Fig. 8.1
Anatomy of the arterial vessels. 1. main trunk of pulmonary artery; 2. right pulmonary artery; 3. left pulmonary artery;
The main anatomic variants of the arterial pulmonary system are represented by the anomalous origin of the left pulmonary artery and by the congenital hypoplasia of the pulmonary artery.
Anomalous origin of the left pulmonary artery is a rare vascular developmental anomaly in which there is a regression of the left pulmonary artery during embryonic life which then arises from the posterior portion of the right pulmonary artery and passes between the trachea and esophagus to reach the left hilum. It may be isolated, often asymptomatic, or associated with other cardiovascular and bronchial anomalies (15%), and has a high mortality and morbidity rate during childhood. In asymptomatic cases it may mimic a mediastinal mass on chest radiography. Symptoms such as expiratory stridor, inspiratory and expiratory wheezes, and recurrent pneumonia are seen at birth in 60% of cases. Treatment is surgical (the vessel is ligated and re-implanted in the correct position).
Pulmonary artery hypoplasia is the most common congenital anatomical variant; it is caused by a reduced caliber of the lobar and segmental branches of the pulmonary artery without involvement of the main trunk. Symptoms are related to overload of the right heart. In patients with preserved heart function follow-up is suggested for evaluation during growth, while patients with advanced symptoms require lung transplantation.
8.2 8.2 Anatomy and Anatomical Variants of the Pulmonary Veins
Partial Anomalous Pulmonary Venous Return (PAPVR)
Venous pulmonary anatomy is shown in Figure 8.2 and Table 8.1. and their principal numeric variants are summarized in Figure 8.3. Partial anomalous pulmonary venous return (PAPVR) is a pulmonary-systemic left-to-right shunt caused by drainage of one or more pulmonary veins into the right heart or the great vessels draining into the right atrium, such as the superior cava vein (SCV), azygos vein, venous brachiocephalic trunk, inferior cava vein (ICV), or coronary sinus.
Table 8.1
Normal anatomy of the pulmonary veins
Left superior pulmonary vein (green) |
Right superior pulmonary vein (blue) |
Left inferior pulmonary vein (yellow) |
Right inferior pulmonary vein (violet) |
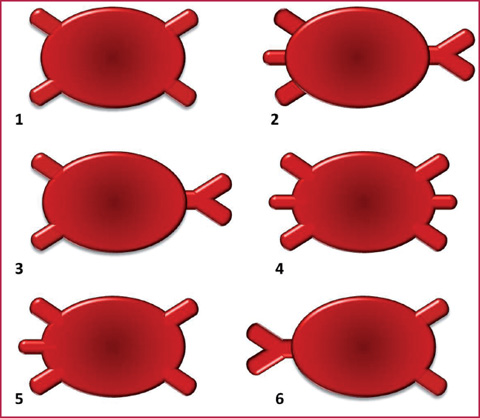
Fig. 8.3
Principal numerical anomalies of the pulmonary veins: 1. normal; 2. three right veins and one left main trunk; 3. two right veins and one left main trunk; 4. three right veins and three left veins; 5. three right veins and two left veins; 6. one right vein and two left veins
Symptoms occur in case of large shunts or in presence of other cardiac or pulmonary anomalies. Usually this anomaly is associated with interatrial septal defects (venous-sinus type).
The most common types are drainage of the superior right lobe into the SCV and drainage of the superior left lobe into a mediastinal vein that drains into the left brachiocephalic trunk. This vessel appears similar to a left SCV passing laterally to the aortic arch and descending to the heart.
CTA enables detection of this anomaly but cannot give any functional information like echocardiography and MRI. Cardiac MRI and MRA enable evaluation of the anatomy, quantification of the left-to-right shunt, volume, and right ventricular function, and show associated anomalies such as interatrial defects (Figs. 8.4–8.5). Surgery is mandatory in patients with a pulmonary to systemic flow ratio > 1.5 due to their high probability of developing pulmonary hypertension and right ventricular failure. Surgery may include the application of an autologous pericardial patch or other devices placed through the interatrial septal defect and bringing pulmonary veins into the left atrium. In the case of veins draining into the ICV, surgical correction including ligature of the veins and reastomosis to the left atrium may be required.
