Fig. 15.1
Phases and lesion morphology of coronary atherosclerosis progression according to gross pathological and clinical findings (Reproduced from Fuster et al. [11] with permission)
Table 15.1
Atherosclerotic lesion types
Plaque type | Characteristics of plaque | Associated clinical syndrome |
---|---|---|
I | Intimal thickening, macrophages, isolated foam cells | Asymptomatic |
II “fatty streak” | Accumulation of intracellular lipid in infiltrating macrophages and smooth muscle cells | Asymptomatic |
III | As above, plus incipient extracellular lipid and connective tissue deposition | Asymptomatic |
IV “atheroma” | Large extracellular intimal lipid core; inflammatory cell infiltration, including macrophages, foam cells, and T cells | Usually asymptomatic; can also be associated with stable angina |
Va | Atheroma with fibrous layer or layers | Same as type IV |
Vb | Atheroma with extensive calcification in the lipid core or elsewhere in the lesion | Stable angina pectoris; can also be asymptomatic |
Vc | Fibrosed atheroma or organized mural thrombus with minimal or absent lipid component | Same as type Vb |
VI “complicated lesion” | Disrupted type IV or V lesion with intramural hemorrhage and/or overlying thrombus | Acute coronary syndrome or asymptomatic lesion progression |
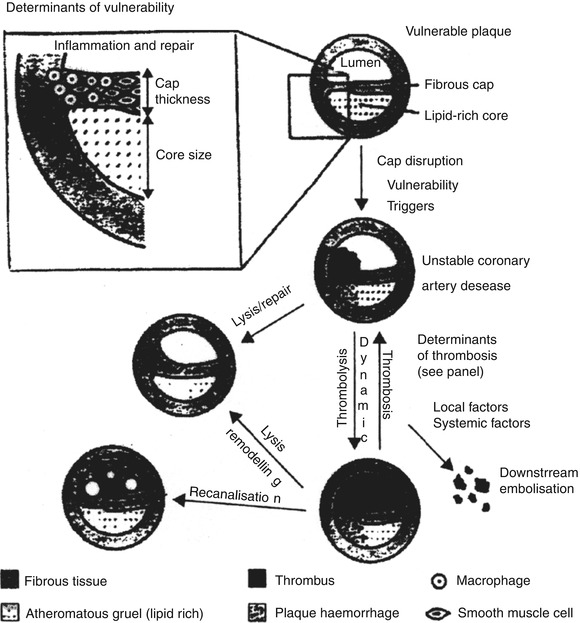
Fig. 15.2
Plaque vulnerability, disruption, and thrombosis: anatomical changes leading to acute coronary syndromes and subsequent plaque remodeling (Reproduced from Fuster et al. [11] with permission)
The atherosclerotic plaque tends to grow in fits and starts, rather than in a continuous fashion. Sudden plaque growth occurs when the plaque ruptures or ulcerates, resulting in intracoronary thrombosis [7, 8] (Fig. 15.3). Even mildly or moderately stenotic arteries can acutely progress to severely or totally occlusive lesions [6] (Fig. 15.4). They account for many acute coronary syndromes (unstable angina, acute infarction) but also may remain asymptomatic.
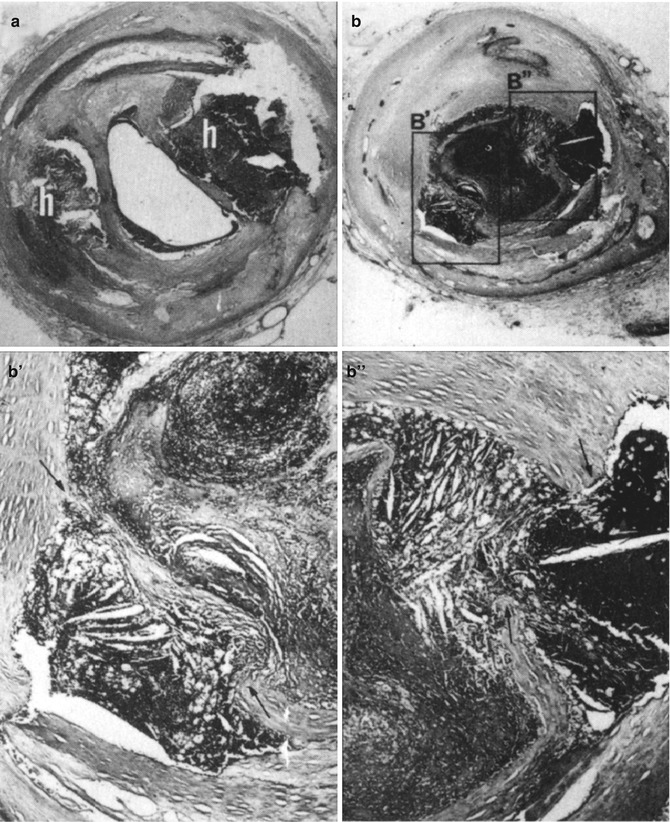
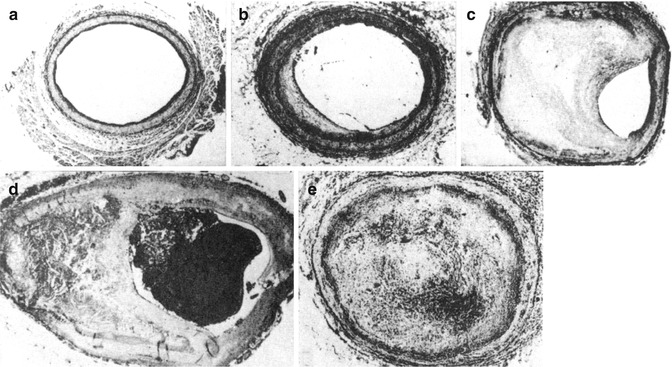
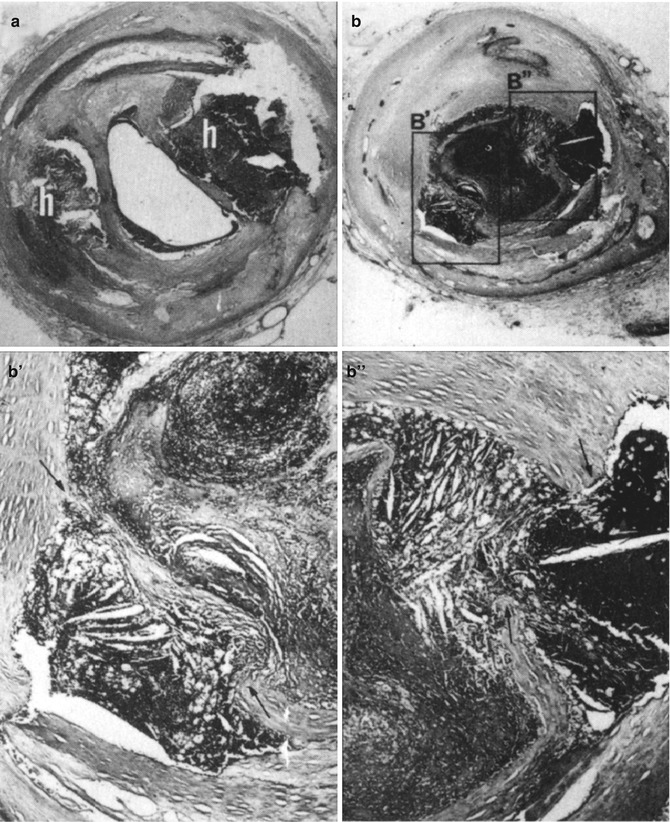
Fig. 15.3
(a, b) Two consecutive segments of atherosclerotic coronary arteries, 1 cm apart: (a) plaque hemorrhage (h), (b) plaque rupture with extrusion of atheromatous debris inducing thrombosis. Areas boxed in b are shown at higher magnification in B′ and B″. Note extruded cholesterol/fatty acid material, through breaks (arrows) in intimal lining of lumen, admixed with thrombus (Reproduced from Fuster and Kottke [12] with permission)
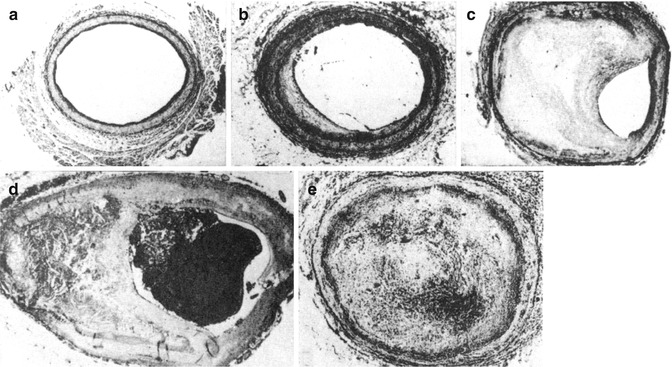
Fig. 15.4
(a–e) Slow progression of coronary atherosclerosis: (a) normal artery, (b) fatty streak with fibrosis, (c) advanced fibrous plaque. Rapid progression of coronary atherosclerosis: (d) occlusion by thrombus formation, (e) thrombus during process of fibrotic organization (Reproduced from Fuster and Kottke [12] with permission)
Plaque disruption occurs not only in advanced stenotic plaques, but independently of lesion size and degree of stenosis [8]. Most acute coronary syndromes actually result from the disruption of lesions which are initially not flow limiting, due to rapid progression to severe stenoses [9, 10]. Culprit lesions in acute coronary syndromes tend to have less calcification and fibrous tissue, which implies softness and vulnerability to shear forces [15] (Fig. 15.3).
Nevertheless, lipid composition of a disrupted lesion can vary. Disrupted and thrombosed plaques with little lipid content are described in a recent autopsy report of plaque morphology in sudden cardiac death [16].
The unstable phase of plaque development may be followed by a chronic phase with a calcified type Vb or fibrotic type Vc lesion (Fig. 15.4). It is often marked by stable angina pectoris. Severely stenotic plaques can occlude without plaque rupture due to stasis, leading to a thrombus. Occlusion of such a vessel is often clinically silent, as distal myocardium is frequently supplied by collateral flow [17].
The primary and secondary prevention of acute coronary syndromes includes aggressive cholesterol-lowering therapy, demonstrated to significantly improve prognosis following acute coronary syndromes and in hypercholesterolemic patients without a history of coronary disease [18–20]. The change does not appear to involve only a minimal regression of the atherosclerotic plaque, as demonstrated in a number of angiographic studies [21, 22]. Rather, lowering of cholesterol plus the more recently appreciated anti-inflammatory effects of statins stabilizes the atheromatous plaque by increasing the net efflux of lipid from the plaque, thereby decreasing the lipid content, decreasing the number of macrophages, and lowering of indices of inflammation such as local temperature, uptake of FDG, and formation of a thicker fibrous cap. This helps make the plaque more resistant to disruption [7, 23–25]. Furthermore, the anti-inflammatory effects of statin therapy independent of cholesterol lowering appear to promote the stabilization of lesions in acute coronary syndromes [26].
15.3 Myocardial Perfusion SPECT Imaging
Clinical manifestations of coronary artery disease include angina pectoris, myocardial infarction, congestive heart failure, and sudden death. It may be asymptomatic until advanced in severity or complications. Most diagnostic methods, both invasive and noninvasive, depend on the detection of luminal narrowing of the epicardial coronary vessels. Vessel narrowing of up to 75 % of the cross-sectional area (or <50 % of luminal narrowing) does not affect resting coronary flow. Increase of coronary flow caused by exercise or pharmacological stress exaggerates flow nonuniformity, through either increased metabolic demand or vasodilation [27] (Fig. 15.5). The easiest method of increasing coronary flow is physical exercise, using a motorized treadmill or a stationary bicycle. In patients who are unable to exercise adequately, pharmacological agents (adenosine, dipyridamole, regadenoson, dobutamine, and arbutamine) are used for transient elevation of coronary flow.
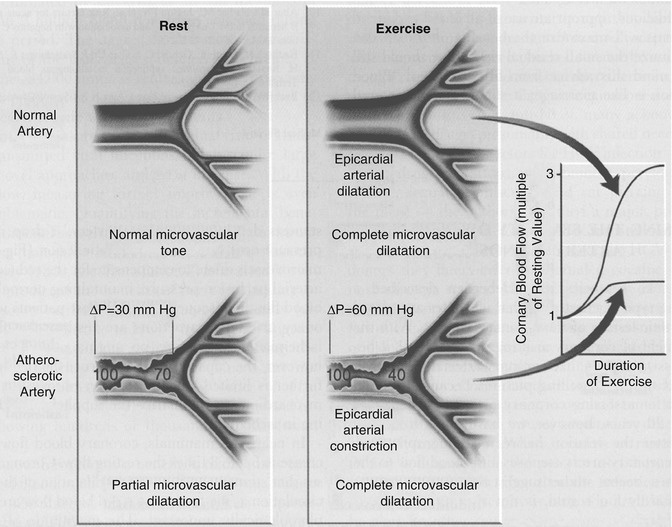
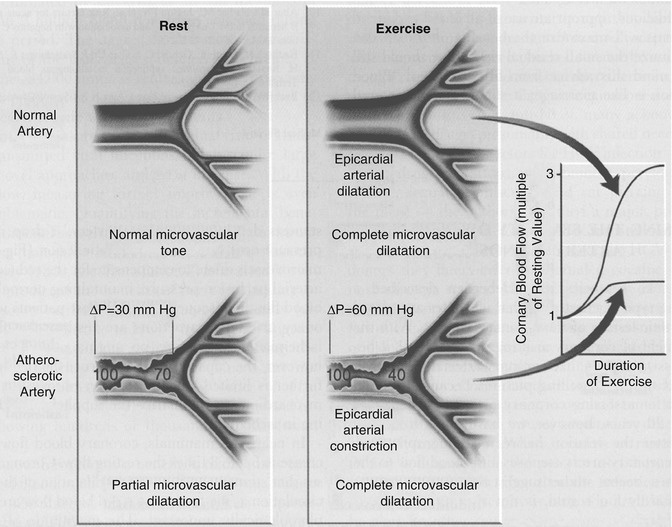
Fig. 15.5
Regulation of blood flow in normal and stenosed coronary arteries: In the normal artery, the epicardial and microvascular vessels dilate with exercise or pharmacological stress. In the atherosclerotic artery, when the patient is resting, there is a drop in pressure across the stenosis in the epicardial artery. During exercise or vasodilation, the pressure drop across the stenosis increases, and the microcirculation has limited additional capacity for dilation, resulting in blood flow not adequate to meet the metabolic demand of exercise or to keep up with increased blood flow in the normal regions. As a result, the fractional flow reserve is reduced (Reproduced from Wilson [29] with permission)
Myocardial perfusion imaging (MPI) maps the relative distribution of coronary flow, which is normally almost uniform in the absence of prior infarction or fibrosis. In the presence of luminal narrowing, flow nonuniformity corresponds to the anatomical location of the coronary stenoses and to the cumulative severity of the obstructions along the coronary arterial tree, the size of its watershed, modulated by the presence, size, and flow reserve of collateral vessels [28]. Therefore, MPI can diagnose not only the presence of coronary artery disease, but also its extent, severity, and physiological impact, thereby providing great prognostic power.
