Fig. 10.1
Cardiac probe (a) and curvilinear probe (b). Both are low frequency probes (2–5 MHz) which allow for adequate visualization of pleural effusions and deeper thoracic structures
Unlike in the abdomen, there are no cystic structures in the chest to assist in optimizing gain on the US machine for visualizing fluid densities [6]. The liver and the spleen may instead serve as reference structures for determining the gain needed to image heterogeneous structures clearly (Fig. 10.2). At that level of gain, increasingly anechoic findings will more likely represent fluid. Under normal conditions, air-filled lung has high acoustic impedance that facilitates the distinction between subcutaneous tissue, pleura, and pulmonary parenchyma. A free-flowing pleural effusion (transudates, noncomplicated exudates) represents a homogeneous, anechoic media compared to surrounding structures. Tissue-specific acoustic profiles are frequently blurred in the presence of exudative effusions, particularly when the pleural space contains pus, cells (inflammatory or malignant), clot, or other relatively echoic materials.
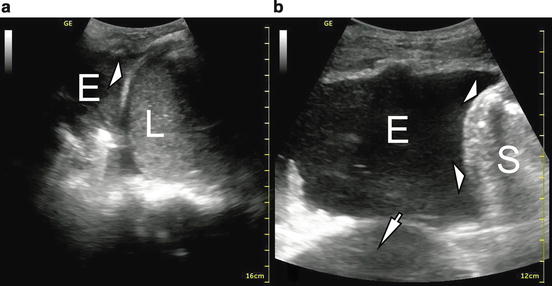
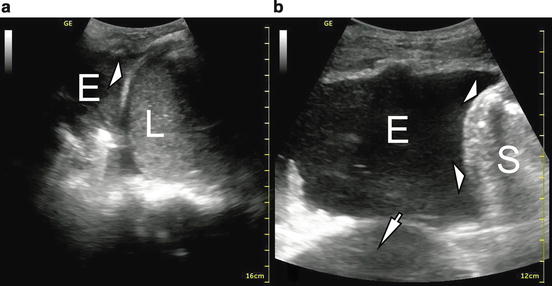
Fig. 10.2
B-mode image of pleural effusions. L liver, S spleen, E effusion. Standard gain for appropriate imaging of the liver (a) or spleen (b). Diaphragm (white arrowheads). In image b, a pericardial effusion can be seen (white arrow)
Scanning of the most dependent regions of the thorax is usually indicated in order to evaluate an effusion. If a patient can be positioned upright, all areas of the chest may be scanned for effusions. A short-handled probe may be wedged between a supine or semi-recumbent, nonmobile patient and the bed in order to visualize posterior collections, though this technique may be uncomfortable for both the patient and the sonographer. Alternatively, patients may be situated in a lateral decubitus position to facilitate ultrasound evaluation, especially for moderate to large effusions. Patients with previously established pleural space infections may warrant a more comprehensive examination of less dependent regions to maximize the likelihood of locating clinically relevant and potentially drainable pleural fluid collections. As is the case with other point of care ultrasound applications, “ruling in” a pleural fluid collection with bedside ultrasound tends to be easier than comprehensively ruling out the presence of a pleural fluid collection, especially a loculated, nondependent one.
Patients on mechanical ventilation, particularly those on high levels of positive end expiratory pressure (PEEP), may be at higher risk of complications from thoracentesis. Particular care must be taken in this population to avoid iatrogenic pneumothorax. A minimum amount of pleural fluid for thoracentesis in mechanically ventilated patients is not known, but effusions 15 mm thick have been safely sampled using US [17]. An experienced proceduralist using US guidance should be required for pleural fluid sampling in patients on mechanical ventilation.
Image Acquisition and Interpretation
With the probe indicator pointing cephalad and the orientation marker on the upper left corner of the US display screen, superior structures will be oriented screen-left. The same superficial structures seen in pneumothorax evaluation (discussed below) should be seen in pleural effusion analysis, but due to the deeper field of view, they will often appear closer to the top of the screen (more superficially) and appear smaller in comparison. These include subcutaneous tissue, ribs and rib shadows, sliding lung (apposed parietal and visceral pleura), and B-lines.