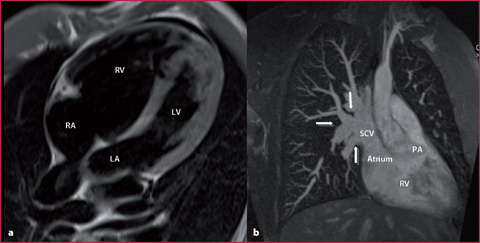
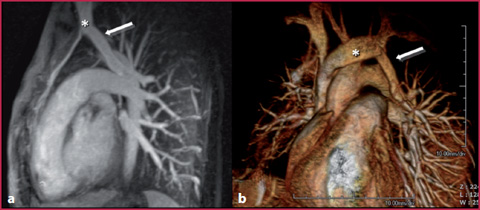
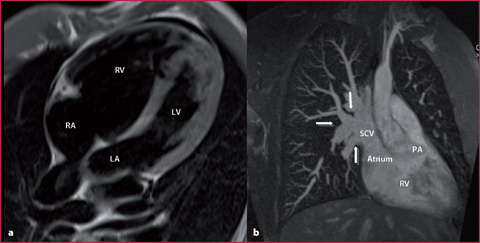
Fig. 8.4
35-year-old man with undetermined severe enlargement of the right ventricle during echocardiography. a T1 weighted sequence in four-chamber view confirms the enlargement. b Maximum Intensity Projection (MIP) reconstruction obtained using MR angiography proves the confluence of different pulmonary venous vessels into the superior vena cava. PA, pulmonary artery; RV, right ventricle; LV, left ventricle
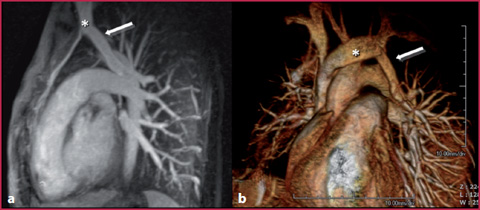
Fig. 8.5
20-year-old woman affected by Turner syndrome underwent MRA to evaluate the thoracic aorta. MIP (a) and VR (b) reconstructions showed the confluence of the left superior lobar pulmonary vein (arrow) into the homolateral brachiocephalic trunk (*) as a collateral finding
Total Anomalous Pulmonary Venous Return (TAPVR)
Total Anomalous Pulmonary Venous Return (TAPVR) is a rare anomaly (2% of cardiac malformations) occurring when the pulmonary veins fail to drain into the left atrium and form an unusual connection with some other cardiovascular structures (Fig. 8.6). TAPVR is classified in four types (Table 8.2).Infants affected by TAPVR may show cyanosis and congestive heart failure; one third of them have other associated cardiac lesions, in particular heterotaxy syndrome and asplenia.
