Parallel Imaging
Mark Doyle
OVERVIEW
Parallel imaging, sometimes referred to as reduced field of view (FOV) imaging, is a means to rapidly acquire k-space data by exploiting the spatial relationships that exist between several imaging receiver coils. Alternatively, parallel imaging can be used to acquire high-resolution k-space matrices in a conventional scan time. Parallel imaging is a feature unique to magnetic resonance imaging (MRI) because it exploits special properties of k-space.
REDUCED FIELD OF VIEW BASIC PRINCIPLES
An understanding of the basic properties of k-space is central to appreciating the mechanism of parallel imaging. Each magnetic resonance (MR) data set is acquired in the k-space domain, which is achieved by application of a frequency encoding gradient, in combination with successive applications of a phase encoding gradient to encode each line of k-space separately. Once assembled, the k-space data are Fourier transformed to generate an image. Normally, the number of lines acquired in k-space is determined by a combination of the FOV of the body region being imaged and the resolution required. When the FOV is made smaller and resolution maintained, the net scan time is reduced, but signal aliasing may result as the FOV becomes smaller than the body region. Usually, some degree of signal aliasing is permissible, especially if the region of interest is toward the center of the image, and this region is unaffected by the folded over signal. Further, resolution is uniform throughout the image, such that image regions contaminated by fold-over data, and regions not obscured by signal aliasing are represented at the same resolution as in a higher FOV scan.
PARALLEL IMAGING APPROACH
Parallel imaging is an FOV reducing strategy that avoids the fold-over artifact that is associated with conventional reduced FOV approaches. There are two features that make parallel imaging possible when k-space data are acquired corresponding to a small FOV:
Signals corresponding to spatially distinct receiver coils are separately acquired.
Special image processing is applied.
A key feature of parallel imaging is the use of several receiver coils. Coils are typically configured with 4, 8, 16, or even more elements. Each coil element primarily receives a signal from a localized region of the body. In this scenario, coil elements close to a slice region receive the strongest signal from that region, whereas coil elements that are more distant receive relatively little signal. Further, some coil elements, which have sensitivity to regions outside of the selected FOV, will contribute strongly to alias artifact (see Fig. 26-1). As is common to alias artifact, the aliased signal appears at a region remote from the source of the signal (see Fig. 26-2). Each coil element generates a complete image, and these are typically combined to form an image with increased signal-to-noise ratio (SNR). However, by the simple addition of multiple images form the multiple coils, the image contribution from some coil elements may introduce substantial fold-over artifact to a region that, when imaged by a separate coil element, did not previously have a significant artifact. In this way, addition of the separate images may serve to distort some regions while improving the SNR in others.
Fold-over artifacts originate from tissue outside of the FOV, but within the sensitivity range of the receiver element(s). Depending on the sensitivity characteristics of the receiver coil, the fold-over artifact may be very faint if the region is physically remote from the coil
element or bright if close. Stated mathematically, each separate receiver coil contributes the following two features of interest:
element or bright if close. Stated mathematically, each separate receiver coil contributes the following two features of interest:
A range of sensitivities over the body
A range of contributions to fold over artifacts
In the final image, which is a composite of each separate image, all these features are apparent, and only prior knowledge of the coil sensitivities allows the details of the fold-over artifact to be appreciated.
REDUCED FOLD-OVER APPROACH
To avoid aliasing artifact, we could consider a first-order approximation approach, whereby a composite image is compiled only from regions of the separate images in which each region is best observed, with good SNR and without appreciable signal alias. In this (generally unworkable) approach, the signal sensitivity is reduced, because no signal averaging occurs, and the final image looks like a patchwork with sharp intensity transitions. This is an unsatisfying approach because not all the coil information is used.