A key anatomic landmark in the analysis of an effusion is the hemidiaphragm on the side of interest. When imaging the lower thoracic area, the diaphragm appears as a curved, band-like, smooth-bordered structure originating superficially and inferiorly and arching deep and superiorly (Fig. 10.3). Respirophasic motion of this structure, particularly in the spontaneously breathing patient, should aid in differentiating the diaphragm from aerated lung. Identifying the diaphragm with confidence will help the proceduralist locate an appropriate site for thoracentesis or tube thoracostomy. As free fluid (e.g., ascites and cystic fluid collections) can also be located below the diaphragm, identifying anechoic fluid while scanning the lower thorax is insufficient to diagnose a pleural effusion. The hemi-diaphragm must first be identified to ensure that the apparent fluid identified is cephalad to the hemidiaphragm, thus preventing nondiagnostic or erroneous sub-diaphragmatic punctures with potentially serious complications.
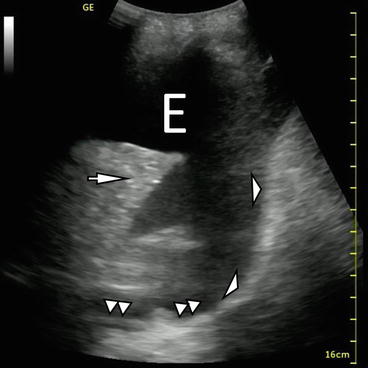
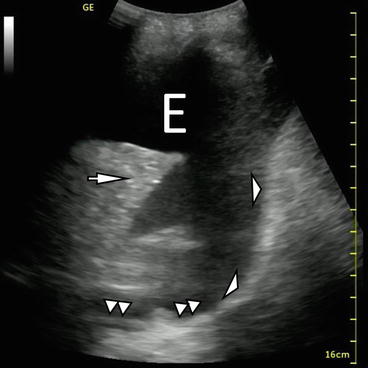
Fig. 10.3
B-mode imaging of diaphragm (white arrowheads), effusion (E), and atelectatic lung (white arrow). The V-line, a sonographic representation of the thoracic spine (double white triangles) is also demonstrated
On the left side, concurrent pericardial effusion can sometimes be seen (Fig. 10.2). When imaging the thorax from the mid-axillary line, Atkinson et al. [21] reported that the ultrasonographic signature of the thoracic spine – a densely echoic line extending cephalad from the deep portion of the diaphragm – is only visible when pleural fluid has displaced the normally interposed lung. They argue that visualization of the deep and posterior spine (termed V-line) is strongly supportive of the presence of pleural fluid (Fig. 10.3).
Heterogeneously echoic structures below the diaphragm are the spleen (left side) or the liver (right side). Ultrasonographic characteristics of pleural fluid can lend clues to the etiology of the fluid itself. Pleural fluid will generally appear anechoic, though the presence of cells, septations, clot, or air in the pleural space can cause a heterogeneous appearance (Figs. 10.4 and 10.5). Free-flowing fluid usually appears homogeneously anechoic. Complex fluid due to hemopneumothorax, parapneumonic, or malignant effusions can assume a range of ultrasonographic appearances. However, for reasons discussed below (see Evidence Review and Evidence-Based Use), use of the ultrasound appearance of pleural fluid alone to guide decision-making regarding whether diagnostic sampling or other procedures should occur should generally be avoided.