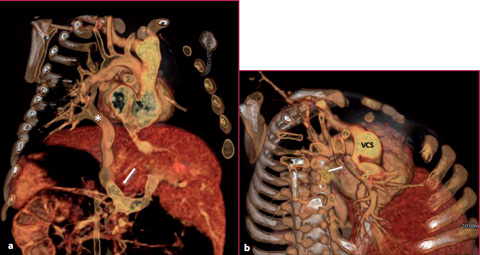
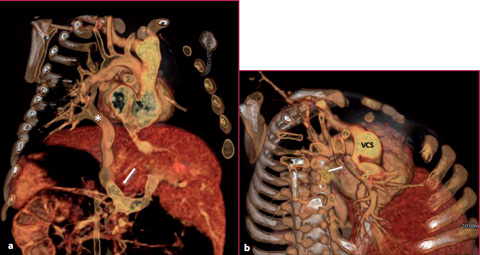
Fig. 8.6
Infant with severe dyspnea. VR reconstruction obtained with CTA shows (a) the confluence of the left pulmonary veins into a large venous trunk (*) and into the portal vein (arrow), while (b) the right pulmonary veins converge into a large venous trunk (arrow) and into the superior vena cava
Table 8.2
Types of TAPVR
Type I | Supracardiac (55%): usually four pulmonary veins converge behind the left atrium and form a common vein, know as the vertical vein that drain to the left or right brachiocephalic vein or the azygos or the superior vena cava |
Type II | Cardiac (30%): pulmonary veins drain into the coronary sinus or into the right atrium |
Type III | Subdiaphragmatic (13%): pulmonary veins drain into the ICV or into the portal vein |
Type IV | 2 or more of the previous levels: the vertical vein drains into the left innominate vein, and the anomalous vein or veins from the right lung drain into the right atrium or the coronary sinus |
CTA appearance is variable and related to the site of anomalous drainage and to the degree of obstruction.
The structure in which the anomalous vein ends usually appears dilated; the right heart chambers are enlarged because both the systemic and pulmonary veins drain into it and the left atrium is normal.
Survival is possible only in the presence of an interatrial septal defect or patent foramen ovale, which causes a right-to-left shunt.
Surgical correction includes anastomosis of the venous collector to the posterior wall of the left atrium. In the case of supracardiac anomalous venous pulmonary return a latero-lateral connection between the confluence of the pulmonary veins and the posterior wall of the left atrium must be created. If the anomaly is at the cardiac level, the obstruction is removed by pulling down the roof of the coronary sinus and placing a patch between the coronary sinus and pulmonary vein.
In the case of infracardiac anomalous pulmonary venous return, a latero-lateral anastomosis between the confluence of the pulmonary veins and posterior wall of the left atrium must be created.
8.3 8.3 CTA Technique
Patient Preparation
Supine position;
Peripheral venous access using a 18–20 G needle, preferably in the right arm; The patient should wear no metallic items.
Image Acquisition
Acquire topogram on a coronal plane and place the scan volume from the jugular notch to the end of the diaphragmatic dome;
Place the Region of interest (RoI) at the level of the main pulmonary trunk (if a bolus tracking technique is used) and start the scan when a 40 Hounsfield Unit (HU) value is reached;
Acquire images in the caudocranial direction.
To obtain adequate opacification of the pulmonary vessels it is essential to use:
a high flow rate (at least 4mL/s);
a high concentration of an iodinate contrast agent;
a quick scan duration.
By using a CT scanner which is slower than new-generation scanners, the duration of contrast agent administration should be equal to the scan duration; scanning should start no later than 4–6 seconds after the bolus injection, i.e. the moment at which the threshold value is achieved (recommended value 40 HU) (Tables 8.3-8.4).
Table 8.3
Technical parameters used with different CT scanners
4 MDCT | 16 MDCT | 64 MDCT | 128 MDCT | Dual source | |
---|---|---|---|---|---|
kVp | 120 | 120 | 100 | 100 | 100 |
mAs | 100-130 | 100-140 dose modulation | 150 dose modulation | 150 dose modulation | 150 dose modulation |
Collimation | 4 × 2.5 | 16 × 0.75 | 64 × 0.6 | 64 × 2 × 0.6 | 64 × 2 × 0.6 |
Slice thickness (mm) | 3 | 1 | 1 | 1 | 1 |
Recon increment (mm) | 1 | 1 | 1 | 1 | 1 |
Table 8.4
Contrast medium administration using different CT scanners
Cm | 4 MDCT | 16 MDCT | 64 MDCT | 128 MDCT | Dual source |
---|---|---|---|---|---|
Concentration (mgl/mL) | 400 | 400 | 400 | 400 | 400 |
Cm volume (mL) | 90-110 | 70-110 | 70-90 | 70-90 | 70-90 |
Saline flush volume (mL) | 50-50 | 50 | 50 | 50 | 50 |
Flow velocity (mL/s) | 3.7 | 3.7 | 3.7 | 3.7 | 3.7 |
When using a faster CT scanner, the use of a higher threshold value (60–80 HU) or a longer delay after reaching the threshold value of 40 HU is recommended to obtain better timing between contrast agent administration and the start of scanning.
