Reference and year of publication
Study design
Number of patients
IMRT dose/fraction size, Gy
Chemotherapy
% with resected disease
Median progression-free survival time, months
Median overall survival time, months
Neoadjuvant chemoradiation therapy
Pipas et al. [51]
Phase II
37 (12 % resectable, 70 % borderline resectable, 18 % unresectable)
54/2.0 with ENI
Before RT: cetuximab Concurrently: cetuximab gemcitabine
76 % (R0 from total resected: 92 %)
24.3
Patel et al. 2011 [52]
Retrospective
17 (100 % borderline resectable)
45–50/1.8–2.0 without ENI
Before RT: gemcitabine docetaxel capecitabine Concurrently: fluorouracil
52 % (R0 from total resected: 89 %)
10.5
15.6
Adjuvant chemoradiation therapy
Herman et al. [53]
Phase II
48 (40 negative margins, 8 positive margins)
50.4/1.8
Concurrently: erlotinib capecitabine After RT: gemcitabine erlotinib
15.6
24.4
Abelson et al. [54]
Retrospective
29 (22 negative margins, 7 positive margins)
50.4/1.8
Concurrently: fluorouracil
N/A
20.4
Rates at 1 year = 79 %
2 years = 40 %
17.2.3 IMRT Techniques
17.2.3.1 Treatment Volume
The GTV generally comprises the primary tumor and any grossly involved lymph nodes (minimum diameter >1 cm) based on computed tomography (CT), magnetic resonance imaging (MRI), and (optionally) 18F-fluorodeoxyglucose positron emission tomography (PET) [57]. The clinical target volume (CTV) is the area thought to harbor micrometastasis and is usually covered by a 0.5–1.5-cm margin around the GTV. The need for ENI for unresectable or neoadjuvant/borderline resectable cases is controversial, but ENI is typically used even though its benefits have not been proven in a planned prospective randomized study. Findings from some recent trials suggest that omitting ENI can both be effective and allow dose escalation of radiation or chemotherapeutic agents [45, 58, 59]. However, if the dose constraints for normal tissues permit, the retropancreatic space between the root of the celiac and superior mesenteric arteries could be reasonably included in the CTV, because microinvasion of the neural plexus in this region is common and causes severe back pain that reduces the patients’ quality of life. When IMRT is used for adjuvant therapy, the CTV is the preoperative tumor bed and the region at highest risk for residual subclinical disease. Clinical studies have also involved targeting the following volumes as specific regions of interest (ROIs), according to the guidelines used to construct the 3D fields used in RTOG 9704: the celiac artery (the most proximal 1.0–1.5 cm of that artery from where it splits from the aorta and including up to its first branching), the superior mesenteric artery (the most proximal 2.5–3.0 cm of that artery from where it splits from the aorta), the portal vein, the site of pancreaticojejunostomy (may be omitted if clinically appropriate), and the aorta (from the cephalad contour of the celiac artery, and portal vein or pancreaticojejunostomy site [whichever is most cephalad] to the bottom of the L2 vertebral body). The PTV is the CTV plus a setup margin and the internal target volume (ITV). ITV is generated via management of respiratory motion, as described below .
17.2.3.2 Respiratory Motion Management
The delivery of highly conformal radiotherapy is usually problematic because the pancreas moves with respiration. According to fluoroscopic, ultrasonographic, MRI, and four-dimensional (4D) CT studies [60–67], the peak-to-peak magnitude of movement associated with respiratory motion can be as large as 20–30 mm [68]. Using cine MRI, Feng et al. [65] found that the motion of the borders of pancreatic tumors was highly variable among patients and reported that a margin of 20 mm inferiorly was required to provide 99 % geometric coverage. Mori et al. [66] used a 256-multislice 4D CT scanner and verified a displacement of the GTV by >10 mm in the superior-inferior direction.