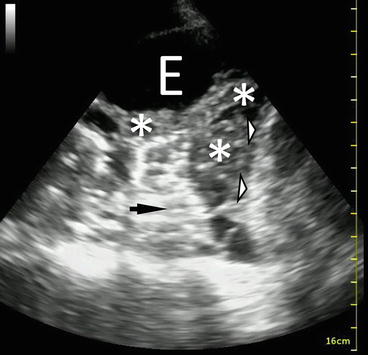
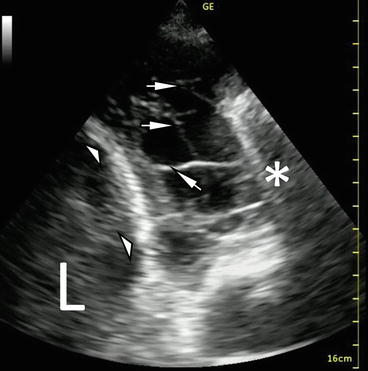
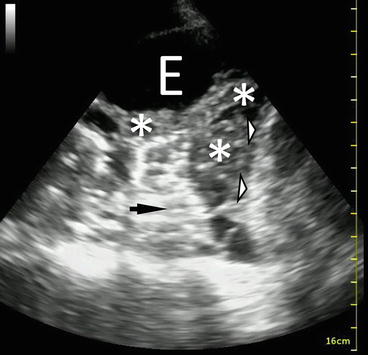
Fig. 10.4
B-mode image of a complex pleural effusion with visible loculations. E effusion. Diaphragm (white arrowheads), lung (black arrow), complex loculated effusion (asterisks)
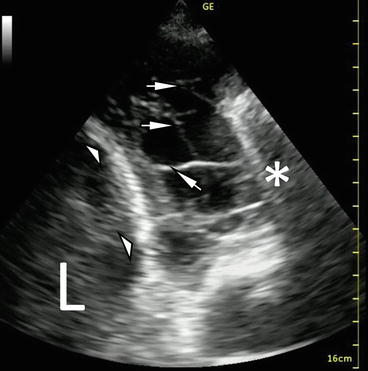
Fig. 10.5
B-mode picture of septated (complex) pleural effusion imaged with cardiac probe. Note that cephalad is to the right in this image. Diaphragm (white arrowheads), septations in the pleural space (white arrows). A single B-line (white asterisk) can also be seen projecting deep from the visceral pleura. L liver
Basic Competencies
The principle goals for point of care US in the evaluation of pleural effusion should be to identify the presence or absence of pleural fluid and to determine the feasibility of diagnostic or therapeutic thoracentesis. The initial ultrasonographic approach to the patient should consider patient positioning and identification of key anatomic landmarks. If a patient can sit up in bed and lean forward over a table, a detailed examination of the lower posterior thorax can be performed to more thoroughly assess dependent fluid collections.
The area from the mid-axillary line to approximately mid-scapular line should be systematically scanned with a curvilinear or cardiac probe, and the diaphragm should be clearly identified on the right side (inferior) of the screen. The probe should be applied firmly to the skin, with adequate interposed gel, and oriented perpendicularly to the skin at all times. Inspiration, either patient or ventilator-driven, can help to identify the diaphragm through its downward motion. Inferior to the diaphragm, the spleen or liver should be identified. We often mark with a sterile marker the level of the diaphragm, keeping in mind that diaphragm motion will change that level slightly during the respiratory cycle. Scanning above the level of the diaphragm can then be performed to identify relatively anechoic collections consistent with pleural effusion. Observation of atelectatic or consolidated lung in the far field is helpful in confirming the presence of interposed pleural fluid between the parietal and visceral pleura (Fig. 10.2). If pleural fluid is identified, the depth of the effusion should be measured with the gradations on the screen. The approximate thickness of subcutaneous tissue should be noted and needle length adjusted accordingly if instrumentation is being considered. We then typically scan superiorly, identifying the vertical height of the effusion; the interface where the effusion diminishes in depth and eventually disappears, replaced by the bright reflection of air-filled lung, marks the superior edge of the effusion. This upper border of the effusion can also be marked with a sterile marker for reference.
Since images will only be obtained through intercostal windows, a skin mark may be made superficially just above the superior border of the lower rib of the appropriate intercostal space in order to guide subsequent thoracentesis or tube thoracostomy. The ribs should be palpated, and marking the course of the ribs above and below the procedural mark with a sterile marker can be helpful for orientation. We usually identify a relatively dependent site to maximize ability to extract pleural fluid while maintaining a margin of safety above the dome of the diaphragm. Keep in mind that the diaphragm level may rise during the course of pleural fluid drainage.