If the study is positive for pulmonary embolism, scanning can be extended into a venous phase to the inferior limbs in search of deep vein thrombosis (DVT). In this case scanning may be extended to the pelvis and popliteal region, and the presence of DVT may be detected 3 minutes after the bolus as a luminal defect in the vessel.
8.4 8.4 MRA Technique
Patient Preparation
Supine position, arms along the body and electrodes on the thorax for cardiac gating;
Peripheral venous access (22–20 G), preferably in the right arm to avoid artifacts during the passage of the contrast medium into the left venous brachiocephalic trunk;
Remove all metallic items from the thorax region;
Superficial phased-array coil.
Image Acquisition
White-blood sequences (2D or 3D) acquired while holding one’s breath, in which it is not possible to distinguish between arterial and venous vessels (Table 8.5).
Table 8.5
Technical parameters of bSSFP sequences
TR (ms) | 337.1 |
TE (ms) | 1.22 |
Flip angle | 80° |
Field of view (mm) | 340 x 340 |
Slice thickness (mm) | 4-8 |
Matrix 256 x | 168 |
Balanced-Steady State Free Precession (bSSFP) Sequences
These sequences enable an overview of the entire thorax with coronal and axial acquisition and selection of the region to study with the contrast medium.
Acquire a localizer on three planes;
Place the field of view on the coronal and axial planes.
3D Gradient-Echo (GRE) Sequences
These sequences combine high spatial resolution (high matrix value) with thin slice thickness (1 mm) and minimum acquisition time (Table 8.6).Also in this case, digital subtraction of the post-contrast images from the pre-contrast mask is possible (Fig. 8.7).
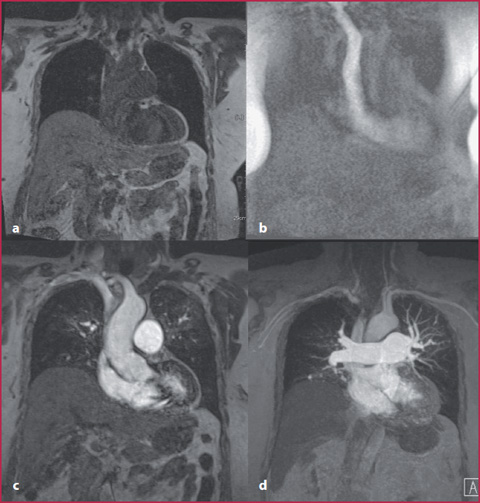
Acquire a localizer on three planes;
Acquire a pre-contrast mask (GRE 3D) on a coronal plane (the fewer the number of images, the shorter the acquisition time);
Observe the arrival of the contrast medium using the MR-fluoro technique (multiple images at a low spatial resolution of the thorax to visualize contrast medium arrival in real time);
Acquire post-contrast sequences (GRE 3D, the same as for the mask) when the contrast medium is in the right atrium.