15.3.1 SPECT Tracers
The ideal tracer of coronary flow would be extracted by the myocardium with 100 % efficiency, like microspheres. Its myocardial uptake would be linearly related to coronary flow, and the tracer isotope would have optimal emission photon energy for imaging with a gamma camera. Currently, two classes of tracers are widely used for conventional MPI: thallium-201 and the Tc-99m-labeled tracers. Three agents are approved for clinical use in the USA: thallium-201, Tc-99m sestamibi, and Tc-99m tetrofosmin. None of the available conventional agents have all characteristics of an ideal myocardial perfusion agent.
15.3.1.1 Thallium-201
Thallium-201 (Tl-201) has been in clinical use for more than three decades. Tl-201 is a metal in group III-A of the periodic table and, as an isotopic cationic tracer, has properties similar to those of potassium. Tl-201 is extracted with a high extraction fraction by the ATPase-dependent Na+/K+ channels (Table 15.2). Diagnostic and prognostic data on Tl-201 are extensive. Another advantage of Tl-201 is the ease of use. Only one injection is needed [30]. Among the conventional tracers, Tl-201 is the one preferred for evaluation of myocardial viability. Major drawbacks of Tl-201 include low energy of its principle X-ray photons (69–80 keV) and its long half-life (72 h), which limits the injected dose, due to its relatively higher radiation dose to the patient. The limited dose leads to suboptimal image quality due to noise. The low emission energies lead to a high scatter fraction in the imaging energy window and greater susceptibility to attenuation, especially in obese and female subjects. Compared with competing modalities (stress echo, Tc-99m-based tracers), a Tl-201 study is relatively long (4–6 h) in duration.
Table 15.2
Tracers for SPECT myocardial perfusion imaging
Thallium-201 | Tc-99m sestamibi | Tc-99m tetrofosmin | |
---|---|---|---|
Brand name | N/a | Cardiolite | Myoview |
Class | K+ analog | Isonitrile | Diphosphine |
Preparation | Cyclotron | Kit (heated) | Kit (cold) |
Charge | Cation | Cation | Cation |
Lipophilicity | Low | High | High |
Redistribution | Yes | Minimal | Minimal |
Tissue clearance | 50 %/4 h | >6 h | >6 h |
Excretion | Renal | GI (renal) | GI (renal) |
Time of imaging (min) | 5–10 | 20–60 | 10–45 |
Completion time (h) | 4–6 | 3–4 | 3–4 |
Counts | Adequate | High | High |
SPECT | Yes | Yes | Yes |
Extraction | 0.85 | 0.39 | 0.24 |
Gating | ± | Yes | Yes |
Heart-liver(1 h) | 2.6 | 1.2 | 1.4 |
TEDE (rem/3.5 mCi) | 2.1 | 1.1 | 0.8 |
Clinical use | |||
Diagnosis | Yes | Yes | Yes |
Prognosis | Yes | Yes | Yes |
Viability | Yes | Yes | Yes |
A dose of 3–4 mCi of Tl-201 is injected intravenously at peak stress (exercise or pharmacological). Stress SPECT imaging starts 10–15 min following the injection. Tl-201 uptake in the myocytes requires active metabolic transport, i.e., only viable myocytes take up and retain the tracer. Washout of Tl-201 from the myocardium starts immediately after initial uptake. Initial tracer uptake is a function of tracer serum concentration, which is highest after injection, before complete extracellular volume mixing, the coronary flow, and of the integrity of the myocytes. Early (post stress) images reveal the degree of regional uniformity of the coronary flow, i.e., demonstrate presence or absence of coronary artery disease. Washout of Tl-201 from the myocardium is faster from areas with higher initial uptake (and higher regional flow) and is slower from hypoperfused but viable areas supplied by arteries with inadequate flow during stress. This leads to a “filling in” or “redistribution” of initial defects (Fig. 15.6). The customary time for delayed imaging is 3–4 h after tracer injection. Tl-201 redistribution is consistent with presence of viable but hypoperfused (or ischemic) myocardium. Delayed imaging (up to 24 h) may reveal redistribution in some defects which appeared “fixed” at 3–4 h. In addition, or alternatively, reinjection of a small booster dose of Tl-201 (1 mCi) at rest may reveal improved uptake in regions with “fixed” defects [31–35]. On the other hand, regions with old infarction, where viable myocardial mass, not vessel diameter, is the flow-limiting factor, do not demonstrate redistribution, i.e., replacement of contractile myocardium by fibrotic tissue. Figure 15.7 shows typical Tl-201 SPECT stress and redistribution images and both types of myocardial behavior.
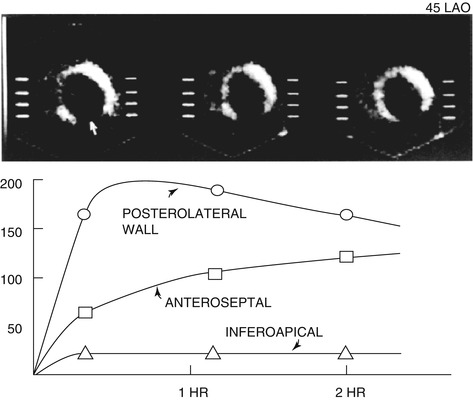
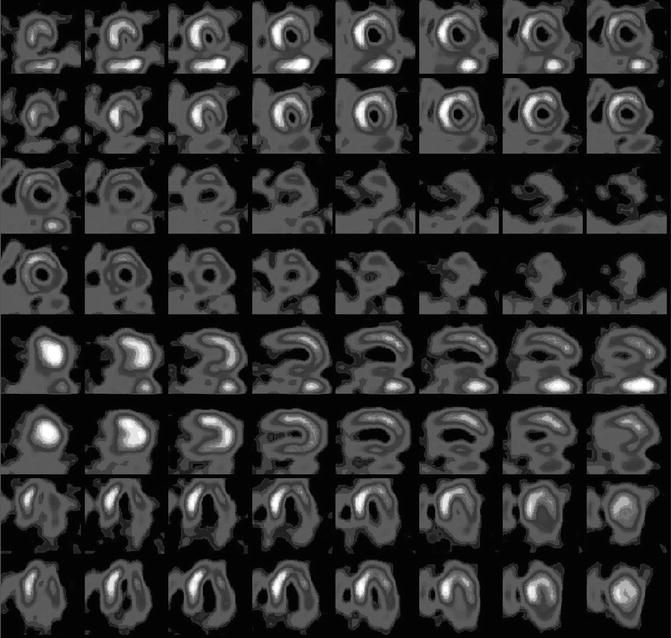
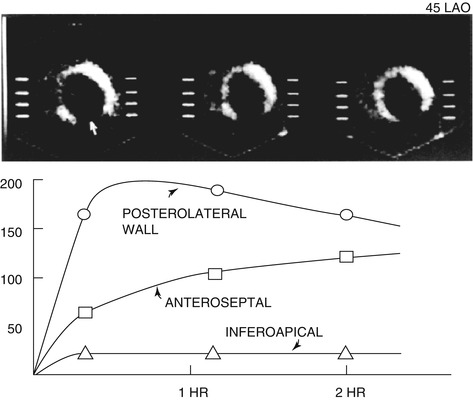
Fig. 15.6
Sequential LAO planar images obtained 10 min, 1 and 2 h after low-level treadmill exercise, 10 days after a myocardial infarction. There is a persistent defect involving the inferoapical segment (white arrow) compatible with scar. The posterolateral wall shows normal uptake and washout, while the anteroseptal region demonstrates an initial defect with delayed redistribution indicative of ischemia (Reproduced from Gibson and Beller [36] with permission)
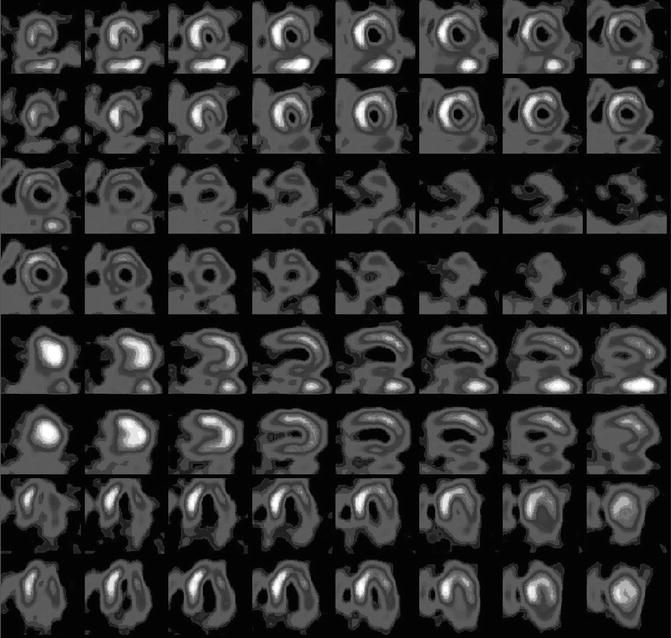
Fig. 15.7
Thallium-201 stress and redistribution images of a patient showing a severe fixed inferolateral defect; a severe, partially reversible basal inferolateral defect; a reversible basal inferoseptal defect; and a mild to moderate reversible anterolateral defect
Overall sensitivity of Tl-201 SPECT imaging for the detection of CAD is >80 %. Sensitivity for detection of multivessel CAD is higher than for detection of single-vessel disease. Sensitivity for detection of left anterior descending and right coronary artery disease is higher than for detection of circumflex disease [37]. Specificity of the findings is 50–80 %, depending on the degree of catheterization and laboratory referral bias. Specificity is lower in patients with LBBB, left ventricular dysfunction, end-stage congestive heart failure, left ventricular hypertrophy, and/or marked obesity [38].
15.3.1.2 Tc-99m Sestamibi, Tc-99m Tetrofosmin
The technetium-99m-based tracers, Tc-99m sestamibi (Cardiolite), and Tc-99m tetrofosmin (Myoview), have more similarities than differences (Table 15.2). A shorter physical half-life of Tc-99m (6 h) allows the use of higher tracer doses (up to 50 mCi/day). Combined with more optimal photon energy for gamma camera imaging (140 keV) compared with Tl-201, image quality is less noisy, and frequency and severity of attenuation artifacts are decreased. Negligible washout of Tc-99m-based tracers [39] (Fig. 15.8) necessitates the use of two separate tracer injections: one for rest imaging and one for stress imaging. SPECT imaging is usually started 20–60 min after tracer injection. The delay is needed for blood pool clearance and partial liver clearance (Fig. 15.8). Gating of Tc-99m sestamibi or Tc-99m tetrofosmin images, made possible because of the high photon flux, allows simultaneous evaluation of perfusion and resting function. Widely available commercial software is used for the quantification of left ventricular ejection fraction, left ventricular volumes, and left ventricular mass and for semiquantitative evaluation of myocardial systolic thickening [41] (Fig. 15.9). In laboratories equipped with a suitable gamma camera positioned at the side of the treadmill or upright bicycle, first-pass blood pool imaging can be performed for the evaluation of left and right function during exercise stress [42].