![]() FIGURE 26-2 The separate contributions from two coil elements are shown, one positioned on the chest wall (top left) and one positioned on the back (cover left). There is only one field of view (FOV) for the entire composite image (right). However, the chest coil has high sensitivity to the heart (light circle on chest coil image) and low sensitivity to the back (dark circle on chest coil image). Conversely, the back coil has high sensitivity to the back (dark circle on back coil image) and low sensitivity to the chest. However, the back coil contributes a large artifact signal (light circle on back coil image) originating from the back region but appearing over the chest wall. |
K-SPACE AND THE FIELD OF VIEW
Another, more general, approach can be used, which exploits some of the properties of k-space. There is a relationship between k-space and the FOV. When k-space is “fully sampled,” it means that the sampling density in k-space matches the FOV, that is, the number of lines sampled and the line spacing is such that the FOV is correctly encompassed. To increase the FOV, the sampling density of k-space must be increased. Conversely, to reduce the FOV
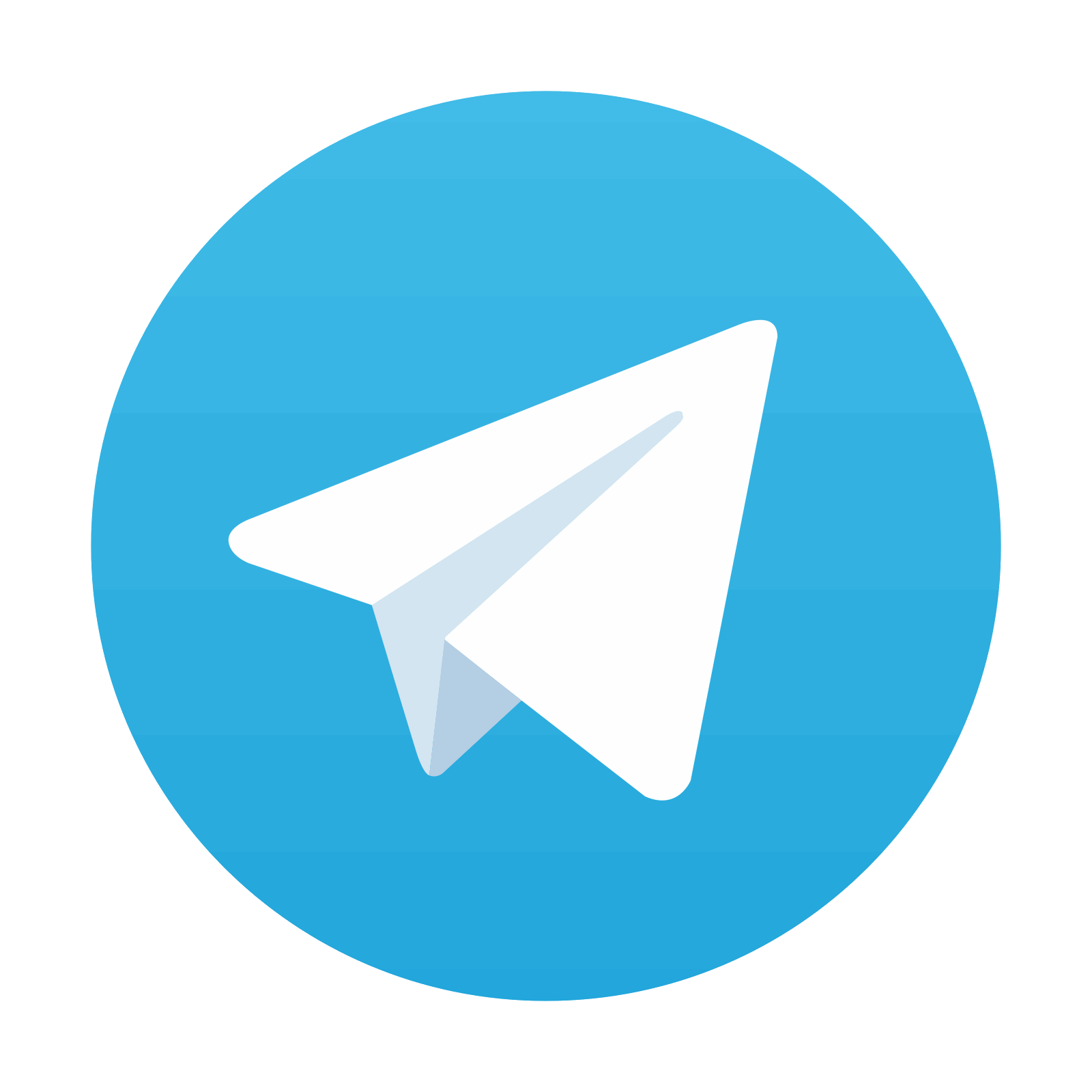
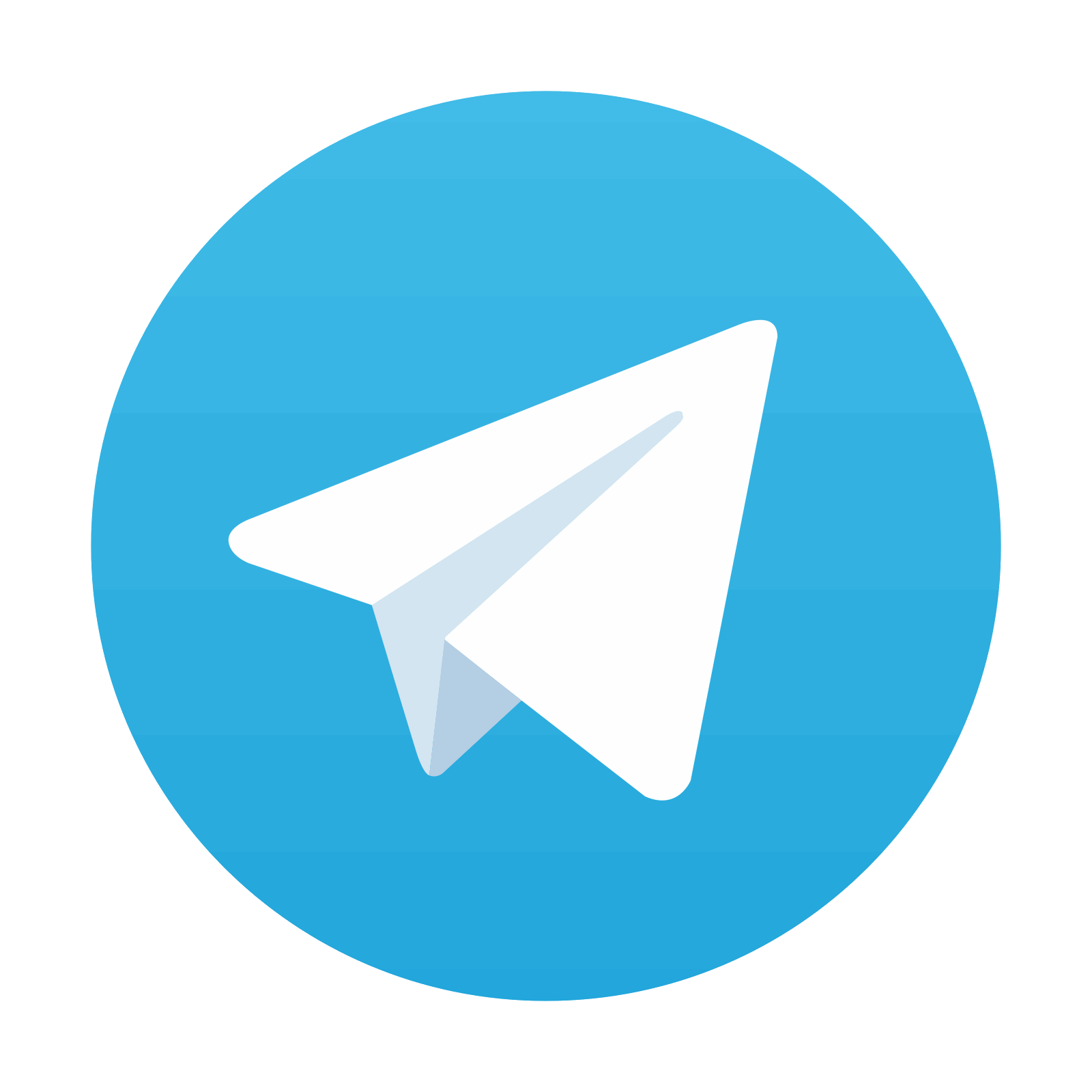
Stay updated, free articles. Join our Telegram channel

Full access? Get Clinical Tree
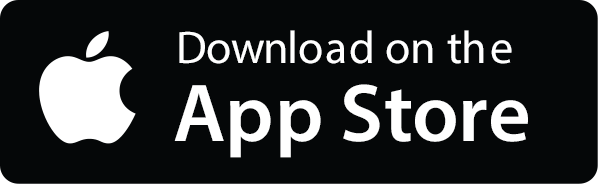
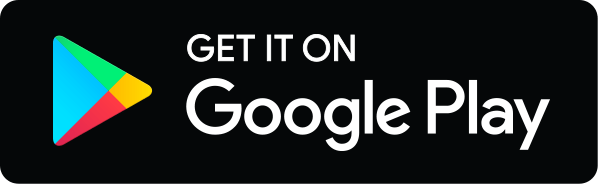