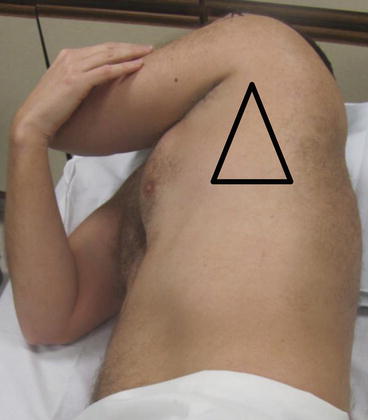
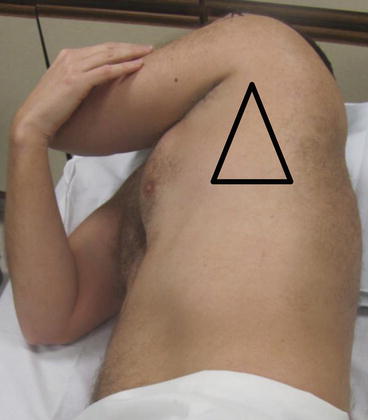
Fig. 10.6
The “triangle of safety” is the recommended location for safe performance of tube thoracostomy. The triangle is demarcated anteriorly by the lateral border of pectoralis major, posteriorly by the lateral border of latisimus dorsi, and inferiorly by the 5th intercostal space
Pleural procedures should be carried out immediately following the ultrasound and site marking, and should be performed in the same patient position and directional orientation as the ultrasound (perpendicular to the skin surface). Excessively medial posterior (i.e., towards the spine) puncture sites for thoracentesis should be avoided due to the risk of puncture or laceration of the intercostal artery, which is more likely to be exposed below the rib in this region [22]. The site identified for thoracentesis should be above the diaphragm, in a relatively lateral position, and identified as having an adequate depth of fluid.
For chest tube placement, lateral positions in the “triangle of safety” should be considered, depending on patient circumstance and fluid location. The “triangle of safety” is denoted by the lateral border of the pectoralis major anteriorly, by the lateral border of the latissimus dorsi posteriorly, and by a line extended along the 5th intercostal space inferiorly (Fig. 10.6) [23]. The chosen area should be cleaned, then sterilely prepped and draped after ultrasound. We find that real-time ultrasound using a sterile probe cover is sometimes beneficial but is typically not necessary during most pleural procedures.
The volume of pleural fluid can be estimated with one of the several published techniques. Balik et al. [9] found the maximal distance between the diaphragm and the base of the lung at end-expiration correlates with the volume of effusion according to the following equation: volume of effusion (in mL) = 20 × distance of separation (in mm). Another group [24] found that end-expiratory intrapleural distances > 45 mm may reliably predict larger (around 800 mL) effusions. Remerand et al. [10] calculated pleural fluid volume by multiplying the area of the effusion (as measured in two ultrasonographic planes) in supine patients with free-flowing effusions at the midpoint between caudal and apical limits of the effusion. No one method has been exhaustively validated, but the method by Balik is frequently cited in the literature [4, 9–11].
Advanced Competencies
Different disease processes of the pleura and pleural space may be elucidated sonographically based on varying acoustic profiles [20, 25]. Four patterns have been described to categorize the effusion in a manner that suggests a specific disease process. Anechoic fluid is homogeneously dark and is most consistent with transudative fluid. Complex nonseptated fluid contains more cellular debris, fibrin, blood, and is more likely to be exudative. Complex septated fluid may have organized locules, septations, and pleural adhesions, and is consistent with exudative fluid that likely requires tube thoracostomy [26]. Homogeneously echogenic fluid is generally consistent with empyema. Establishing appropriate gain settings is crucial to the correct interpretation of complex pleural fluid images, since overgain may cause relatively anechoic fluid to appear more echogenic.
Color Doppler has been reported as a means to distinguish small effusions from pleural thickening [27]. In patients suspected of having effusion based on radiography, Doppler analysis can be performed in addition to standard B-mode imaging. When B-mode images suggest the presence of effusion, a positive Doppler signal suggests the presence of fluid (dynamic) rather than pleural thickening (static). M-mode identification of the pleural fluid sinusoid sign can also be helpful in distinguishing small effusions from pleural thickening [28]. As most point-of-care pleural ultrasound in the intensive care unit is done to determine an optimal site for thoracentesis or tube thoracostomy, making a fine distinction based on ultrasound between a very small pleural effusion that is unlikely to be clinically significant and pleural thickening is not likely to be excessively important in the critical care setting.
Evidence Review and Evidence-based Use
A growing body of evidence supports the use of US in routine evaluation and management of pleural effusions in the critically ill. As a diagnostic tool, US outperforms conventional chest radiography. Diacon et al. [3] prospectively compared clinical exam and CXR to US for identifying a site of at least 10 mm of fluid for thoracentesis in 255 cases for possible instrumentation. US increased the number of accurate sites detected by 26 % and prevented possible organ puncture in 15 % of clinically and radiographically determined sites. Kocijancic et al. [25] determined that US had a positive predictive value of 92 % for detecting small effusions. In the surgical intensive care unit, a focused thoracic US examination demonstrated 83.6 % sensitivity, 100 % specificity, and 93.6 % accuracy for detecting pleural effusion [29].