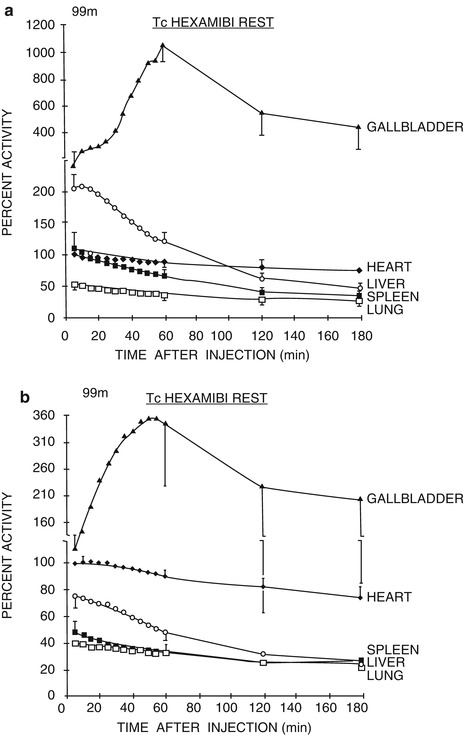
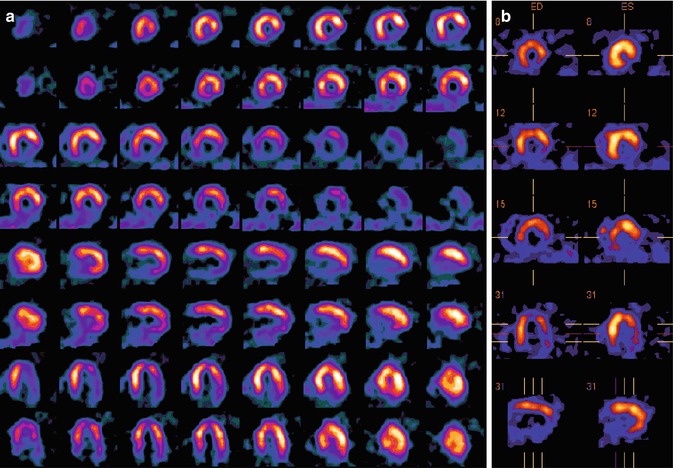
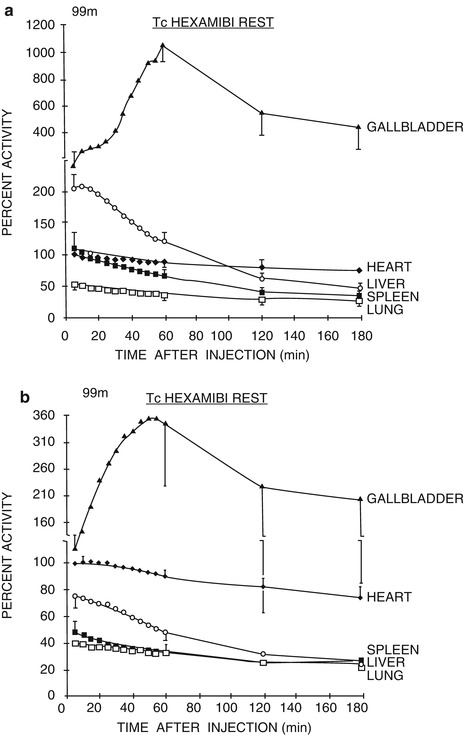
Fig. 15.8
(a) Organ time-activity curves after injection of Tc-99m sestamibi at rest in five normal volunteers (mean ± SD). The data are normalized to cardiac activity at 5 min after injection. (b) Organ time-activity curves in five normal volunteers after injection of Tc-99m sestamibi during exercise (Reproduced from Wackers et al. [40] with permission)
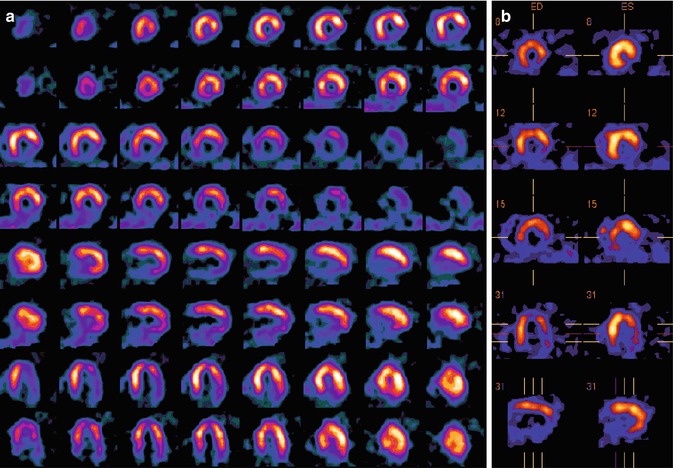
Fig. 15.9
(a, b) Stress and rest gated Tc-99m sestamibi imaging study. (a) Stress and rest Tc-99m sestamibi SPECT images showing severe inferolateral and posterolateral defects at stress with improvement in the inferoapical and lateral walls at rest and persistence of the inferior and posterior defects. (b) Stress Tc-99m sestamibi imaging with gating of the same patient as in (a), showing end-diastolic and end-systolic gated images. There is excellent wall thickening in the septum and anterior wall, absence of uptake or thickening in the inferolateral and posterolateral walls, and decreased thickening in the basal septum
Tc-99m sestamibi and Tc-99m tetrofosmin can also be used in the setting of acute coronary syndromes. Patients with recent or current episodes of chest pain can be injected with the tracer at rest, medically stabilized or treated with thrombolysis or percutaneous revascularization, and imaged for diagnostic or prognostic purposes several hours later [43]. Patients in whom an acute infarction has been excluded, and at low risk for unstable angina, can be referred from the emergency department or chest pain unit to undergo stress and rest imaging.
Excretion of the Tc-99m tracers is hepatobiliary and, to a lesser extent, renal [44]. High subdiaphragmatic uptake in the liver or intestines occasionally interferes with the evaluation of cardiac perfusion. The average total length of the rest-stress imaging and stress-rest sequence is 3–4 h. In direct comparisons, the diagnostic accuracy of sestamibi and tetrofosmin is similar to that of Tl-201 [45, 46]. However, the linearity between flow and uptake of Tc-99m sestamibi or Tc-99m tetrofosmin is suboptimal at high flow rates (Fig. 15.10), due to the lower extraction fraction, which is achieved with coronary vasodilators (adenosine and dipyridamole) [47–49].
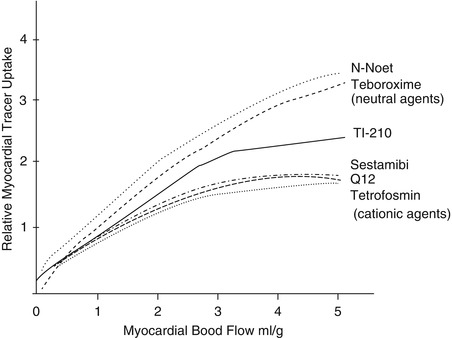
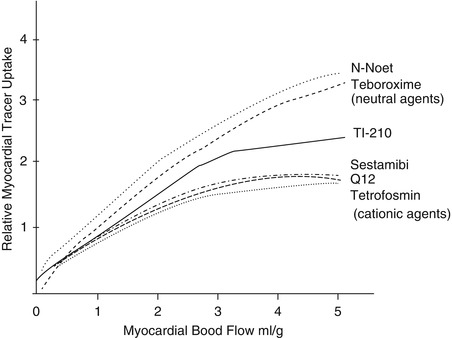
Fig. 15.10
Relationship between myocardial blood flow and uptake of various perfusion tracers (Reproduced from Jain [44] with permission)
In an attempt to utilize advantages of both types of tracers, dual-tracer protocols were developed: Tl-201 is injected at rest, and rest imaging is started within 15 min. Exercise or pharmacological stress is followed by Tc-99m MIBI or Tc-99m tetrofosmin injection at peak stress. The test is completed in less than 3 h [50]. A potential drawback derives from different pharmacokinetic properties of the tracers, resulting in nonparallel flow-uptake relationships and different spatial contrast due to different isotope energies.
Tc-99m teboroxime (Cardiotech) was another FDA-approved perfusion agent that was marketed about two decades ago. This highly lipophilic agent readily crosses myocardial cellular membranes but, unlike MIBI, rapidly diffuses out of the cells in 6–10 min, allowing only a few minutes for imaging [51]. It requires a separate rest and stress injection. There is some evidence that the differential washout rate of Tc-99m teboroxime obtained from stress-redistribution images can differentiate between ischemic and infarcted myocardium as well as stress-rest imaging [52]. One advantage of teboroxime is its very high extraction fraction, which is higher than any of the other conventional agents at high flow rates during pharmacological vasodilation. Due to the demanding rapid imaging protocol required, its use never became extensively applied, in spite of examples of good quality images obtained with either multiheaded or single-headed gamma cameras in some laboratories. Its commercial availability has been stopped for over a decade ago. Its applicability may conceivably be revived with the use of new high-speed, high-sensitivity SPECT gamma cameras.
15.3.1.3 Investigational Perfusion Radiotracers
Several new perfusion tracers have been developed and are under investigation. One type includes the so-called Q compounds (Q3 and Q12) labeled with Tc-99m. They are mixed cationic ligands, consisting of monophosphate ligands complexed to a Schiff-base ligand. Radiolabeling requires boiling in a water bath with Tc-99m pertechnetate. The Q agents have a rapid clearance from the blood by hepatobiliary and renal excretion. Myocardial uptake of Q12 is stable without redistribution. However, the first-pass extraction is only 29 %, a limitation with high-level exercise and pharmacological stress [34].
Another promising agent was Tc-99mN-NOET. It is a nitrido dithiocarbamate, a neutral lipophilic compound. It also requires boiling a precursor tri-sulfophenyl phosphine with Tc-99m pertechnetate, followed by compounding with dithiocarbamate. Blood activity clearance by hepatobiliary excretion is slower than for Tl-201 or Tc-99m MIBI or Tc-99m tetrofosmin. The agent’s features are a high extraction fraction and followed by significant washout [44]. However, high lung uptake in some patients has discouraged its clinical application [53].
15.3.2 Stressors
15.3.2.1 Exercise
Exercise treadmill stress testing (ETT) is the most frequently used test for noninvasive diagnosis of coronary artery disease. Sensitivity of a symptom-limited ETT for diagnosis of CAD is 65–70 % [54]. When combined with myocardial perfusion imaging, sensitivity increases to 85–90 %, while specificity is increased as well [37]. Motorized treadmill exercise is almost universally used in the USA, while the upright stationary bicycle is more popular in Europe and elsewhere. All monitored parameters (i.e., ECG, blood pressure, patient’s appearance, and symptoms) are valuable not only for diagnosis but also for prognosis. Using a Bayesian approach, pre- and posttest probability for presence of the disease can be reliably estimated. Short-term prognosis for major cardiovascular events can also be derived from easily obtained parameters. The single most powerful prognostic predictor in both men and women is exercise capacity (length of the exercise) [55, 56].
15.3.2.2 Pharmacological Stress Testing
Patients who cannot exercise for noncardiac reasons (e.g., orthopedic, neurological, peripheral vascular) or are unable to exercise adequately (for a meaningful period of time and/or to an adequate heart rate) are candidates for pharmacological stress testing. Five agents are currently approved for use in conjunction with MPI: adenosine, dipyridamole, regadenoson, dobutamine, and arbutamine. Adenosine, dipyridamole, and regadenoson are coronary vasodilators. Dobutamine and arbutamine are beta-adrenergic agonists and increase myocardial oxygen demand; they also have some direct vasodilatory effect [57]. Pharmacological stress makes possible evaluation of patients unable to exercise for noncardiac reasons, including sick and debilitated patients. However, physiologically useful parameters derived from an exercise test, valuable for a comprehensive evaluation, are lost.
Adenosine
Adenosine is an endogenous coronary vasodilator produced from ADP and AMP in myocardial and vascular smooth muscle cells. Adenosine affects two kinds of receptors: A1 and A2. Activation of the A1 receptor slows AV conduction. Activation of the A2 receptor leads to coronary vasodilation (Fig. 15.11, Table 15.3). The half-life of adenosine is extremely short (seconds only). Perfusion tracers are therefore injected during continuous adenosine infusion (140 μg/kg/min for 6 min). Side effects of adenosine include flushing in 37 % of patients, chest pain in 35 %, shortness of breath in 35 %, and gastrointestinal symptoms in 15 %. Chest pain is not indicative of myocardial ischemia. Vasodilation causes a modest blood pressure drop, usually accompanied by compensatory tachycardia, although transient 2nd-degree AV block is seen in 3–4 % of patients and 3rd-degree AV block in <1 % of tested patients. All side effects and hemodynamic changes are transient and reversible. Use of an antidote (IV aminophylline) is very rarely needed. Most situations can be controlled by decreasing the infusion rate and/or by shortening the duration of the infusion. Adenosine may trigger bronchospasm and should not be used in patients with bronchospastic disease, particularly those who have clinical asthma and/or are being treated with bronchodilators. Caffeine, theophylline, and their metabolites competitively block adenosine receptors. Therefore, patients should abstain from caffeine-containing beverages and medication for 12–24 h prior to the test [59].
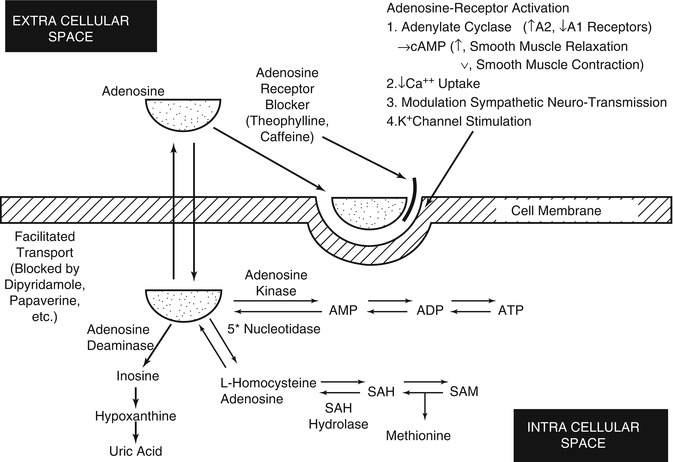
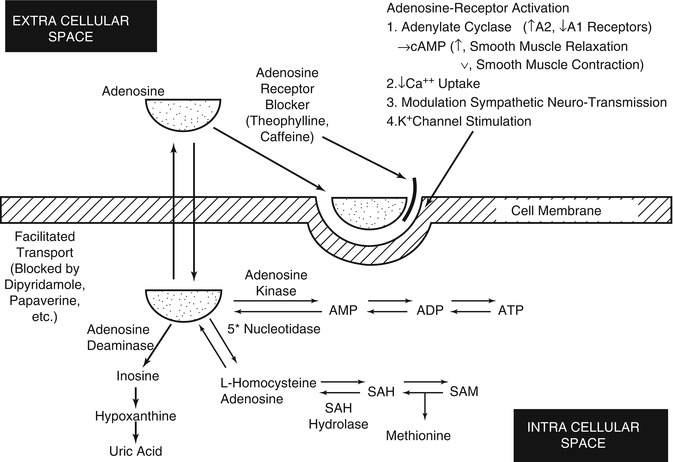
Fig. 15.11
Mechanisms of the vasodilating stress agents. Adenosine is synthesized intracellularly and leaves the cells to act on surface membrane receptors. Dipyridamole blocks adenosine reentry into the cell, increasing extracellular adenosine that can bind to the receptor. Methylxanthines, such as theophylline and caffeine, competitively block the receptor sites (Reproduced from Iskandrian et al. [58] with permission)
Table 15.3
Coronary vasodilators
Adenosine | Regadenoson | Dipyridamole | |
---|---|---|---|
Effect | Direct | Direct | Indirect |
Half-life | <10 s | 8.5 min | Minutes |
Onset of action | Seconds | Seconds | Minutes |
Time to peak effect | ca. 1 min | 20–40 s | ca. 7 min |
AV block | 7 % | 3 % | 0 % |
Diagnostic utility | Inability to exercise | Inability to exercise | Inability to exercise |
Contraindications | Bronchospasm | Bronchospasm | |
Unstable/severe ischemia | Unstable/severe ischemia | Unstable/severe ischemia |
Dipyridamole
Dipyridamole is an indirect vasodilator: It increases intravascular concentration of endogenously produced adenosine by blocking its cellular reuptake (Fig. 4.11). Dipyridamole has a longer half-life than adenosine and does not affect AV conduction. Dipyridamole is usually infused for 4 min. The perfusion tracer is injected at 7 min. In some laboratories, the patient is asked to perform low-level exercise or handgrip exercise to enhance its effects. Contraindications for dipyridamole use are similar to those for adenosine, although chest pain is less frequent. An effective antidote is IV aminophylline (50–100 mg IV), which can be used to normalize hemodynamic changes, relieve ischemia, and/or treat side effects [59, 60]. Dipyridamole potentiates the effects of exogenously infused adenosine. Therefore, oral dipyridamole, when being taken for its antiplatelet effects, should be discontinued on the day of the stress test.
