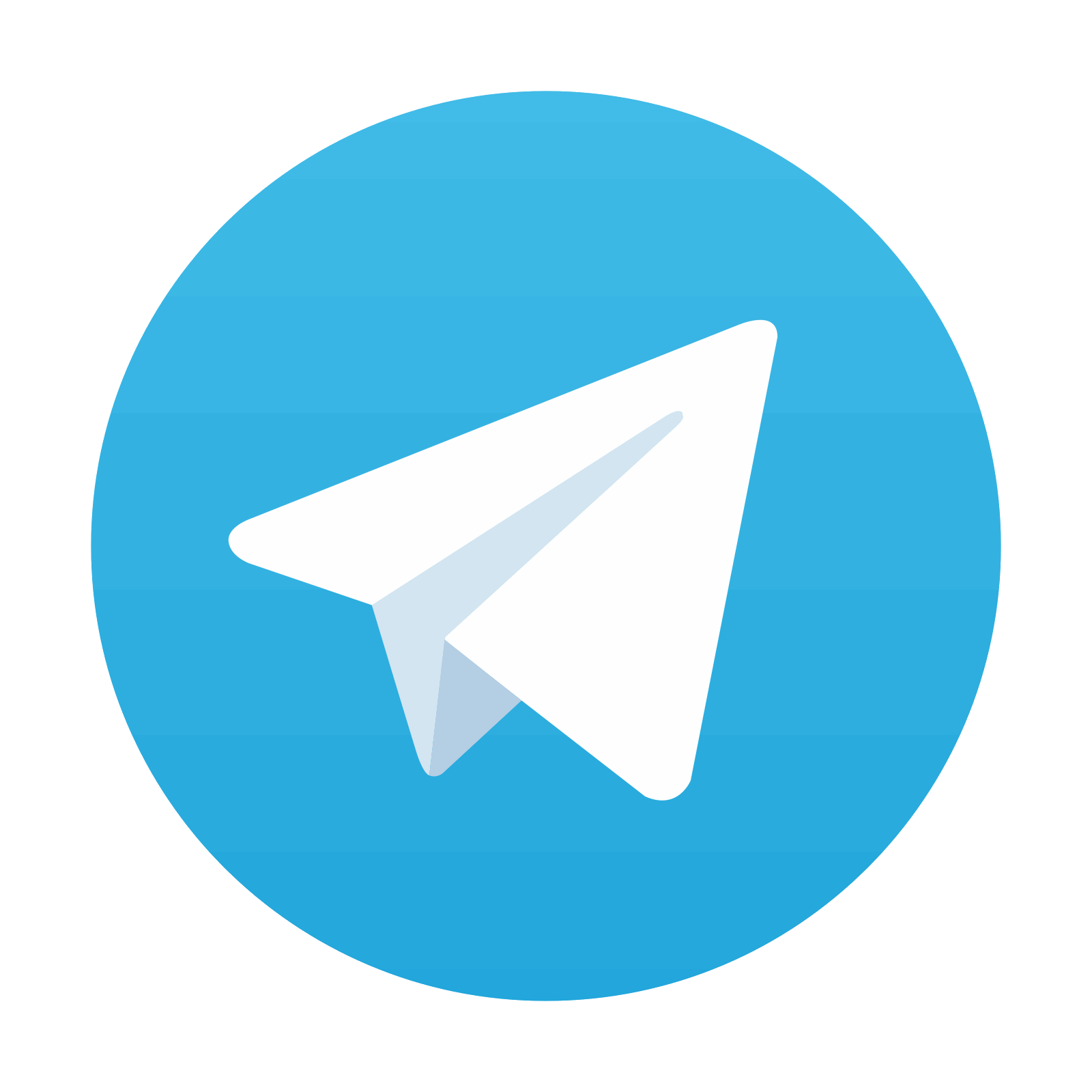
Stay updated, free articles. Join our Telegram channel

Full access? Get Clinical Tree
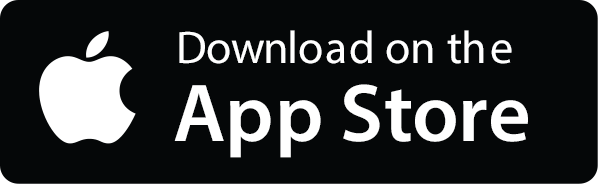
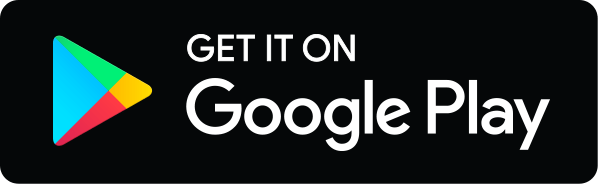