Traditionally, radiation treatment planning for pancreatic cancer involves adding large margins around the CTV to generate the PTV [67] to account for respiratory motion during free breathing; however, the use of large margins can result in high-grade gastrointestinal toxicity [68], as the PTV often contains large volumes of organs at risk.
Respiratory motion of the pancreas is also a critical factor limiting IMRT. The interplay between motion of the multileaf collimator and motion of the target could lead to degradation of the planned dose distribution [69, 70]. Several investigators have proposed various dose delivery methods to resolve problems related to respiratory motion. The use of breath-hold (BH) techniques can reduce the impact of respiratory motion and has been used successfully in the treatment of cancer at various sites [71–76]. Dawson et al. showed the reproducibility of organ position using active breathing control during liver radiotherapy [74]. Hanley et al. applied a deep inspiration BH technique for the treatment of thoracic cancer [75]. Respiratory gating has been used for the treatment of lung and liver cancer [76–79]. Taniguchi et al. showed in a dosimetric analysis that expiratory gating might be preferable to inspiratory BH and free breathing for avoiding normal tissue toxicity [80]. Dynamic tumor tracking [81–84] has reduced the impact of respiratory motion and interfraction motion during radiation treatment. Gibbs et al. found the CyberKnife system, which includes dynamic tumor tracking, to be one of the most effective techniques for treating pancreatic tumors that move with respiration [85].
At Kyoto University, the use of a visual feedback technique during end-exhalation (EE)-BH conditions led to highly reproducible positioning of pancreatic tumors [67, 86]. IMRT in combination with EE-BH could be a reliable method to facilitate dose escalation in locally advanced pancreatic tumors with small margins. This technique and its application in the treatment of locally advanced pancreatic cancer are described in the following sections:
17.2.3.2.1 Simulation and Planning for IMRT with the End-Expiration Breath-Hold Technique: The Kyoto University Regimen
CT-Based Simulation
For CT-based treatment simulation, patients fast for at least 3 h and are positioned supine on individualized vacuum-molded pillows to ensure immobilization (BodyFIX, Medical Intelligence, Schwabmünchen, Germany) with both arms raised (Fig. 17.1). A marker block with two infrared-reflecting dots is placed on the anterior abdominal surface. A real-time position management (RPM) system (Varian Medical Systems, Palo Alto, CA) is used to monitor the abdominal skin-surface displacement in the anterior-posterior direction. Patients are given video goggles that display the extent of abdominal displacement acquired by the RPM system; the abdominal motion signal gives visual feedback to the patient. CT scans are obtained during EE-BH at a slice thickness of 2.5 mm.
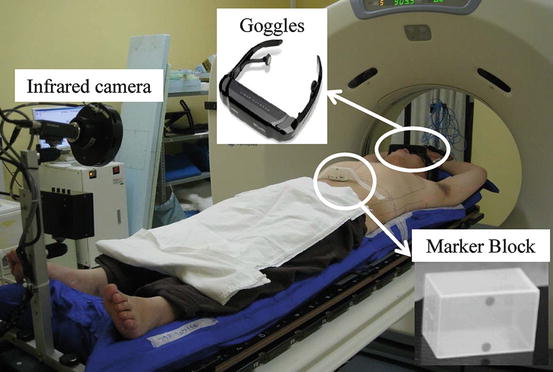
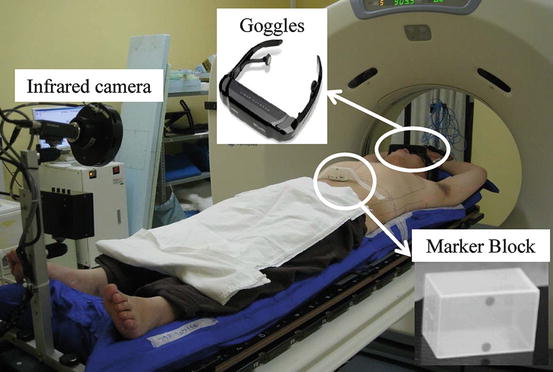
Fig. 17.1
Patient setup. All patients were placed supine on an individualized vacuum pillow with both arms raised, and an infrared-reflective marker block was placed on the anterior abdominal surface. Patients wore goggles on which the extent of abdominal displacement was displayed for visual feedback
IMRT Planning
The GTV includes the primary tumor, and the CTV is defined as the GTV plus a 5-mm isotropic margin. The retropancreatic space between the root of the celiac trunk and superior mesenteric artery is included in the CTV. The PTV is the CTV surrounded by another 5-mm isotropic margin. The GTV, stomach, duodenum, small intestine, liver, kidneys, and spinal cord are delineated. The planning OAR volume is determined from the BH-CT for the dose-limiting organs (the stomach, duodenum, and small intestine). The PTV is divided into two regions to satisfy the dose constraints for the PTV and planning OAR volume, i.e., the volume after deducting the planning OAR volume from the PTV and the volume where the PTV and planning OAR volume overlap.