Table 8.6
Technical parameters of GRE T1 3D sequences
TR (ms) | 2.58 |
TE (ms) | 1.08 |
Flip angle | 15° |
Field of view (mm) | 400 × 400 |
Slice thickness (mm) | 1.5 (gap 20%) |
Matrix | 448 x 176 |
Acquisition time (s) | 17 |
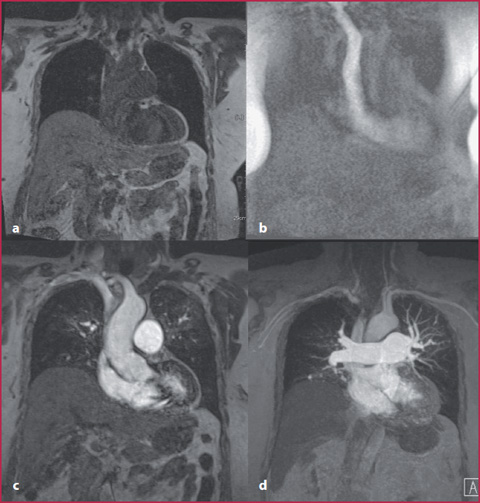
Fig. 8.7
a Pre-contrast GRE T1 coronal mask. b Fluoro-MR with real-time visualization of the CM. c Post-contrast GRE T1 sequence. d Post-contrast T1 weighted image with subtraction of the pre-contrast mask
Time-Resolved Sequences
These sequences enable dynamic evaluation of the same vascular region during all phases after contrast medium administration (Fig. 8.8, Table 8.7). They are useful in the evaluation of pulmonary arteriovenous malformation and in the follow-up of pulmonary embolism, particularly in patients with overlapping pulmonary hypertension.
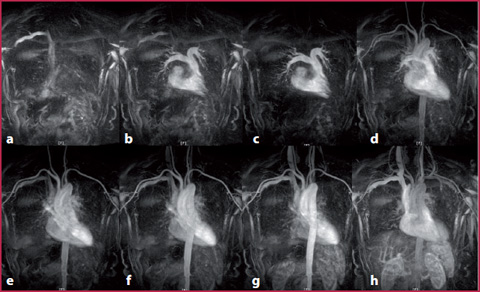
Acquire localizer on three planes;
Acquire a pre-contrast mask on a coronal plane;
Inject contrast medium and acquire images at the same time (while holding expiratory breath);
Optional post-processing subtraction of post-contrast acquisition from the pre- contrast mask allows MIP reconstructions that facilitate image evaluation. Contrast medium parameters are summarized in Table 8.8.
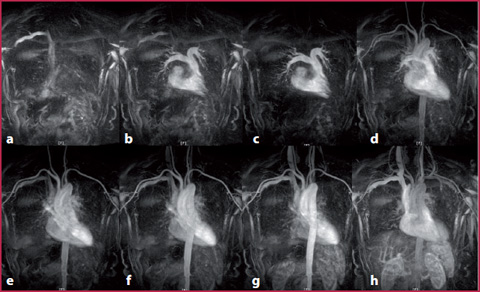
Fig. 8.8
It is possible to see the contrast medium arrival in the superior vena cava (a), at the level of the pulmonary vessels (b, c), into the arterial vessels (d–f), in the venous phase, (g) and during recirculation
Table 8.7
Parameters of time-resolved sequences
TR (ms) | 2-5 |
TE (ms) | 1-2 |
Flip angle | 20-50° |
Table 8.8
Parameters for contrast medium administration
Cm concentration | 0.5 M |
Cm volume (mL) | 10 (circa 0.2 mL/kg) |
Saline flush volume (mL) | 20 |
Flow velocity (mL/s) | 1.5-2 |
8.5 8.5 Pulmonary Embolism
Clinical Picture and Diagnosis
Pulmonary embolism occurs due to the migration of solid, liquid or air clots from a peripheral site through the venous system, or from the right heart into one or more arterial pulmonary vessels.
It determines a total or partial reduction of blood supply with secondary effects such as respiratory and circulatory alterations and may cause hemorrhagic infarction of the lung parenchyma.
It is the third cause of death among acute cardiovascular diseases after myocardial infarction and stroke with an incidence of 1.5/1000 per year and a mortality rate of 10-30% if not identified and treated.
The most common causes of pulmonary embolism are DVT and all other conditions that may cause clot formation such as:
Diagnosis is critical for treatment and prognosis, but sometimes clinical identification is difficult because of other related factors and symptoms.
slow venous flow;
coagulation disorders;
altered vessel walls.