Regadenoson
The first historically available vasodilator stress agents, dipyridamole and adenosine, are effective and useful, but they do have significant side effects, making stress testing an unpleasant experience in many patients, and are contraindicated in patients with bronchospastic disease. It has been established that there are four adenosine receptor subtypes distributed in various locations: A1, A2A, A2B, and A3. The selective activation of A2A receptors leads to coronary vasodilation, while A1 are responsible for decreased AV conduction and chest pain, while the stimulation of A2B receptors leads to peripheral vasodilation, mast cell degranulation, and bronchiolar constriction, and the stimulation of A3 leads to ischemic preconditioning and mast cell degranulation[64]. Regadenoson is an A2A receptor agonist that is a coronary vasodilator with very weak affinity for A1, A2B, and A3 receptors that are associated with adenosine’s unpleasant side effects. It is supplied in prefilled syringe doses of 0.4 mg in 5 mL of solution. It is administered as a single-dose bolus (less than 10 s), which leads to increased coronary blood flow to more than twice baseline levels within 30 s, with maximal vasodilation 1–4 min after injection, and decreases to less than twice baseline within 10 min. This is accompanied by decrease in systolic and diastolic blood pressure and increase in heart rate[65]. In the ADVANCE MPI multicenter trial comparing myocardial perfusion imaging with regadenoson compared to adenosine pharmacological stress, it was demonstrated that regadenoson was similar to adenosine for the detection of ischemia[66].
Methylxanthines block the effects of regadenoson, as for adenosine. Patients should be instructed to avoid consumption of any products containing methylxanthines, including caffeinated coffee, tea, and other caffeine-containing beverages of drug products for at least 12 h prior use. In clinical trials conducted during regadenoson’s development, where side effects were compared to adenosine, 80 % of subjects had some kind of adverse reaction, including dyspnea (28 %), headache (26 %), flushing (16 %), chest discomfort (13 %), angina or ST segment depression as evidence of myocardial ischemia (12 %), dizziness (8 %), chest pain (7 %), and nausea (6 %). In most categories, this profile was similar to side effects from adenosine, except for a lower rate of chest discomfort (13 % vs. 18 %) or evidence of ischemia (12 % vs. 18 %). There was a similar rate (26 % vs. 30 %) of rhythm or conduction abnormalities, but a lower rate of 1st-degree block (3 % vs. 7 %) or 2nd-degree AV block (0.1 % vs. 1 %). There was a higher rate of respiratory adverse reactions, such as dyspnea or wheezing compared to placebo (12.9 % in the asthma group and 19 % in the COPD patient group), but most respiratory adverse reactions resolved without therapy. Aminophylline was used 3 % of the time to treat side effects from regadenoson, versus 2 % for adenosine. As for dipyridamole and adenosine, serious ischemia, leading to myocardial infarction, ventricular arrhythmias, and cardiac arrest, has occurred following regadenoson injection [67, 68].
Dobutamine and Arbutamine
Dobutamine is a synthetic catecholamine with predominantly β1 affinity and short plasma half-life (approximately 2 min). In the presence of significant epicardial coronary artery stenosis, the increase in oxygen demand caused by positive inotropic and chronotropic effects of dobutamine can induce myocardial ischemia. Additionally, dobutamine at higher doses induces coronary vasodilation. The infusion rate used for diagnostic imaging (40–50 μg/kg/min) is higher than the customary therapeutic infusion rate of dobutamine (10–20 μg/kg/min) used for inotropic support in the intensive care units. The side effects of dobutamine in our patient series included supraventricular and ventricular arrhythmia (6 % of patients), palpitations (40 %), chest pain (20 %), shortness of breath (17 %), headache (15 %), and GI symptoms (5 %). Dobutamine was used mostly in patients who are unable to exercise and have bronchospastic disease [38], but its use has decreased since the introduction of the selective A2A vasodilator agonist regadenoson
Arbutamine is also a synthetic catecholamine. It has been marketed with a computerized feedback system between arbutamine infusion rate and the heart rate. This approach attempts to minimize the time required to reach a selected peak heart rate. However, added complexity and expense of this approach limited its widespread use [38].
15.3.2.3 Combined Pharmacological and Exercise Stress Testing
Many laboratories have found it useful to combine low-level treadmill exercise with either adenosine, regadenoson, or dipyridamole. This has been found to reduce the unpleasant side effects of flushing, headache, dizziness, or nausea due to either stressor. Image quality is also improved through a decrease in hepatic and gut uptake of the technetium-99m perfusion tracers, which is more common with adenosine or dipyridamole, compared to exercise [61, 62, 69]. On the other hand, the incidence of stress-inducible ischemia is more common due to increased myocardial demand, leading to a higher prevalence of ischemic chest pain and ischemic ECG changes. The combination of adenosine or regadenoson and symptom-limited exercise in patients who can exercise but where it is not certain that they can attain maximal heart rate has been found safe and useful to achieve maximal level of stress [63]. Combined exercise with pharmacological stress should be avoided in patients with left bundle branch block or RV pacemaker, since the likelihood of false-positive myocardial perfusion stress images is increased with exercise.
15.3.3 Methods of SPECT Imaging
15.3.3.1 SPECT Imaging
Single-photon emission computed tomography (SPECT) has by now been well standardized and optimized for either thallium-201 or Tc-99m sestamibi or Tc-99m tetrofosmin imaging as well as optimized for each imaging system manufacturer. This includes timing of acquisition, choice of collimators, choice of step-and-shoot versus contiguous acquisition, circular versus elliptical orbits, filtered versus iterative reconstruction, filtering, display, quantification, and correction for movement artifact. The greatest area of concern and therefore undergoing the greatest evolution is the challenge of addressing inaccuracies in diagnoses and inefficiencies in patient management arising from mistaking soft tissue attenuation artifacts from true perfusion defects.
Solutions to the problem of soft tissue attenuation include training in recognition by the technologist and the interpreting physician and the utilization of various compensation strategies, such as prone imaging, ECG gating, image quantification, and the use of attenuation correction hardware and corrective reconstruction.
The inspection of the original multiplanar images allows one to detect movement artifact, as well as ascertain the presence of overlapping soft tissue likely to cause attenuation artifact, either due to an overlapping diaphragm, causing inferior wall defects, or in women and obese men, overlapping breast tissue, causing anterior, anteroseptal, or anterolateral defects. This allows the interpreting physician to properly evaluate the obtained images and avoid an unnecessarily false-positive reading.
If an inferior wall defect is recognized early, while the patient is still in the laboratory, it has been demonstrated that inferior wall defects due to attenuation artifact seen in the standard supine position resolve after repeat imaging in the prone position, which improves diagnostic accuracy. Limitations of this approach include the creation of a new anterior wall attenuation defect and the apparent lack of efficacy in anterior wall attenuation artifacts [70, 71]. Nevertheless, this strategy is useful when other means are not available or in combination with gated SPECT imaging.
15.3.3.2 Gated SPECT Imaging
ECG gating of the SPECT myocardial perfusion images provides, independently from the perfusion information, important information on global LV and RV function, LV ejection fraction, and regional wall motion and thickening. This information provides information that incrementally add to the value of myocardial perfusion imaging alone. It can also help enhance the accuracy of perfusion imaging. When there is a wall motion abnormality corresponding to a perfusion abnormality, the presence of disease can be made with greater confidence, resulting in enhanced accuracy [72]. However, normal wall motion associated with a reversible perfusion defect does not exclude reversible ischemia. Normal wall motion in the presence of mild to moderate fixed perfusion abnormality could be due to non-transmural wall injury, insufficient to cause a discernible wall motion abnormality.
15.3.3.3 Quantification
Quantitative analysis of myocardial perfusion images compares the patient’s distribution at rest and during stress, after normalization to an area of the best tracer uptake, against an aggregate profile obtained from patients with known absence of disease. Software developed for this purpose provides indices of the extent and severity of perfusion abnormalities outside the normal limits, at arrest and stress, plus an index of change between stress and rest. These indices are expressed for the entire myocardium, for each of the coronary vascular territories or arbitrary segments (e.g., quadrants). These normal limits reflect the average differences between men and women, as well as a range of regional variation commonly seen in low-risk subjects. The use of this technique has been shown to enhance the accuracy of interpretation for a single reader or for readers who are less than expert [73]. The use of one of the commercially available software packages also provides standard indices that provide objective indices that can be utilized for serial studies. It also provides an index of LV cavity size, and its change with stress, which is of prognostic usefulness (see below).
Nevertheless, quantification of relative distribution of tracer at rest and stress does not express absolute uptake, thus being unable to alert for a diffusely uniform decrease in uptake during stress, indicating diffuse or extensive disease, which can be obtained only from quantitative PET imaging. In addition, quantification does not help one decide if a defect is due to disease or attenuation artifact [73, 74].
Quantification of gated SPECT images provides a global LVEF, plus indices of regional wall motion and wall thickening, useful in evaluation of function. LV dyssynchrony that occurs with primary contractile dysfunction, conduction abnormalities, and pacing can be quantified with phase analysis, adapted from its previous use in gated radionuclide blood pool imaging [75]. In this method, the first harmonic Fourier phase analysis fits a cosine function to the count variations over the gated cardiac cycle, expressing the function as an amplitude, reflecting the amount of change over time, and phase (in degrees), reflecting the onset of mechanical movement. Whereas its original use was applied in planar gated blood pool imaging, recent applications used three-dimensional SPECT gated blood pool images to overcome problems of overlap of adjacent cardiac structures and inaccurate localization of left ventricular and right ventricular abnormalities [76], which was subsequently applied to myocardial perfusion imaging [77]. This technique has been found useful in patients with advanced systolic heart failure and evidence of dyssynchrony on ECG due to conduction abnormalities, single ventricle pacing, in predicting which patients would benefit from cardiac resynchronization therapy [78, 79].
15.3.3.4 Attenuation Correction
Commercial SPECT attenuation correction systems determine the distribution of nonuniform attenuation in the chest utilizing an external source of radiation, with either external collimated radionuclide sources [80, 81] or X-ray CT offered on hybrid SPECT-CT systems [82, 83]. The former systems use collimated stationary or moving line sources of gadolinium-153 (Gd-153), which has a physical half-life of 242 days, with photon energies of 97 and 103 keV [84]. Emission and transmission information is separated with physical collimation of the radiation sources and electronic masking of the field of view of the detector during simultaneous emission-transmission imaging or, in some systems, by sequential imaging. In other systems, separation is achieved during simultaneous acquisition of emission and transmission information with either Gd-153 or, alternatively, using barium-133 (Ba-133) (physical half-life of 10.5 years, gamma energy 356 keV) [85] by energy separation.
For the external radiation source transmission scans, limitations include limited source strength and therefore additional introduced noise, crossover between emission and transmission data. Sequential emission-transmission imaging has the disadvantage of greater chance for misalignment of the two scans due to body movement or changed respiratory pattern.
Hybrid SPECT-CT systems, combining a multidetector SPECT gamma camera with a single slice, and most recently, multislice CT, developed for oncology and bone imaging, have been applied for cardiac imaging with attenuation correction. The attenuation map is provided by a low-dose noncontrast CT scan, performed after the emission scan [82, 83]. An advantage of CT transmission imaging is greater photon flux and thus less noise. A disadvantage is the production of unique artifacts from metallic objects, such as stents, pacemakers, and pacemaker and ECG monitor leads [86].
Regardless of the type of manufacturer, careful attention is required to minimize additional image noise introduced by the attenuation correction by good quality control and the use of iterative reconstruction. Care is needed to minimize and compensate for truncation and its artifacts and minimize misregistration. Misregistration can be often corrected by operator-dependent realignment of the transmission images with the emission images. Attenuation-corrected images are sensitive to artifact stemming from intense subdiaphragmatic activity close to the heart, particularly activity that is changing or in motion in the course of the acquisition [87].
In spite of inauspicious results with early attenuation correction that did not take into account scatter and depth-dependent resolution blur, attenuation correction has been shown to achieve significant improvement in the specificity of detection of coronary disease, across a wide spectrum of multiple centers with different methods of attenuation correction [88, 89]. ECG gating and attenuation correction appear to provide synergistic and complementary improvement in diagnostic accuracy [90, 91]. The improvements in specificity as a result of attenuation correction were seen in all patients, but particularly in obese individuals [92]. This has enabled the use of a single normal database for both men and women [93]. The improved detection of patients with disease and the identification of patients with normal perfusion have also facilitated the practice of stress-only imaging, thereby saving time and cost of SPECT imaging [94]. Research has suggested that a routine examination of all acquired images, spine SPECT images without and with attenuation correction, as well as prone SPECT images resulted in the fewest equivocal studies [95]
15.3.3.5 Novel Processing Software
Significant advances have been made recently in SPECT image reconstruction software. They built upon earlier advance in iterative reconstruction techniques, which were developed to achieve improved image contrast while reducing noise levels, compared with earlier standard filtered back-projection techniques. The new algorithms correct for losses in spatial resolution due to depth-dependent scatter and the line response function of the collimator, the gamma camera, and the patient, while suppressing noise in the image reconstruction process. Depending on the application need, the resolution recovery aspects may be emphasized to improve image quality, or the noise suppression aspects can be emphasized to permit either decreased imaging time or reduced radiotracer dose [96]. Sometimes termed as “wide beam” reconstruction method, it was shown that the scan time may be reduced by 50 % without compromising qualitative or quantitative imaging results [97, 98]. If the reconstruction is optimized for reduced count densities, satisfactory results were obtained even with quarter-time acquisition [99]. Both half-time and half-dose wide beam reconstructions were shown to provide myocardial perfusion SPECT image quality superior to full-time full-dose standard iterative reconstruction [100].
Another development that improves image quality is “motion-frozen” processing of gated cardiac images, which decreases blurring of perfusion images due to cardiac motion. This technique shifts counts from whole cardiac cycle into the end-diastolic position, resulting in less noisy images while enhancing resolution [96].
15.3.3.6 New Dedicated Cardiac SPECT Gamma Cameras
In the past, imaging systems were designed specifically for cardiac use, but their design differed from standard single-head or dual-head imaging gamma camera systems mainly in the smaller size of the gamma camera heads and their compact arrangement. Recently, a number of innovative new imaging systems were introduced into routine clinical use that were not only designed for cardiac use but also used novel approaches in imaging technology and design. The goals were to achieve improved spatial resolution and sensitivity, to be sure, but also to improve patient comfort by permitting imaging in an upright or reclining position and eliminating the need for positioning the patient’s arms above the head by allowing arms to be comfortably supported at shoulder level and reducing or eliminating claustrophobic effects [96]. It has been determined that compared to supine acquisition, in the upright position, anterior wall attenuation was significantly less in those imaged upright (6.1 % vs. 52 %), particularly among women. Inferior attenuation was more common among women imaged upright (50 % vs. 24 %), but was not affected among men. Lateral attenuation was more prevalent in the upright group, especially in women and those with BMI >30 [101].