In another study of pleural effusion in ICU patients the sensitivity of handheld US and standard radiography was 91 % and 74 %, respectively (not statistically significant). Specificity was 100 % for US versus 73 % for CXR (p = .008). The authors attribute three cases of US false negative to the inability to diagnose a small effusion (<50 mL) in patients with higher body mass indices (BMIs) [30]. Additionally, the ability of US to differentiate effusion from consolidation was demonstrated in a study of 97 ICU patients with findings suggestive of effusion on CXR. Effusion was better predicted by US (R2 = 0.74, ROC 0.99) compared to CXR (R2 = 0.51, ROC 0.70). The lack of lateral chest radiography in the ICU may weaken the ability of CXR to reliably identify pleural effusion [24].
Quantitation of pleural fluid volume by US has been well studied. Rothlin et al. [31] used portable handheld US in trauma patients to quickly rule in the presence of pleural fluid, which in that setting helped to diagnose traumatic hemothorax. More specific techniques to ascertain the precise volume of pleural fluid have been described by Balik et al. (discussed above) and Vignon et al. [24]. The latter group found that end-expiratory intrapleural distances of > 45 mm on the right and > 50 mm on the left predicted effusions > 800 mL with sensitivity of 94 % and 100 % and specificity of 76 % and 67 %, respectively, for each hemithorax. Their model was less robust for larger effusions. Roch et al. [32] conducted a prospective study of 42 ICU patients on mechanical ventilation who had effusion identified on CXR and in whom drainage was considered. An ultrasound-measured distance of 5 cm between the posterior chest wall and the lung base correlated with > 500 mL effusions with sensitivity 83 %, specificity 90 %, positive predictive value (PPV) 91 %, and negative predictive value (NPV) 82 %.
US has been shown to be a useful but imperfect diagnostic adjunct to clinical exam and standard radiography in the assessment of pleural fluid characteristics prior to thoracentesis. In a study of 118 thoracenteses in febrile medical ICU patients with effusions, 43 % of collections characterized as either complex septated, homogeneously echogenic, or complex nonseptated and relatively hyperechoic turned out to be empyema on initial or follow-up thoracentesis [12]. Ultrasonographic pleural fluid characteristics must be considered in the overall clinical context, however. Hirsch et al. evaluated 50 patients (24 % in the ICU) and, in 46 aspirations, 16 were complex septated, of which one was a transudate. Anechoic effusions were mixed (6 transudates, 5 exudates, 1 hematoma) [33]. Chen et al. [11] reviewed the US findings of 149 Light’s criteria-confirmed transudates and demonstrated that greater than half had a complex nonseptated appearance. Kearney et al. [13] found that US can better identify septations than CT scan, but that US characteristics did not reliably predict the nature of fluid in empyema. The authors found that some stage I exudates (pH > 7.2, lactate dehydrogenase [LDH] < 1,000 IU/l, glucose > 2.2 mmol/l, no organisms) contained septations and that some anechoic collections turned out to be frank pus when aspirated. These studies have considerable variation in the US protocol used, timing of imaging, and sampling. Further study is needed before US can be used as an effective prognostic tool for pleural effusion in ICU patients.
There is strong evidence that US increases the safety and yield of thoracentesis. Safety has been addressed in small retrospective studies of patients on antiplatelet medications (Abouzgheib 2012, n = 24; Dammert 2013, n = 43) who underwent US-guided pigtail catheter placement and suffered no complications [15, 18]. A larger study of 450 thoracenteses (67.8 % performed with US, 32.2 % performed without) revealed a significantly higher pneumothorax and tube thoracostomy rate of 4.1 % in the non-US group compared to 0.7 % in the US-guided group [17]. Lichtenstein et al. [16] prospectively studied 40 mechanically ventilated patients with effusions at least 15 mm and found no complications (pneumothorax or hemoptysis) in 45 procedures. However, in a prospective, randomized study, Kohan et al. [34] found no difference in the rate of complications in 205 patients who underwent thoracentesis with or without chest ultrasound. Most current studies of thoracentesis or tube thoracostomy involve US guidance in their protocols, reflecting that routine use of ultrasonography in this setting is effectively the standard of care.