IMRT plans are based on the BH-CT set produced by inverse planning by a commercially available planning system (Eclipse version 8.6; Varian Medical Systems). The prescribed dose is 39 Gy in 15 fractions, based on the clinical results from McGinn et al. [45] and Allen et al. [87]. Five coplanar ports with gantry angles of 40°, 100°, 180°, 260°, and 320° are selected. Radiation is delivered with a 15-MV photon beam at a dose rate of 600 monitor units (MU)/min. The radiation dose is calculated by using the analytical anisotropic algorithm (version 8.6.15) with heterogeneity correction. The calculation grid size is 2.5 mm × 2.5 mm. Dose-volume constraints are summarized in Table 17.2.
Table 17.2
Dose-volume constraints in intensity-modulated radiotherapy planning in the Kyoto University regimen
Structure | Dose-volume constraints |
---|---|
PTV | Maximum dose ≤110 %a |
D98% >36 Gy | |
PTV minus Planning OAR Volume | D95% >95 %a |
Stomach and duodenum | V42 < 0.5 mL |
V39 < 1.0 mL | |
V36 < 10.0 mL | |
Liver | Mean dose <30 Gy |
Kidney | V20 < 30 % (in each kidney) |
Spinal cord | Maximum dose <36 Gy |
After the optimization process, the intensity fluences are converted into continuous sets of multileaf collimator segments for dynamic delivery. MUs for each port are kept at <150 MU so that the duration of the EE-BH is kept at 15 s or less, with a dose rate of 600 MU/min. Typically the IMRT technique involves using 5–7 ports to deliver the entire prescribed set of MUs, and one set of MUs in one port can be delivered during a 15-s EE-BH by using the highest dose rate. Therefore, one fraction requires a number of BHs that equal the number of ports. An IMRT dose distribution on a CT image obtained during EE-BH while the patient uses the visual feedback technique is shown in Fig. 17.2 .