Unlike conventional gamma cameras, where only a small portion of the field of view is actually used to image the heart, these new devices all have in common the concept that all available detectors image just the cardiac field of view. These designs vary in the number and type of moving (scanning) or stationary detectors and in whether sodium iodide, cesium iodide, or cadmium zinc telluride (CZT) solid-state detectors are used. They share a fivefold to tenfold gain in count sensitivity and either no loss or even a gain in resolution. This results in the ability to reduce the imaging time for a cardiac perfusion scan to 2 min or less if performed with a standard dose, or a fivefold or even tenfold decrease in injected dose, or some combination of the time/dose reduction [102].
Two of these systems use solid-state CZT detectors arranged in a pixilated array with each detector unit. The direct conversion of energy into electronic impulse provides 1.65 times superior energy resolution and thus reduced scattered photon acceptance resulting in twice and better resolution of 5 mm versus conventional 11 mm, while the pixilated array design provides localization [102].
Some of these systems come with attenuation correction using a CT transmission scan, yielding excellent clinical agreement when compared with conventional SPECT with attenuation correction [103].
This has resulted in possibilities of flexible acquisition protocols, tailored to the needs of the patient and/or constraints faced by the laboratory. This includes stress-only MPI in low-risk patients with a 2-min acquisition with a standard tracer dose to the more conventional 15-min rest/12-min stress MPI studies, simultaneous dual isotope studies, studies performed with as little as 185 MBq (5 mCi) or less [102]. Clinical studies have shown diagnostic and prognostic performance of these protocols at least as equal as that obtained with conventional gamma cameras and standard protocols [104–110]. These developments are a challenge to the cardiac imaging community to assure high quality and consistency by devising standardized protocols tailored for these new instruments.
15.3.4 Clinical Utility of Myocardial Perfusion Imaging
Initially, myocardial perfusion imaging was a primarily diagnostic method for noninvasive detection of coronary artery disease. Over the past two decades, emphasis has shifted from diagnosis to evaluation of prognosis. Analysis of extensive clinical, angiographic, and perfusion databases has identified patients who are at high risk for adverse outcome (death or nonfatal cardiac events) [111, 112]. Advances in surgical, percutaneous, and medical therapy of CAD have fundamentally changed the natural history of the disease. Thus, appropriate identification of high-risk patients, followed by appropriate therapy, favorably modifies prognosis. Conversely, the identification of low-risk patients with a benign prognosis reduces the need for costly and potentially detrimental invasive testing and therapy. A normal SPECT myocardial perfusion imaging study has been shown to be extremely effective in predicting a good prognosis in a variety of settings.
15.3.4.1 Diagnosis
Appropriate candidates for stress testing with MPI are patients with intermediate pretest probability for the presence of coronary artery disease. The pretest probability is determined from easily obtained parameters: age, gender, symptoms, and rest ECG [113]. Such patients might be chronically symptomatic with some atypical features at presentation. MPI is inappropriate for patients with a low pretest probability due to the high rate of false-positive results in such patients. Patients with several risk factors for CAD and typical symptoms with a high probability of CAD do not gain so much from MPI for diagnosis, as the diagnosis is nearly certain on clinical grounds. However, such patients would benefit from MPI for risk stratification. Exercise stress testing without MPI is inadequate for diagnostic purposes in patients who are unable to exercise adequately and in those with nondiagnostic baseline ECG, such as LBBB, paced rhythm, left ventricular hypertrophy, or users of digoxin [114]. In other patients, MPI adds to the diagnostic accuracy of ECG stress testing alone.
Average sensitivity and specificity of MPI for diagnosis of CAD have been reported close to 90 and 70 %, respectively. The gold standard for diagnosis of CAD remains coronary angiography, despite its known limitations and likely systematic underestimation of the extent of disease. True sensitivity and specificity with each new tracer and each new imaging protocol have been difficult to ascertain because of posttest angiographic referral bias. Patients with negative results on MPI are rarely referred for coronary angiography, except where clinical ECG responses or clues in the MPI images suggest possible global “balanced ischemia.” This practice is justified because of the known excellent prognosis of patients with a normal MPI study [115]. Nevertheless, this referral bias limits the usefulness of retrospective validation studies using a clinical test population.
15.3.4.2 Prognosis
Several distinct patient groups are commonly referred for MPI:
1.
Patients with stable CAD evaluated for prognosis
2.
Patients with acute chest pain syndromes
3.
Patients after an acute myocardial infarction
4.
Patients followed after revascularization procedures (CABG, PTCA, coronary stenting)
5.
Patients evaluated prior to noncardiac surgery
6.
Patients surveyed after cardiac transplantation for presence of transplant vasculopathy
Risk Stratification in Stable CAD
Recent technological advances in image acquisition and processing such as tomographic imaging (SPECT), gating, attenuation correction, and Tc-99m-based tracers allow for more accurate simultaneous evaluation of myocardial perfusion and function. Perfusion abnormalities can be classified according to size, localization, severity, and reversibility. Left ventricular volumes, systolic wall thickening, segmental wall motion, and ejection fraction can be quantified. Right ventricular size and function can be assessed.
Retrospective and prospective observations have defined patterns which are compatible with high-risk prognosis as well as benign prognosis. A normal perfusion pattern in patients with an adequate level of stress and with high-quality study is consistent with an excellent short-term prognosis, regardless of coronary anatomy [115]. The extent of perfusion abnormalities characterized by number of abnormal segments, severity of defects, and extent of reversibility (ischemia) defines prognosis. When integrated with results of the exercise stress test and parameters of left and right ventricular function, combined information has a prognostic value which exceeds prognostication based on performance of coronary angiography [112] (Fig. 15.12). The average annual cardiac event rate in patients with abnormal images is 12-fold than in patients with normal images. Both fixed and reversible defects are prognostically significant. Fixed defects are a predictor of death, whereas reversible defects are an important predictor of nonfatal myocardial infarction [116]. The event rate is significantly greater in patients with severe than in those with mild abnormalities (10.6 % annual hard event rate vs. 3.5 %) [114]. Incorporation of other SPECT variables, such as stress-induced LV dilation, LVEF, and LV volumes, further enhances the prognostic power of SPECT imaging [114, 117, 118]. A high likelihood of multivessel (hence surgical) CAD is indicated by the presence of perfusion defects in each of the three coronary artery territories, diffuse slow washout of Tl-201, prominent pulmonary Tl-201 activity, transient stress-induced LV dilation, fall in LVEF on the gated stress SPECT MPI images, and the “left main pattern” of anterior, septal, and posterolateral defects [119].
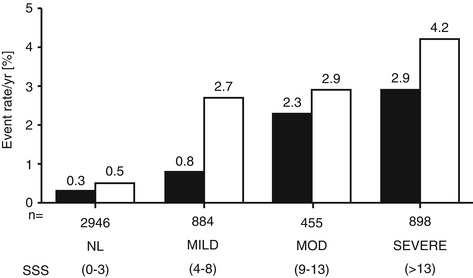
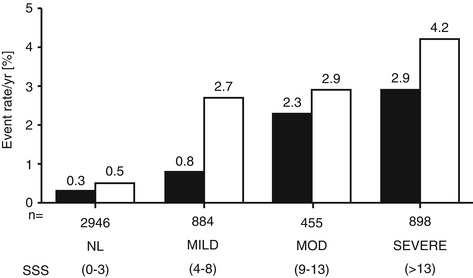
Fig. 15.12
Rates of cardiac death (solid bars) and MI (open bars) per year, as a function of scan result. The number of patients within each category is shown underneath each pair of bars. NL normal, MILD mildly abnormal, MOD moderately abnormal, SEVERE severely abnormal (Reproduced from Bockisch et al. [86] with permission)
Acute Chest Pain Syndromes
Acute chest pain may be due to myocardial ischemia as a result of a coronary artery plaque rupture and may be potentially life threatening. However, in only 40 % of emergency department (ED) visits for chest pain the pain of cardiac origin. Rapid and reliable triage is needed for speedy diagnosis of acute myocardial infarction and to prevent unnecessary hospitalizations and inappropriate discharges from the ED [120]. Current diagnostic tools include clinical observation, serial ECGs, ST segment monitoring, serial measurements of serum markers of myocardial necrosis (such as CK-MB, troponins), and noninvasive cardiac imaging. Many medical centers have recently established dedicated chest pain evaluation units, usually adjacent to the ED. Some centers perform MPI at rest. Abnormal results lead to hospital admission. Others perform stress testing, with or without MPI, 6–12 h after a negative workup for an acute MI [121, 122]. Based on the results of MPI, a patient’s short-term prognosis can be determined. Safety of early stress testing has been well documented. In several cost analyses, up to a 50 % decrease in hospital charges and a 50 % shorter hospital stay can potentially be realized [123]. In another study, a cost saving of $800 per patient was reported [124]. In still another, a cost saving of $4,000 per patient was realized if patients with normal resting myocardial perfusion images were discharged home from the emergency department [125].
MPI After an Acute Myocardial Infarction
The purposes of early or predischarge MPI evaluation after an acute myocardial infarction are (a) to assess the extent of sustained damage, including determination of the ejection fraction, and (b) to detect residual ischemia, both in the infarct-related territory and in the other vascular territories using either exercise MPI (Fig. 15.13) or pharmacological stress (Fig. 15.14). In the era of acute interventions (i.e., thrombolysis, PTCA, stenting), the urge to perform invasive assessment (by angiography) is often irresistible. However, recent reports support a less aggressive approach: Patients with a limited amount of ischemia after an acute myocardial infarction can be risk stratified noninvasively and, if found to have a low-risk profile, treated medically with the same results as those treated with interventions [128–130].
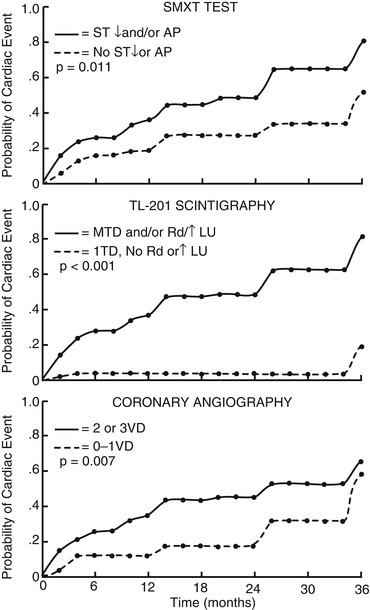
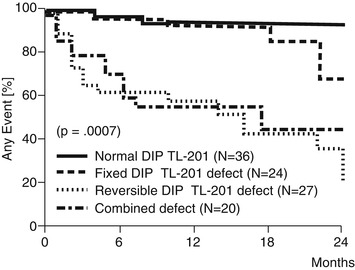
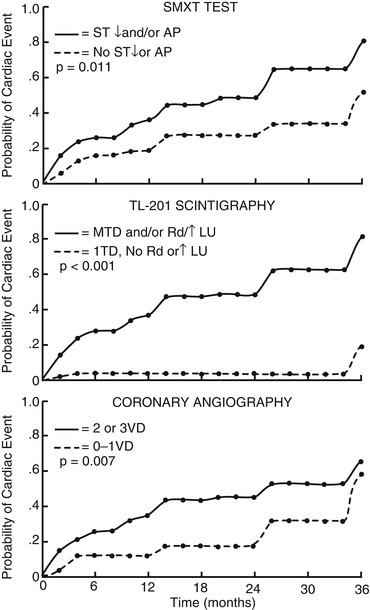
Fig. 15.13
Cumulative probability of cardiac events as a function of time for different subgroups formed by exercise test response (top), scintigraphic findings (middle), or angiographic findings (bottom) before hospital discharge following myocardial infarction. The solid and dashed lines represent the high-risk and low-risk cumulative probability, respectively (Reproduced from DePuey et al. [100] Gibson et al. [126] with permission)
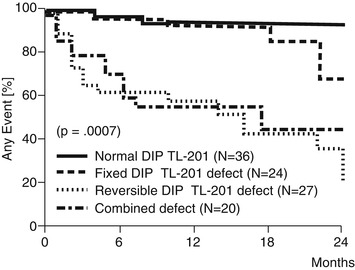
Fig. 15.14
Incidence of cardiac events (cardiac death, nonfatal myocardial infarction, unstable angina pectoris, or occurrence of functional class III or IV angina or the need for a revascularization procedure) in 107 asymptomatic patients with coronary artery disease stratified by intravenous dipyridamole (DIP) thallium (TL) results. N number of patients at entry (Reproduced from Chawla et al. [101] Younis et al. [127] with permission)
MPI in Patients After Revascularization Procedures
In view of the possibility of restenosis after percutaneous revascularization and of aortocoronary bypass graft closure after coronary artery bypass surgery, and the frequent absence of reliable symptoms, MPI is an efficient means to determine the need for additional and/or repeat interventions, especially when the clinical symptoms are vague or nonspecific [131–133].
MPI Prior to Noncardiac Surgery
Preoperative evaluation for noncardiac surgery depends partly on a patient’s risk factors. These include severity and/or stability of known heart disease; the presence of concomitant conditions such as diabetes mellitus, peripheral vascular disease, renal insufficiency, and pulmonary disease; urgency of the surgery (emergency vs. elective); and type of surgery planned. Several principles for preoperative evaluation are summarized in the 2002 AHA/ACC Task Force guideline update recommendations [134]:
1.
In case of lifesaving surgery, no cardiac evaluation is needed and it should be performed, if necessary, after the surgery.
2.
No evaluation is needed in stable patients with a history of revascularization in the past 5 years.
3.
Cancelation or delay of elective surgery should be considered for patients with unstable angina, decompensated CHF, significant arrhythmia, and/or severe valvular disease (major predictors).
4.
Patients with mild angina, history of myocardial infarction, compensated CHF, and diabetes mellitus (intermediate predictors) and those with abnormal ECG at rest, advanced age, nonsinus rhythm, low functional capacity, history of stroke, and uncontrolled arterial hypertension (mild predictors) should be triaged according to their functional capacity and according to the risk of planned surgery to noninvasive stress testing (MPI or stress echocardiography).
There is currently no evidence that preoperative revascularization alters the outcome of noncardiac surgery. However, risk stratification based on preoperative testing can help the patient and physician choose the best type and timing of surgery, perioperative care, and long-term postoperative management.