The sensitivity and specificity of US operated by newly trained providers is variable, however. After an 8.5 h multimodality training session in US-naïve ICU residents, Chalumeau-Lemoine et al. demonstrated in 23 patients with confirmed pleural effusions a sensitivity of 58.3 % and specificity of 70 % [35]. This suggests the need for adequate and protocolized training in the use of point-of-care US. A questionnaire-based study of pulmonary and critical care fellowship directors identified significant interest in nonvascular applications of US (including evaluation of pleural effusion). Insufficient faculty expertise, lack of knowledge of the data supporting its use, and financial considerations were cited as barriers to more widespread use of US in critical care medicine fellowships [36]. There is also evidence that bedside US may facilitate care of patients with pleural effusion in nonindustrialized countries [37].
Pitfalls and Precautions
User proficiency in both pleural ultrasound and associated pleural procedures must be optimized for effective and safe performance. Guidelines for minimal competencies in pleural ultrasound have not been published, but regular training and use of this technology under the instruction of experienced operators is vital.
Technical proficiency with the US machine will help to avoid errors in depth and gain selection as well as probe orientation. Overgain will mischaracterize pleural fluid as more complex than it really is. Undergain can lead to poor visualization of the lung and overestimation of the size of an effusion.
An assessment of the integrity of the subcutaneous tissue across which the US waves must project is important. Subcutaneous air will rapidly attenuate sound waves and impair visualization of deeper structures, which can lead to false-negative examinations [31].
Always start a pleural ultrasound exam by identifying the hemidiaphragm to avoid subdiaphragmatic punctures. Marking the levels of both the diaphragm and the superior extent of the effusion, as well as careful marking of the chosen site for a procedure, can aid in orientation following sterile draping of the patient.
Noting the depth of the subcutaneous tissues and the relative depth of the pleural fluid pocket on ultrasound can help increase operator confidence and allow for appropriate anesthesia infiltration prior to procedures.
Carry out pleural procedures in the same positioning and orientation as the ultrasound. Avoid excessive time delay between ultrasound and pleural procedure, and rescan if delays or patient movement do occur.
Certain pleural diseases may mimic effusion. Lymphomas and neurogenic neoplasms transmit US with the production of few or little echoes [20].
The stage at which an effusion is imaged is an important consideration. In empyema, for example, fluid at the early exudative stage will appear different than during the late organizing phase [12]. Care must always be taken to interpret US findings in the context of the clinical situation and the established natural history of various pleural effusions [13].
Serial US exams are readily performed and may help to better characterize the evolution of disease processes within the pleural space.
Avoid overreliance on pleural fluid US characteristics to guide decision-making about sampling and drainage – simple-appearing effusions may be exudative in nature, and transudative effusions may appear complex in the setting of preexisting pleural or parenchymal lung disease.
Some pleural fluid may simply abut the lung and not be walled off in a discrete locule. The relatively higher attenuation of lung tissue relative to fluid could lead to misinterpretation of the fluid-lung interface as a septation [6].
Ultrasound for Pneumothorax
Introduction
In the critically ill patient, pneumothorax (PTX) is associated with high morbidity and mortality and can be readily treated. Despite an incidence of only 3–6 %, mortality ranges from 22 % to 68 % [38–40], hence timely diagnosis and management are essential. Under the best of circumstances, diagnosing pneumothorax can be difficult. The ICU setting imposes additional challenges including comorbid hemodynamic instability, patient positioning limitations, inaccessibility of advanced imaging modalities due to patient transport safety concerns, staffing limitations, and others [40].
Historically, the gold standard for diagnosing pneumothorax has been non-contrast enhanced computed tomography (CT). However, the cost, exposure to ionizing radiation, and safety of ICU patient transport make routine CT scanning a suboptimal diagnostic test when PTX is suspected. Chest radiography, usually performed in the supine or semi-recumbent position, has been shown to lack diagnostic accuracy [41–45].