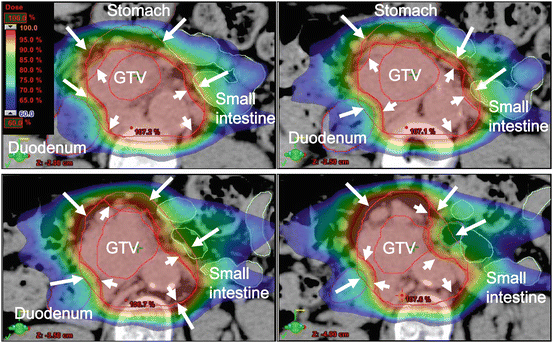
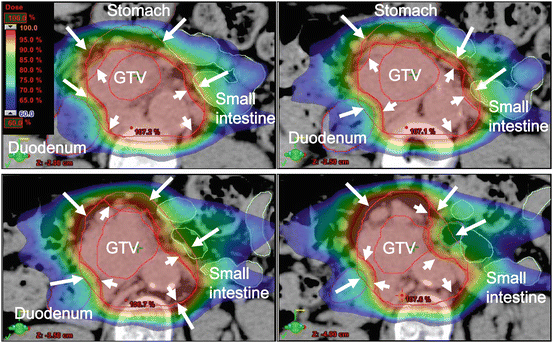
Fig. 17.2
Dose distribution on a CT scan obtained during an end-exhalation breath-hold with a visual feedback technique during IMRT, delivered using the Kyoto University regimen. The CTV is defined as the GTV plus a 5-mm isotropic margin and the retropancreatic space between the root of the celiac trunk and the superior mesenteric artery. PTV1 is the CTV plus another 5 mm of isotropic margin (long arrow). A prescribed dose of 39 Gy in 2.6-Gy daily fractions was specified for PTV2 (small arrow), which is the volume remaining after subtraction of the planning OAR volume from PTV1. The OAR volume includes the volume of the stomach plus a 10-mm isotropic margin, and the volume of the duodenum, the small intestine, and the spinal cord, plus 5-mm isotropic margins. The dose to the PTV1 was to be at least 36 Gy in 15 fractions. A dose-escalation study based on this regimen is ongoing at Kyoto University
Quality Assurance
The American Association of Physicists in Medicine Task Group 76 recommended that respiratory management techniques such as BH, respiratory gating, and dynamic tumor tracking techniques be considered in cases where organ motion of >5 mm is observed in any direction [88]. Although the BH technique is applied to a moving target, the position of that target can still vary (Fig. 17.3). Investigators at Kyoto University evaluated the source and effects of variations in respiratory pattern under EE-BH conditions, such as pulsation from the abdominal aorta and baseline drift, on BH/IMRT dose distribution. That group found that the effect of aortic arch pulsation on BH/IMRT dose distribution was often negligible; a baseline drift of >5 mm should be avoided in using the BH/IMRT [89].
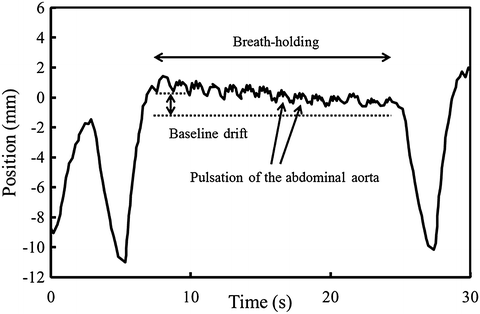
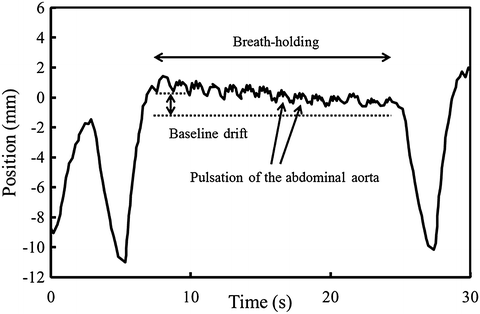
Fig. 17.3
An abdominal motion pattern as documented by an external respiratory monitoring system during end-exhalation breath-hold. Abdominal motion varied with pulsation of the abdominal aorta and with baseline drift
An example of the EE-BH technique used for IMRT for locally advanced pancreatic cancer is given in the case report that follows.
17.2.3.2.2 Case Presentation: Using the Kyoto University Regimen to Treat Locally Advanced Pancreatic Cancer with IMRT
A 43-year-old man presented with a 1-month history of epigastric and back pain. CT (Fig. 17.4a) and sonographic imaging showed a mass in the pancreatic head. At laparotomy, the tumor was deemed unresectable because of invasion of major vessels including the superior mesenteric artery. The diagnostic workup revealed a 4-cm pancreatic adenocarcinoma that was diagnosed as stage III (cT4N0M0). The recommended treatment was gemcitabine-based induction chemotherapy followed by consolidative chemoradiation therapy; the patient is elected to participate in a dose-escalation clinical trial involving BH/IMRT that was underway at Kyoto University Hospital.