MPI After Cardiac Transplantation
Long-term survival after heart transplantation, in excess of 80 % after 1 year, is now common [135, 136]. Immunologically mediated obstructive coronary vasculopathy has emerged as the most devastating late complication. Pain symptoms of myocardial ischemia are absent because of denervation of the transplanted heart. Frequent invasive (angiographic) evaluation is not practical. MPI and perhaps stress echocardiography are emerging as surveillance methods for detection of asymptomatic myocardial ischemia [137, 138].
15.4 PET Myocardial Perfusion Imaging
In spite of its high diagnostic accuracy and prognostic value, conventional myocardial imaging suffers from limitations of attenuation artifact and spatial nonuniformity, particularly in obese patients, only partially mitigated by gated acquisition or attenuation correction [139], limited resolution, and decreased tracer extraction fraction during stress [140]. Limitation is posed in some patients by inadequate exercise level and by an occasional inadequate effect of pharmacological stress.
15.4.1 Principles of PET Imaging
PET myocardial perfusion imaging offers an alternative for patients with the above difficulties. PET imaging utilizes tracers containing isotopes which decay by the emission of positrons; these undergo annihilation together with a local electron to produce two simultaneous high-energy 511 keV photons, 180° apart. Positron emission tomography (PET) gamma cameras are able to document these events through coincidence detection. Localization is achieved electronically, rather than through collimation, greatly enhancing sensitivity. Resolution is also significantly improved (4–6 mm) compared with SPECT or planar imaging (1.5–2.5 cm). The attenuation fraction of the coincident pair of photons is high but uniform along any given path. Attenuation correction is achieved with a separate PET acquisition for each subject using an external source of coincident photons. This is done either by rotating rod sources containing germanium-68 or, more frequently now, with a CT scan contained within a hybrid PET-CT scanner. This transmission acquisition yields an attenuation map, which is used to correct the emission images.
Limitation on resolution comes from the following: (a) the mean distance that the positron travels before undergoing annihilation [140–143]; (b) the deviation from 180° of the angle between the two coincident photons, depending on the positron momentum at the time of annihilation, producing an error of about 1.7 mm [144]; (c) the proportion of coincident photons which undergo scatter; (d) random coincidences [145, 146]; (e) the noise of acquisition and that introduced by the attenuation correction; and (f) the intrinsic resolution limit of the gamma camera itself. Modern PET cameras achieve an intrinsic resolution of 3–5 mm [147].
15.4.2 Cardiac PET Perfusion Tracers
The most common PET myocardial perfusion tracers include N-13 ammonia, rubidium-82, F-18 flurpiridaz, and O-15 water (Table 15.4). Only rubidium-82 and N-13 ammonia are approved for clinical use. Both N-13 ammonia and O-15 water require an on-site or near-vicinity cyclotron and a chemistry laboratory equipped to produce the tracer. Excellent timing between production and patient use is required, due to their short half-lives. Rubidium-82 is produced on-site by a commercially available generator consisting of an alumina column containing strontium-82, which decays to rubidium-82, which is eluted with saline. Its availability is reestablished within 6–8 min after each use. The generator is changed every 4 weeks. However, N-13 ammonia use is limited by the necessity of being located close to a cyclotron-radiopharmacy unit, while the use of rubidium-82 is limited by the high fixed cost of the generator. F-18 flurpiridaz is currently evaluated in post-Phase III trials, and awaiting US FDA approval, as an alternative, being, like F-18 FDG with a sufficiently long physical half-life to be produced in regional cyclotron radiopharmacy and distributed through the existing commercial radiotracer supply network.
Table 15.4
Positron-emitting tracers of myocardial blood flow
Agent | Physical half-life | Mean positron range (mm) | Production |
---|---|---|---|
N-13 ammonia | 9.8 min | 0.7 | Cyclotron |
Rubidium-82 | 75 s | 2.4 | Generator |
F-18 flurpiridaz | 110 min | 0.2 | Cyclotron |
O-15 water | 2.0 min | 1.1 | Cyclotron |
The imaging session begins with a short scout perfusion acquisition or a scout transmission CT scan, in order to position the patient within the scanner properly. The perfusion tracer is injected intravenously at rest, followed by a PET acquisition, and again during pharmacological stress with either intravenous regadenoson, dipyridamole, adenosine, or dobutamine/arbutamine. The rest and stress perfusion studies can and should be gated, if possible, providing valuable information about LV function at rest and during stress. With a PET-CT scanner, a CT transmission scan with each of the rest and stress perfusion scans is performed. In the case of a dedicated PET scanner, a pin-source transmission scan is usually performed between the rest and the stress perfusion scan. For N-13 ammonia or F-18 flurpiridaz, it is possible to perform either exercise or pharmacological stress, although pharmacological stress is been used in most patients. It is possible to perform supine bicycle exercise on the imaging table, but this has been the exception. The half-lives of Rb-82 and O-15 are too short to allow imaging with exercise on a treadmill. On the other hand, the short half-lives allow a quick succession of resting and stress acquisition and multiple interventions, including vasodilation, handgrip, hand ice immersion, or mental effort [162].
15.4.3 N-13 Ammonia
In the bloodstream, N-13 ammonia consists of the neutral NH3 molecule in equilibrium with NH4 +. At normal pH, NH4 + is the predominant form. The neutral, lipid-soluble NH3 readily crosses cell membranes by diffusion. Inside the cell, the NH3 converts into NH4 + and is trapped in the cell as glutamine in a reaction catalyzed by glutamine synthase [151]. Egress from the cell is slow, mostly through catabolism of proteins and amino acids. N-13 ammonia has been used as a PET myocardial perfusion agent since 1972 [163] with either pharmacological or exercise stress. Its extraction fraction remains high even with high flows during pharmacological vasodilation, although under severe metabolic derangement, the glutamine synthase pathway can be blocked and the uptake of N-13 ammonia can become low [164]. Its half-life allows high-quality image acquisition and gating. In dogs, Gould et al. demonstrated that coronary stenoses of 47 % or greater can be detected by perfusion imaging with N-13 ammonia in conjunction with IV dipyridamole [165]. In human beings, Schelbert et al. correctly identified 52 of 58 stenosed vessels (90 % sensitivity per vessel) and correctly diagnosed the presence of CAD in 31 of 32 patients (97 % sensitivity) [151]. Tamaki et al. [166] demonstrated a sensitivity of 95 % for N-13 ammonia rest and exercise stress imaging (Table 15.5) (Fig. 15.15).
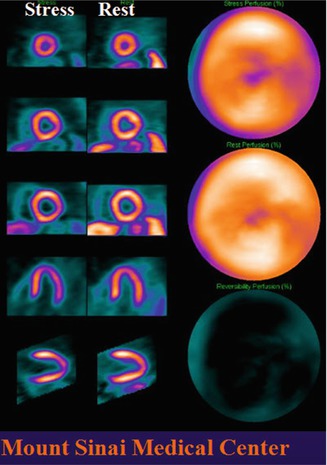
Reference | Agent | Stress | No. of subjects | Sensitivity (%) | Specificity (%) |
---|---|---|---|---|---|
CAD subjects | |||||
Tamaki et al. [149] | NH3 | Exercise | 19 | 95 | 95 |
Allan et al. [150] | Rb-82 | Exercise | 25 | – | 96 |
Schelbert et al. [151] | NH3 | Dipyridamole | 32 | 90 | 97 |
Gould et al. [152] | NH3, Rb-82 Dipyridamole | 50 | – | 95 | |
Samson et al. [153] | Rb-82 | Pharma | 64 | 93 | 50 |
Santana et al. [154] | Rb-82 | Adenosine | 53 | 93 | 75 |
Stewart et al. [155] | Rb-82 | Dipyridamole | 81 | 85 | 90 |
Yonekura et al. [156] | NH3 | Exercise | 38 | 89 | 90 |
Go et al. [157] | Rb-82 | Dipyridamole | 202 | 93 | 78 |
Bateman et al. [158] | Rb-82 | Dipyridamole | 112 | 89 | 90 |
Nandalur et al. [159] | NH3, Rb-82 Ex, Pharma | 1,442 | 92 | 85 | |
Berman et al. [160] | F-18 flurpiridaz Ex, Pharma 143 | 79 | 76.5 | ||
Normal subjects | |||||
Tamaki et al. [149] | NH3 | Exercise | 6 | – | 100 |
Deanfield et al. [161] | Rb-82 | Exercise | 16 | – | 100 |
Schelbert et al. [151] | NH3 | Dipyridamole | 13 | – | 100 |
Berman et al. [160] | F-18 flurpiridaz Ex, Pharma 39 | – | 90 |
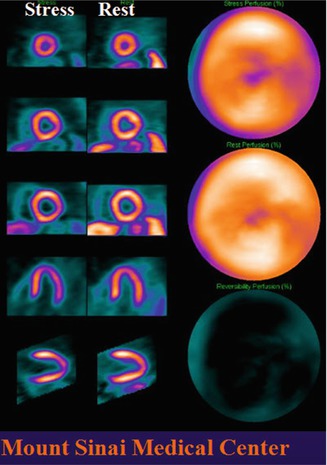
Fig. 15.15
Stress and rest N-13 ammonia PET images demonstrating a mild lateral wall defect during stress, with normal distribution at rest
15.4.3.1 Rubidium-82
Rubidium-82 is a potassium analog. Like Tl-201, Rb-82 is transported into cells by the Na+/K+ ATPase pump. Like Tl-201, Rb-82 extraction decreases at high blood flow [167, 168] and can be altered by drugs, severe acidosis, hypoxia, and ischemia [169–171]. With ischemia, segmental reduction of Rb-82 uptake can persist following exercise, even after symptoms and ECG abnormalities have resolved, for up to 30 min. Owing to its short half-life (75 s), Rb-82 is injected at a high dose (30–60 mCi); this is followed by a short acquisition lasting 4–6 min. The imaging sequence can be fast and efficient. An example of rest and stress Rb-82 PET myocardial perfusion images and gated resting images is shown in Fig 15.16. In chronically instrumented dogs, Gould et al. detected coronary stenoses of 50 % or greater with Rb-82 imaging and dipyridamole stress [172]. Gould et al. [152] compared Rb-82 rest and dipyridamole-handgrip stress imaging with a validated quantitative flow reserve index obtained from contrast angiograms. The results showed a sensitivity of 95 % and a specificity of 100 % for impaired flow reserve. As shown in Table 15.5, studies performed to study the accuracy of Rb-82 PET perfusion MPI for the detection of CAD showed a sensitivity from 85 to 93 % and a specificity of 50–90 %
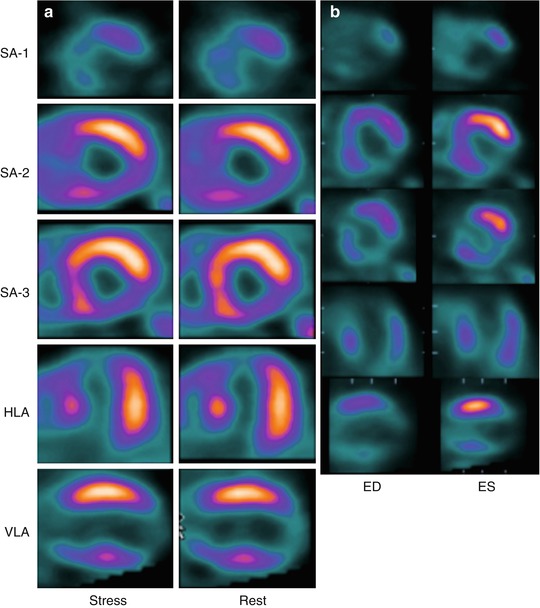
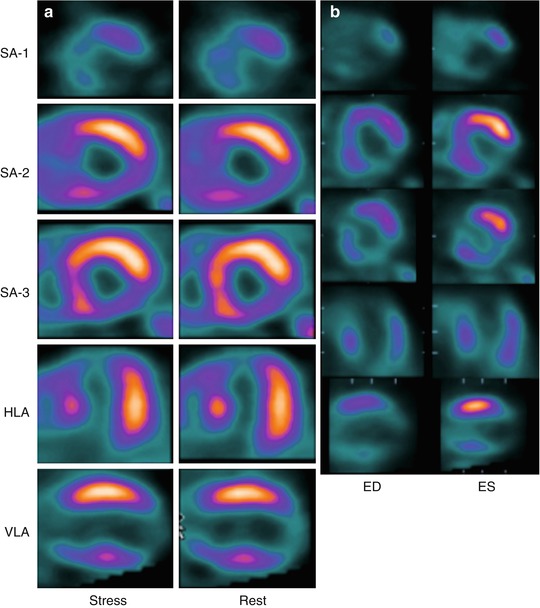
Fig. 15.16
(a) Stress and rest of Rb-82 PET images demonstrating severe extensive apical, septal, and inferior scarring and only minimal basal septal ischemia. (b) Resting end-diastolic and end-systolic gated images, showing poor or absent contractility in the scarred regions and poor overall LV function
The rubidium-82 generator needs to be replaced 13 times per year. Its advantage is 24-h availability in PET centers that do not have a cyclotron or where the cyclotron is preoccupied with production of other isotopes. The disadvantage of Rb-82 is the high fixed cost of the generator, which rivals the yearly costs of a PET camera or cyclotron. It is thus feasible only in centers with a high volume of cardiac PET perfusion imaging. Generally, centers that have an on-site cyclotron perform perfusion imaging with N-13 ammonia, due to the already built-in overhead costs of the cyclotron. Due to the high energy of the positron emitted by Rb-82, the mean travel of the positron is higher compared with the other positron-emitting isotopes, making resolution slightly worse than for F-18 flurpiridaz or N-13 ammonia. Due to the short half-life of Rb-82, the images tend to be noisier, especially on older PET instruments with lower sensitivity. This disadvantage is mitigated with the higher sensitivity of 3D PET imaging, but which has to contend with dead time and high rate of random coincidences and scatter.