Bedside thoracic ultrasound (US) has been increasingly reported in the literature as a safe, inexpensive, portable, and highly accurate modality for rapid evaluation of PTX in the critically ill patient. First described in the veterinary literature for the assessment of horses with suspected thoracic disease [7], thoracic US has been subjected to increasingly rigorous study in the setting of PTX in humans since the 1980s. Expansion of provider awareness and skill in obtaining and correctly interpreting US images has created a powerful new tool for the ICU provider. Additionally, portable US machines have become commonplace for use in vascular access, hence many ICUs are already equipped with the necessary technology for US evaluation of PTX.
Pneumothorax in the ICU is generally due to iatrogenic causes (mechanical ventilation, post-procedure) or secondary to a range of conditions, including blunt or penetrating trauma, or rupture of preexisting bullous disease [46]. Air enters the pleural space and occupies the least dependent location in the thorax, most commonly anteromedial and subpulmonic recesses based on retrospective studies of PTX in supine and semi-recumbent ICU patients [41]. Still, a significant number of pneumothoraces are missed by portable chest radiography. Particularly in the setting of mechanical ventilation, many progress to exhibit tension physiology, a high mortality yet generally treatable condition if addressed early [40, 41].
Technical and Patient Considerations
Of overarching importance is the fact that ultrasound waves do not penetrate air, thus air in the pleural space will create a characteristic ultrasound image based on the differing acoustic impedance characteristics of aerated or nonaerated tissue. This aspect of pleural ultrasound will be revisited in greater detail in the subsequent discussion of normal and abnormal findings that are key to accurate diagnosis of PTX.
The thoracic ribcage enables only small windows for sonographic visualization of the pleural space. Probe selection must account for this window as well as for the depth of structures of interest. Convex/curved or linear array transducers in the 5–9 MHz range are frequently used for pleural analysis (Fig. 10.7). Freestanding portable US units are most commonly reported in the literature. Handheld units are being increasingly studied in the trauma setting [47].
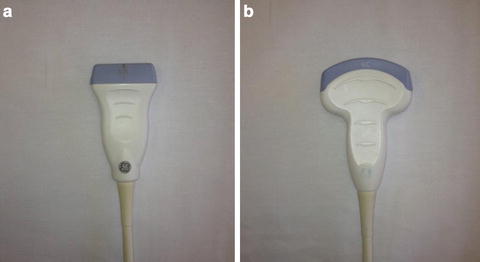
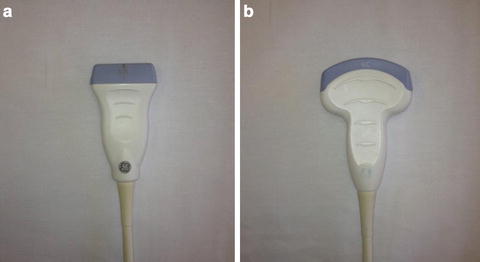
Fig. 10.7
Standard US probes for evaluation for pneumothorax. High frequency linear probe (5–13 MHz) (a), 2.5–5 MHz curvilinear probe (b). The higher frequency enables greater resolution of pleural and other superficial structures and may be preferred
The supine or semi-recumbent position of most ICU patients dictates that pleural air commonly settles in an anteromedial location [41, 48]. Therefore, attention to this area is important when searching for PTX. Preexisting pleural abnormalities such as scarring, adhesions, or plaques may alter the expected anatomic distribution of PTX. A systematic approach to thoracic US has not been firmly established in the literature, however, Lichtenstein et al. [42, 49] outline a method that evaluates the most likely locations in which to identify PTX.
As shown in Fig. 10.8, the anterior and posterior axillary lines divide the thorax into roughly three areas: anteromedial (area 1), lateral (area 2), and posterolateral (area 3). The paraspinal and apical regions have been termed “area 4” and are involved in more comprehensive US examinations of the chest than are routinely performed in clinical practice. The examiner should begin in the second intercostal space in area 1, with the probe marker oriented cephalad (Fig. 10.9). Appropriate anatomic and pleural landmarks (soft tissue, ribs, rib shadows, and pleura – Fig. 10.10) should be identified in this area. If these findings are not seen, the probe should be moved inferiorly until an adequate window is achieved. Scanning of areas 2 and 3 may be performed based on the clinical suspicion for PTX. Imaging of areas 3 and 4 in the supine patient requires the patient to be placed in the lateral decubitus position and/or the use of a short probe to access the chest between patient and hospital bed.