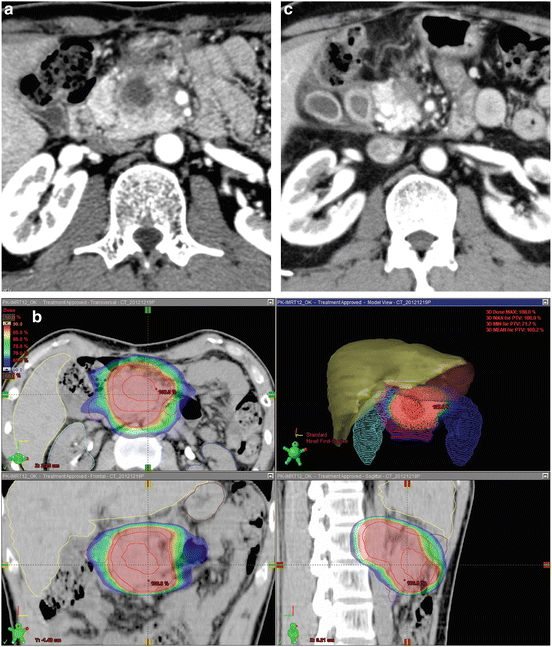
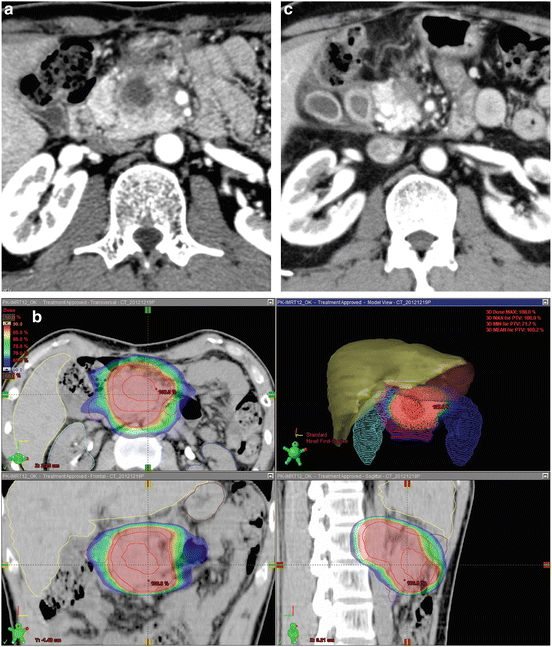
Fig. 17.4
A 43-year-old man presented with epigastric and back pain. (a) CT scans showed a 4-cm mass in the pancreatic head, which was diagnosed as stage III (cT4N0M0) adenocarcinoma. Breath-hold IMRT was administered concurrently with once-weekly full-dose (1,000 mg/m2) gemcitabine. (b) IMRT dose distribution: the prescribed dose was 48 Gy in 3.2-Gy daily fractions for the volume after subtraction of the planning OAR volume from the PTV and 36 Gy in 2.4-Gy daily fractions for the entire PTV. (c) CT scans of the tumor 2 months after treatment
CT simulation and IMRT planning were done according to the Kyoto University regimen, as described in the previous section. The prescribed dose of 48 Gy in 3.2-Gy daily fractions was specified to the PTVboost region (the volume after subtracting the planning OAR volume from the PTV), and the minimum dose to the entire PTV was to be at least 36 Gy in 15 fractions (Fig. 17.4b). Dose constraints for each OAR are shown in Table 17.2.
BH/IMRT took place concurrently with once-weekly full-dose (1,000 mg/m2) gemcitabine. The patients fasted for at least 3 h before receiving each daily fraction. During the treatment, slight nausea and vomiting (<3 times a day) were observed during the acute phase, as was mild abdominal pain. These symptoms were controlled completely with medication, and the patient was able to complete the chemoradiation therapy as planned.