15.4.3.2 Fluorine-18 Flurpiridaz
F-18 flurpiridaz is lipophilic and, like Tc-99m sestamibi, binds to mitochondria with high affinity. Preclinical studies showed that the extraction fraction of F-18 flurpiridaz was greater than that of N-13 ammonia, R-82, thallium-201 (201Tl), or technetium-99m (Tc-99m) sestamibi and was in fact found to be greater than 90 %. Both sensitivity and absolute quantification of MBF would be expected to be more suitable with this high-extraction-fraction tracer because there is significantly less roll-off in extraction at high flows than there is with other tracers [173]. In Phase I trials, the heart exhibited high and sustained retention of F-18 flurpiridaz from the earliest images through approximately 5 h after injection [174]. Similar to the Tc-99m perfusion agents, one administers a slightly lower for resting study dose (approximately 74–111 MBq (2–3 mCi)). After waiting for approximately 50–70 min, perform exercise or pharmacological stress, and administer a higher (approximately 240 MBq (6.5 mCi)) during stress for stress study. In a Phase II study comparing F-18 flurpiridaz MPI to Tc-99m sestamibi SPECT MPI in the same 143 subjects in 21 centers, a higher percentage of images was rated as excellent or good on PET versus SPECT imaging, with a higher diagnostic certainty. In 86 patients who underwent coronary angiography, sensitivity of F-18 flurpiridaz PET for individual coronary vessel disease was higher than SPECT MPI, 79 % versus 62 %, with similar specificity, and a normalcy of 90 % with F-18 flurpiridaz PET versus 97 % with SPECT. In patients with CAD on angiography, the magnitude of reversible defects was greater with PET compared to SPECT [160].
15.4.3.3 Oxygen-15 Water
The use of O-15 water is limited to quantification of coronary blood flow. Water enters all cells by diffusion, with a high extraction fraction even at high myocardial flow. Owing to the very short half-life of O-15, the tracer must be produced by an on-site cyclotron. The images tend to be noisy, and the rapid equilibration between blood pool and the myocardium prevents good quality imaging of the myocardial phase. Routine clinical use could tie up a cyclotron just for this indication in a large number of patients. Therefore, for combined clinical use and quantification of blood flow, N-13 ammonia has been used more extensively in centers equipped with a cyclotron.
15.4.4 Applications of Cardiac PET Perfusion Imaging
PET myocardial perfusion imaging, when performed properly, offers reliable attenuation correction, thus avoiding the attenuation artifacts seen frequently in SPECT imaging, although caution needs to be exercised to recognize and correct for PET emission-transmission misregistration artifacts. PET imaging also offers higher resolution, although if used without gating, the higher resolution is not fully taken advantage of. The high energy of the positrons from Rb-82 also prevents a full utilization of the inherent high resolution of PET. Because clinically useful risk stratification needs to detect significant myocardial mass with disease risk, spatial resolution is not critical in deciding to use PET versus SPECT imaging. The higher resolution is definitely helpful in children with anomalies of the coronary arteries or after repair procedures, such as the switch operation [175, 176]. The high extraction fraction of the PET tracers, especially N-13 ammonia and O-15 water, assures higher sensitivity for disease at high flows during pharmacological stress, resulting in the ability to reliably detect coronary disease down to about 50 % occlusion [177, 178] (Table 15.5). A number of studies have shown a higher accuracy of coronary disease detection by stress PET imaging compared with SPECT Tl-201 imaging [151, 175–177] (Table 15.6). Comparisons of sestamibi and PET perfusion imaging showed that adenosine stress MIBI SPECT imaging significantly underestimates ischemia and defect severity compared with N-13 ammonia PET [179]. More recent studies indicate that the most up-to-date PET imaging achieves both higher sensitivity and specificity for detection of coronary artery disease compared to gated Tc-99m sestamibi SPECT imaging, both with [180] and without SPECT attenuation correction[158]. Figure 15.17 illustrates such a case. The gating of MPI at rest and during stress with PET imaging allows the additional diagnostic and prognostic relevant information about the severity of disease. During gated vasodilator stress Rb-82 PET imaging, LVEF increases with vasodilator stress in patients without significant stress-induced perfusion defects or severe left main/3-vessel CAD. A high LVEF reserve appears to be an excellent tool to exclude left main/3-vessel CAD noninvasively [181] (Fig. 15.18).
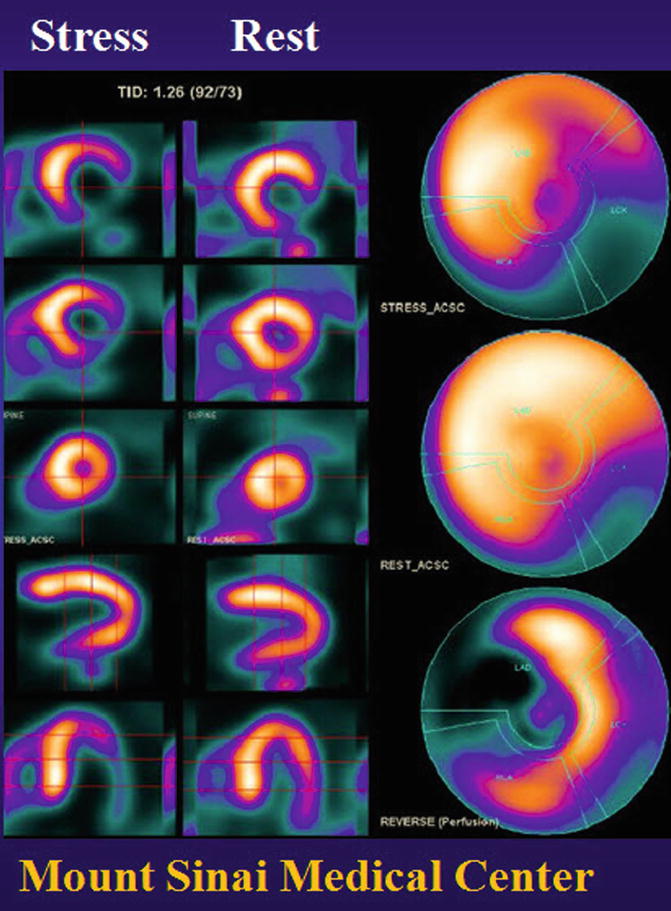
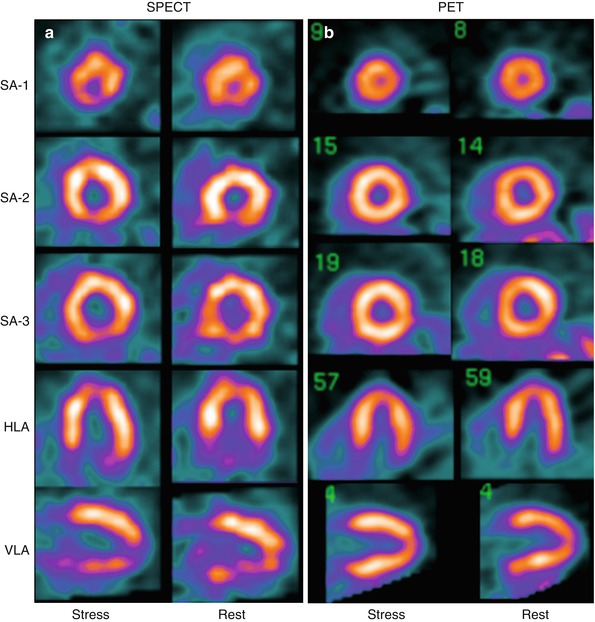
Table 15.6
Comparison of PET and conventional perfusion imaging for CAD detection
Reference | Tracer | Accuracy (%) | Sensitivity (%) | Specificity (%) |
---|---|---|---|---|
Schelbert et al. [151] | NH3 | 90 | ||
Tl-201 | 58 | |||
Go et al. [157] | Rb-82 | 92 | 95 | 82 |
Tl-201 | 78 | 79 | 76 | |
Stewart et al. [155] | Rb-82 | 84 | 88 | 85 |
Tl-201 | 84 | 53 | 79 | |
Bateman et al. [158] | Rb-82 | 89 | 87 | 93 |
Tc-99m MIBI | 79 | 82 | 73 | |
Berman et al. [160] | F-18 flurpiridaz | 79 | 76 | |
Tc-99m SPECT | 62 | 74 |
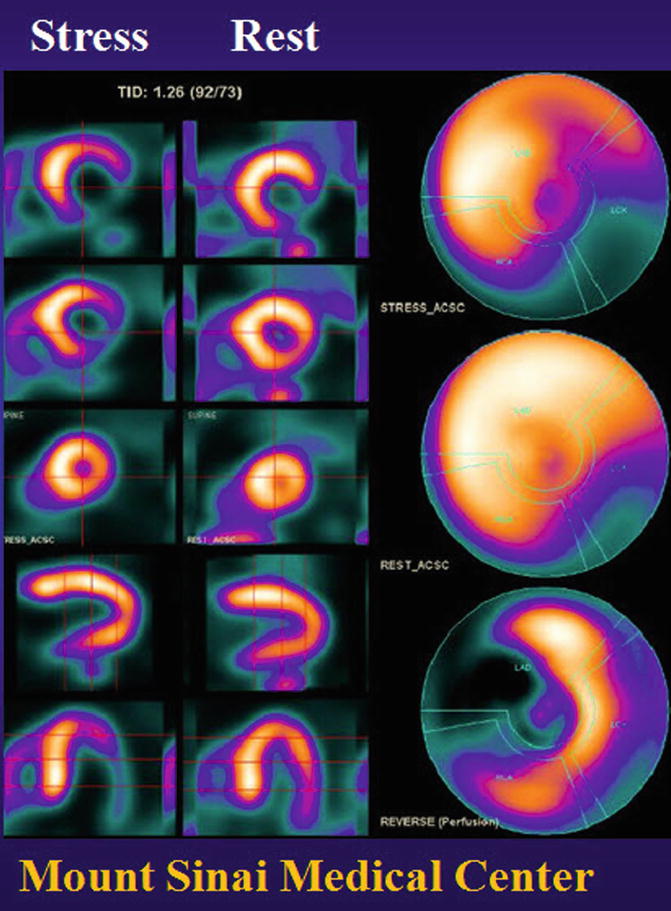
Fig. 15.17
Stress and rest PET images with F-18 flurpiridaz, demonstrating severe inferolateral and mild anterolateral and apicolateral defects with stress, with complete improvement in the anterolateral and apicolateral wall and partial improvement in the inferolateral wall
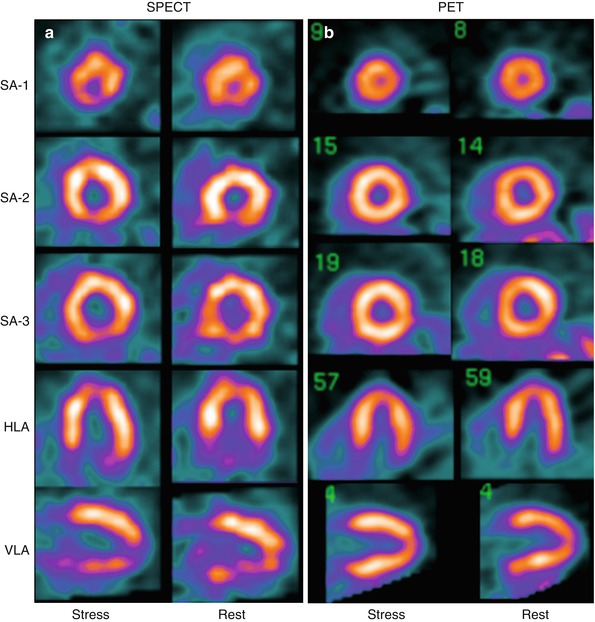
Fig. 15.18
(a) Stress and rest attenuation-corrected (AC) Tc-99m sestamibi SPECT images of a 290-lb, 51-year-old male. The SPECT image shows mild to moderate inferoapical ischemia and partial scarring. (b) The stress and rest PET images show normal distribution
Positron emission tomography for myocardial perfusion is an expensive option, due to the high cost of a PET or PET-CT camera ($1–2 million) and of either cyclotron-produced isotopes or the Rb-82 generator. Patterson et al. [182] have modeled the clinical use of Rb-82 perfusion imaging, stress ECG, Tl-201 SPECT perfusion imaging, and coronary angiography in the diagnosis of CAD. Using published data for accuracy and costs of procedures, assumptions of some cost savings from making the right diagnosis, and costs incurred when the diagnosis is missed, they found PET perfusion imaging to be more cost-effective (lowest cost/deltaQALY) than the other modalities in populations with a low to intermediate probability of CAD. Savings were realized through reductions of costs of missing disease and reduction in costs from unnecessary additional diagnostic and therapeutic procedures. A preliminary study came to the same conclusions when comparing PET myocardial perfusion imaging with stress echocardiography and coronary angiography [183]. This model was verified by clinical cost-effectiveness in 2,159 patients studied with PET MPI with intermediate risk of CAD compared with 102 internal group of patients and an external group of 5,826 patients studied with SPECT MPI, with a 30 % reduction in management costs due to the reduced rate of angiography and CABG surgery and yet no significant difference in cardiac death or myocardial infarction at 1 year follow-up [184]. PET myocardial perfusion imaging has been approved by the CME for reimbursement by Medicare and is reimbursed by most insurance plans. From a more conservative point of view, PET perfusion stress imaging is justified in cases where conventional methods are inconclusive or conflicting or where technical artifacts, e.g., obesity or breast attenuation artifact, are likely to limit the accuracy of conventional imaging.
The ready availability of Rb-82 from a generator allows the PET scanner to be used in patients with acute chest pain [122, 185–187] where logistics and location permit. Goldstein et al. studied 14 patients with Rb-82 within 96 h of onset of symptoms. Positron emission tomography correctly identified segmental blood flow reduction in all instances of myocardial infarction [188]. PET imaging has been shown to be more reliable than ECGs in distinguishing transmural from nontransmural infarction and in the localization of infarction [189].
PET MPI, similarly to SPECT MPI, has great prognostic value, although the literature with PET imaging is less well developed. Marvick et al. noted that defect severity with PET was related to outcome [190]. Work by Yoshinaga et al. supports these findings [191]. Recent work by Chow et al. [192] indicates that patients with normal ribidium-82 PET MPI have good prognosis, regardless of ECG changes during stress. Work by Nemirovsky et al. supports these findings [193]. A study by Yoshinaga et al. [194] obtained a mean follow-up of 3.1 years in 367 patients studied with dipyridamole Rb-82 PET MPI and showed a significant prognostic value of PET MPI for predicting cardiac events and death. They observed significant prognostic value in patients whose diagnosis was uncertain after SPECT MPI and in obese patients. A multicenter observational registry in 7,061 patients from 4 centers underwent a clinical indicated rest-stress Rb-82 PET MPI, with a mean follow-up of 2.2 years. The investigators found that the risk-adjusted hazard of cardiac death increased with 10 % mildly, moderately, or severely abnormal stress PET, compared to normal PET results. The model worked even better when clinical risk factors were combined with PET MPI findings [195]. In a related work, Rb-82 PET findings were particularly helpful in identifying high-risk, older women [196]. Similarly, perfusion findings on 256 N-13 ammonia PET MPI studies were shown to be strong predictors of clinical outcome [197]. Dorbala et al. [198] demonstrated that not only vasodilator stress Rb-82 PET MPI provides incremental prognostic value to historical/clinical variables to predict risk of cardiac events and all-cause death, but also the left ventricular ejection fraction reserve provides significant independent and incremental value to Rb-82 MPI for predicting the risk of future adverse events.