At 3 months after IMRT, the tumor diameter had shrunk by >20 % tumor (Fig. 17.4c), and tumor markers had returned to normal levels. When this chapter was written, the patient had had 12 months of maintenance systemic chemotherapy with no evidence of tumor relapse.
17.3 Conclusion
Both local and systemic progressions of pancreatic cancer are very rapid. The key to control of such tumors via radiation therapy is to reduce toxicity. This allows delivery of intensive systemic therapy and optimization of radiation doses. IMRT can potentially deliver highly conformal doses to pancreatic tumors and facilitate intensification of local therapy without enhancing normal tissue toxicities. Clinical assessment of IMRT as a treatment for pancreatic cancer is in its infancy, but several promising results have already emerged. Further investigations are expected.
Acknowledgments
This writing is supported by Research Infrastructure Fund of Yamaguchi University.
Conflicts of Interest: Ultrasonographic MRI
The authors declare no conflicts of interest.
References
2.
3.
4.
5.
Moertel CG, Frytak S, Hahn RG, O’Connell MJ, Reitemeier RJ, Rubin J, Schutt AJ, Weiland LH, Childs DS, Holbrook MA, Lavin PT, Livstone E, Spiro H, Knowlton A, Kalser M, Barkin J, Lessner H, Mann-Kaplan R, Ramming K, Douglas HO Jr, Thomas P, Nave H, Bateman J, Lokich J, Brooks J, Chaffey J, Corson JM, Zamcheck N, Novak JW (1981) Therapy of locally unresectable pancreatic carcinoma: a randomized comparison of high dose (6000 rads) radiation alone, moderate dose radiation (4000 rads + 5-fluorouracil), and high dose radiation + 5-fluorouracil: The Gastrointestinal Tumor Study Group. Cancer 48(8):1705–1710PubMed