From the beginning, PET perfusion rest and stress imaging has shown its value in basic and clinical research endeavors, based on its ability to measure absolute myocardial perfusion. Conventional methods of expressing blood flow reduction pose significant difficulties. Regional blood flow reduction is usually expressed as a ratio of abnormal to maximal uptake, without consideration of the nonlinearity of the relation of the blood flow response and uptake of all diffusible tracers (Fig. 15.10). The same ratio may describe widely different relations in flow reductions.
The high degree of uniformity through attenuation correction in PEt allows calibration of the PET imaging system, so that the absolute activity in the myocardium can be measured. Unlike routine myocardial perfusion imaging, which requires only a single “snapshot” of tracer distribution at rest and again at stress, quantification of blood flow requires a dynamic acquisition of both blood pool and myocardial activity. Combined with a suitable compartmental model and a method of measuring or estimating the concentration of tracer in arterial blood, myocardial perfusion can be regionally quantified with N-13 ammonia, Rb-82, F-18 flurpiridaz, or O-15 water [150, 199–202]. Using beta-probes and an open-chest dog model, Mullani et al. validated their method of flow quantification using first-pass bolus imaging of Rb-82 [170, 203, 204]. This was also validated using PET imaging with either N-13 ammonia or Rb-92 and a compartmental model in dogs. Compared with coronary flow and flow reserve measurement using coronary electromagnetic flow probes, Yoshida et al. obtained correlation coefficients of 0.94 for N-13 ammonia and 0.88 for Rb-82. A simplified, more practical imaging protocol yielded a correlation of 0.98 and 0.94 between the simplified model and the complete compartmental model for Rb-82 and N-13 ammonia, respectively [205]. More recently, a similar simple model approach was found to be accurate with flow measurement with F-18 flurpiridaz [206].
CFR was found to independently augment clinical outcome prediction in addition to perfusion findings in N13-ammonia PET MPI [197]. The quantification of blood flow at rest and during maximal pharmacological stress allows measurement of flow reserve in various hypertrophies and cardiomyopathies, posttransplant CAD [207], syndrome X, and other vascular endothelial disorders and to study the effects of smoking, diabetes, various medications [208–210], and lipid control [211–214].
Ultimately, utilization of PET for perfusion imaging will be determined by a combination of complex factors such as clinical needs, availability and cost of PET gamma cameras and tracers, and reimbursement for outpatients and inpatients. By this time, the availability of PET cameras has become ubiquitous, fueled by the acceptance of PET imaging in clinical oncological applications and making its availability for myocardial imaging, at least on a part-time basis, easier than in the past. It is for these reasons that PET MPI has witnessed rapid growth in the past decade. This is counteracted by strong pressures to reduce costs of health care by the restrictions on the use of all diagnostic procedures and, when these are necessary, by the use of the least expensive among them, such as simple exercise, ECG stress testing, ECHO stress testing, and SPECT MPI, as opposed to PET MPI.
15.5 Hybrid Myocardial Perfusion and CT Imaging
15.5.1 CT Attenuation Correction
Combining PET and CT imaging as a single combined PET-CT unit has become the preferred approach for PET imaging in oncology. For cardiac PET imaging, the scout CT checks the position of the patient in a few seconds. The CT transmission scan, lasting 10–30 s, saves a significant amount of time compared to transmission imaging using radiation pin-sources. The CT transmission scan is relatively noise free, compared to the dedicated PET transmission scan. It enables one to perform an entire rest and pharmacological stress PET perfusion imaging study with rubidium-82 in 30–40 min, compared to 45–60 min for a dedicated PET scanner.
PET-CT imaging holds both challenges and solution for attenuation correction. There is potential for transmission-emission scan misregistration, particularly for the stress perfusion study, which is susceptible to changes in heart and diaphragm position due to changes in respiratory pattern during stress. This occurs about 10 % of the time, with a potential significant impact of about 5 % of patient studies [215]. The CT transmission and PET emission images can be easily displayed using existing software. It has become standard practice in most laboratories to perform a separate CT transmission scan at rest and during stress.
The lower-energy high-resolution (80–120 keV) X-rays of the CT attenuation correction scan are also more susceptible to artifacts produced by metallic implants or pacemakers than the 511 keV lower-resolution gamma photons of the pin-source transmission scans. The shorter amount of time (10–30 s) taken by the CT transmission scans makes it more vulnerable to diaphragm motion artifact, compared to the much longer time required for the pin-source transmission scan, which averages the heart and diaphragm motion over several minutes.
Hybrid SPECT-CT imaging system was originally designed for oncology and bone imaging. Following a SPECT emission acquisition, a CT transmission scan can be obtained, which is less noisy than radioactive-source transmission scans. In a study using chest phantoms, O’Conner et al. found that the imaging system with SPECT attenuation correction with a CT transmission scan produced more accurate attenuation correction than other systems with either fixed or moving radioactive line sources [216]. On the other hand, sequential emission-transmission imaging is more susceptible to misregistration artifact. Other manufacturers have more recently developed SPECT-CT hybrid systems with higher-quality 2–64-slice CT scanners. These multislice CTs are capable of more than just attenuation correction.
15.5.2 Calcium Scoring
Another application of PET-CT and SPECT-CT is the possibility of obtaining coronary calcium scores in the same imaging session as the PET or SPECT MPI, which is feasible with an 8-, 16-, or 64-slice multidetector CT scanner. Calcium scoring requires gating and a higher current from the CT scanner than a transmission scan, resulting in higher patient radiation exposure, but still lower than diagnostic CT imaging.
The clinical value of coronary calcium scoring is at this time still an open question in clinical practice. Shaw et al. showed in a large observational data series that coronary calcium scoring provides independent incremental information in addition to traditional risk factors in the prediction of all-cause mortality [217]. Berman et al. [218] and Kim et al. [219] showed that while there is a general relationship between the severity of coronary calcifications and the prevalence and severity of myocardial perfusion abnormalities with SPECT or PET imaging, respectively, there were a substantial proportion of patients with no coronary calcifications but abnormal myocardial perfusion findings and normal myocardial perfusion even in the presence of very high coronary calcium scores. Thus, regional coronary disease and calcium deposition provide different, even if complementary, information. In patients with risk factors but few symptoms being screened for CAD, calcium scoring can add specificity when the calcium score is low and the perfusion results are equivocal or abnormal due to endothelial dysfunction. The calcium score can add sensitivity in the detection of preclinical CAD, even in the presence of a normal MPI. Importantly, a stepwise increase occurs in the risk of adverse events with increasing CAC scores in patients with and without ischemia on PET myocardial perfusion imaging [220]. The optimal selection of patients for the acquisition of myocardial perfusion and calcium scoring in the same session remains to be determined.
15.5.3 CT Coronary Angiography
An intriguing possibility is the potential value of CT coronary angiography performed together with PET or SPECT MPI in selected patients. Multislice (16 or greater) CT scans have been found to have sufficient temporal resolution to image, with intravenous contrast, coronary arteries with a diameter of 1.5 or greater with 16-slice CT with a sensitivity of 86–92 %, a specificity of 93–99 %, and an accuracy of 93 % and an even better performance in smaller vessels with 32- or 64-slice CTs [221, 222]. There are limitations in visualizing lesions in the smallest distal vessels and in the presence of heavy calcifications. The latter limitations can be overcome with the aid of MPI [223]. Combined CTA and radionuclide MPI was shown to provide improved diagnostic accuracy for the noninvasive detection of CAD [224]. A meta-analysis of 11 eligible articles including 7,335 patients with suspected CAD revealed that the presence of one or more significant coronary stenoses was associated with an annualized event rate of 11.9 and 6.4 % if revascularizations were excluded [225]. Choudhary et al. showed that a stepwise approach, with high clinical risk, in spite of normal MPI, history, CAC, and CTA can identify about 50 % of the patients with normal MPI who have a higher risk and may benefit from aggressive medical management [226].
Nonetheless, the use of coronary CTA in nonacute setting among US Medicare beneficiaries was more likely to undergo subsequent invasive cardiac procedures and has higher CAD-related spending than patients who underwent stress testing [227]. This was supported by the results from the SPARC study, which assessed 90-day posttest rates for catheterization and medication changes in a prospective registry of 1,703 patients without a documented history of CAD and an intermediate to high likelihood of CAD, undergoing SPECT MPI, or PET MPI, or 64-slice coronary CTA. Overall, noninvasive testing had only a modest impact on clinical management of patients, with an apparent medical and invasive therapy undertreatment in patient with test abnormalities of higher risk. Patients were more likely to undergo cardiac catheterization after coronary CTA than after SPECT or PET MPI after normal/nonobstructive and mildly abnormal study findings [228].
It is conceivable that patients with known or suspected disease could be effectively studied with sequential stress-rest perfusion and functional imaging and if clinically indicated by careful selection, CT angiography, allowing the acquisition of superimposable images of coronary artery anatomy, perfusion, wall motion, and viability [229]. This complete set of spatially mapped information could add precision and ease to decision making for interventions in multivessel disease or in patients with physiologically abnormal perfusion but anatomically normal coronary arteries. This proposition needs to be tested in clinical studies.
15.6 Infarct-Avid Imaging
15.6.1 Introduction
The diagnosis of myocardial infarction is made on the basis of clinical history, the ECG, and myocardial enzymes. In the 1970s and earlier, there was a role for myocardial infarct imaging, due to lack of sufficiently sensitive and specific diagnostic methods. The introduction of specific CK-MB myocardial enzyme assays and assays for myoglobin and troponin has markedly improved clinical diagnosis of acute infarction [230].
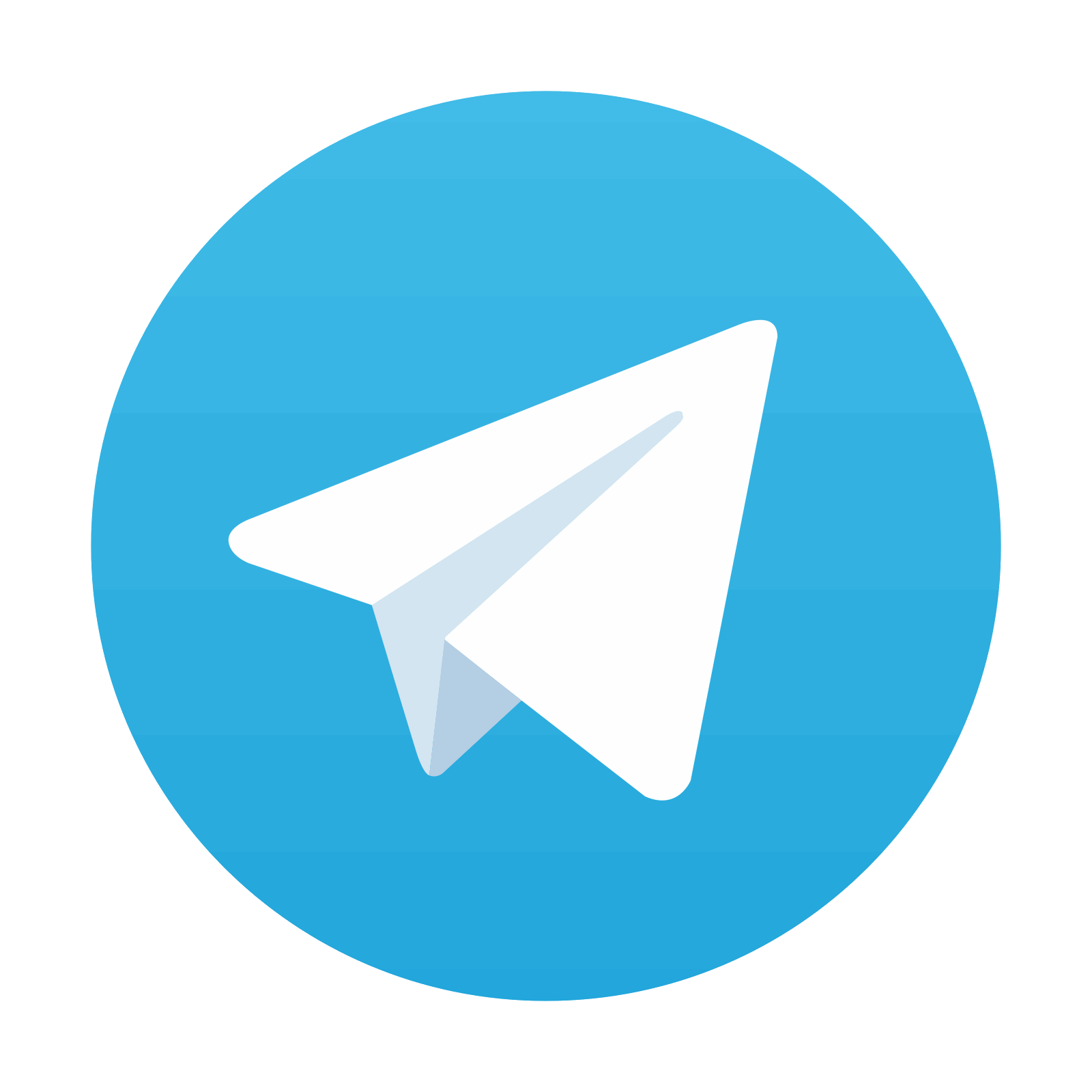
Stay updated, free articles. Join our Telegram channel

Full access? Get Clinical Tree
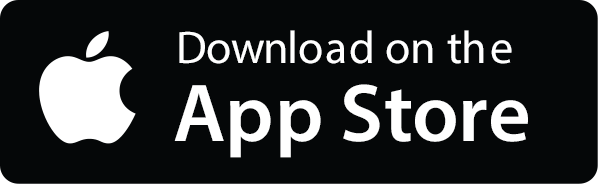
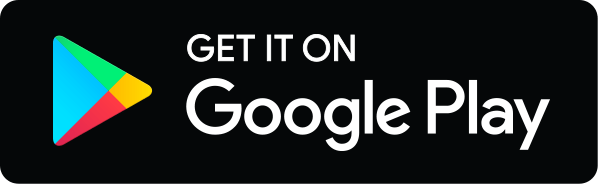