6.
Gastrointestinal Tumor Study Group (1988) Treatment of locally unresectable carcinoma of the pancreas: comparison of combined-modality therapy (chemotherapy plus radiotherapy) to chemotherapy alone. J Natl Cancer Inst 80(10):751–755CrossRef
7.
Burris HA 3rd, Moore MJ, Andersen J, Green MR, Rothenberg ML, Modiano MR, Cripps MC, Portenoy RK, Storniolo AM, Tarassoff P, Nelson R, Dorr FA, Stephens CD, Von Hoff DD (1997) Improvements in survival and clinical benefit with gemcitabine as first-line therapy for patients with advanced pancreas cancer: a randomized trial. J Clin Oncol 15(6):2403–2413PubMed
8.
9.
Ueno H, Ioka T, Ikeda M, Ohkawa S, Yanagimoto H, Boku N, Fukutomi A, Sugimori K, Baba H, Yamao K, Shimamura T, Sho M, Kitano M, Cheng AL, Mizumoto K, Chen JS, Furuse J, Funakoshi A, Hatori T, Yamaguchi T, Egawa S, Sato A, Ohashi Y, Okusaka T, Tanaka M (2013) Randomized phase III study of gemcitabine plus S-1, S-1 alone, or gemcitabine alone in patients with locally advanced and metastatic pancreatic cancer in Japan and Taiwan: GEST study. J Clin Oncol 31(13):1640–1648CrossRefPubMed
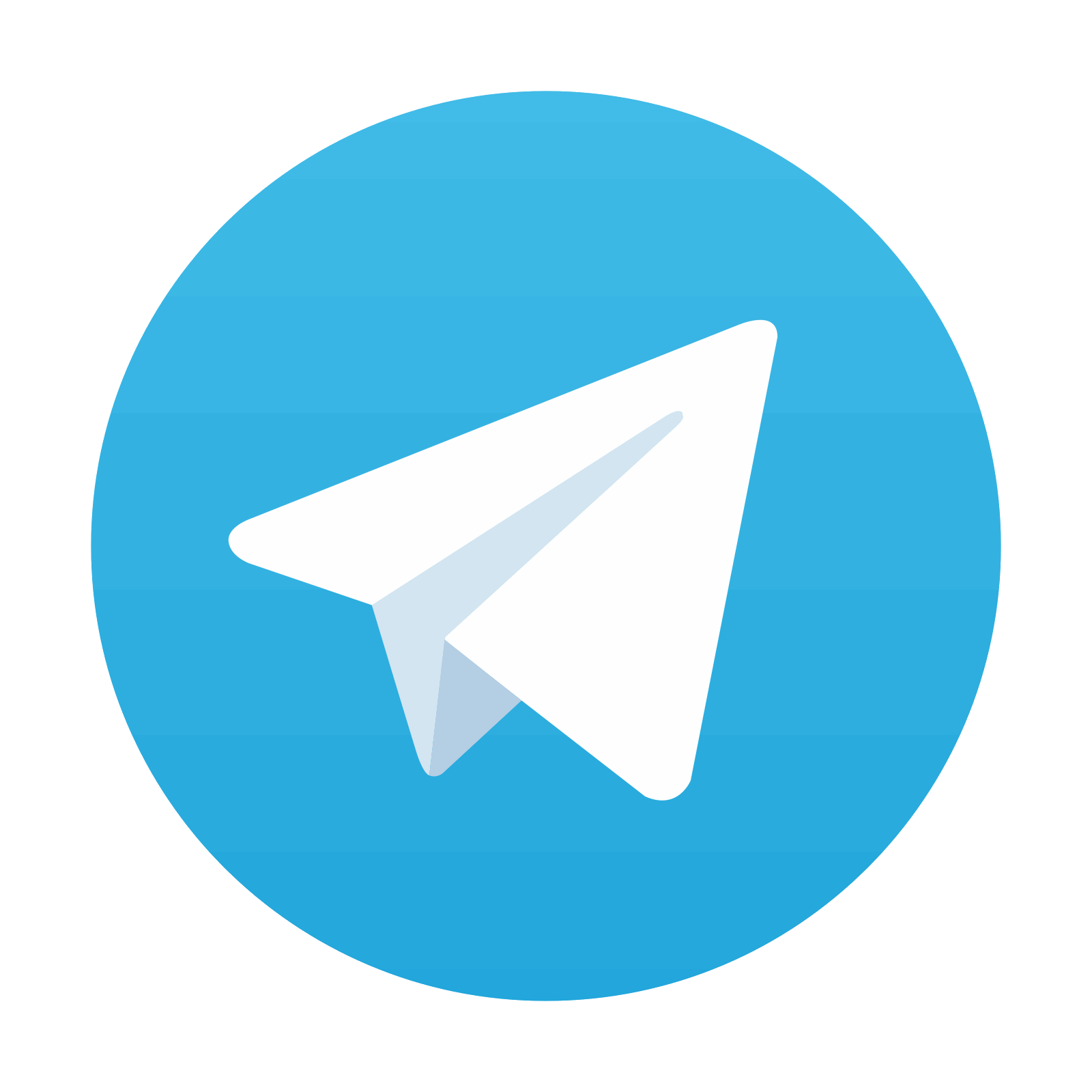
Stay updated, free articles. Join our Telegram channel

Full access? Get Clinical Tree
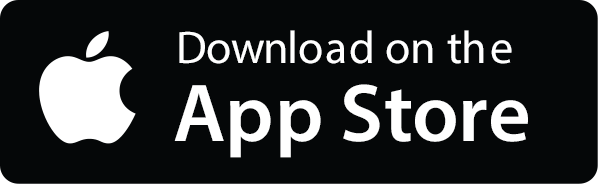
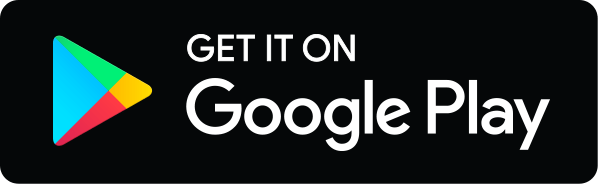