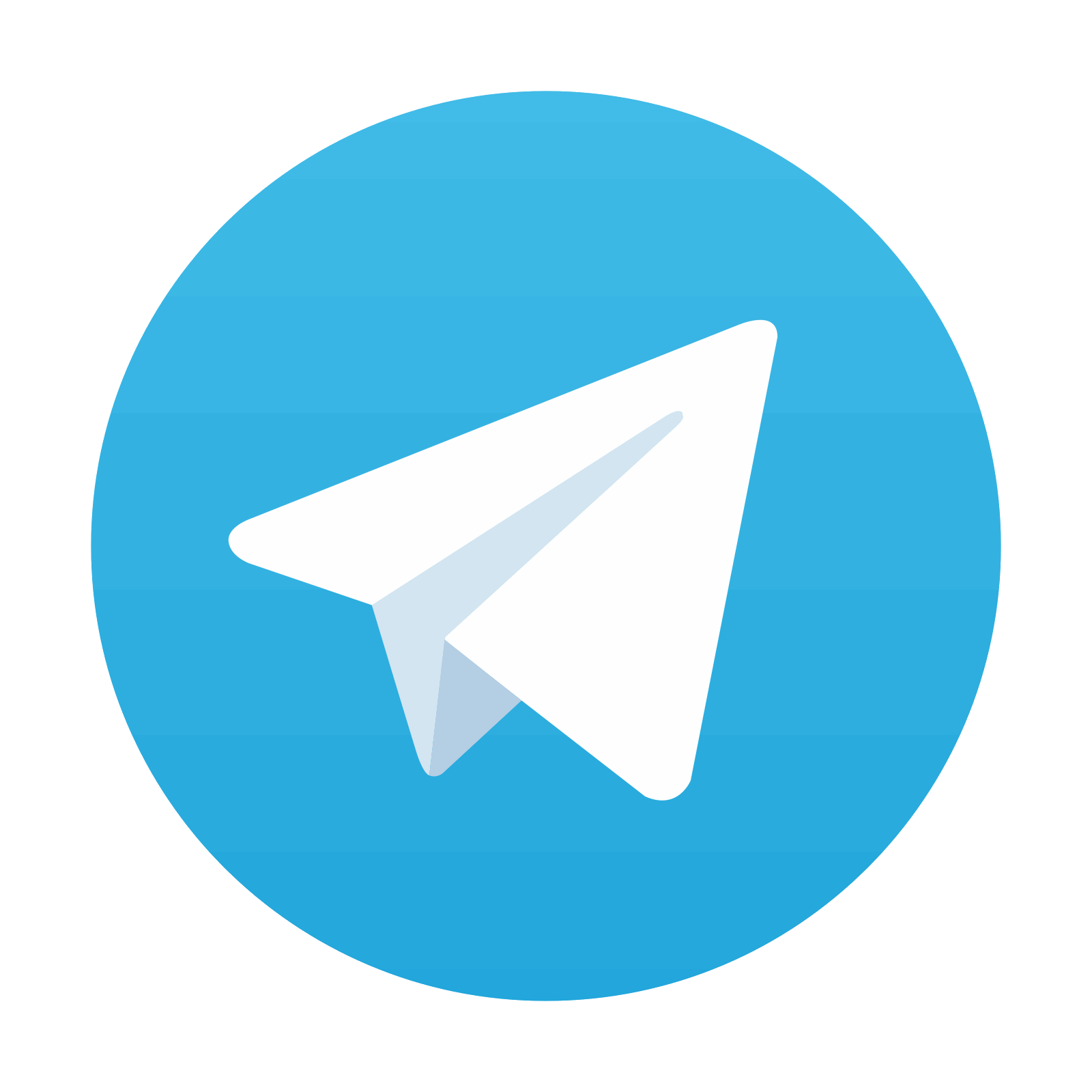
Stay updated, free articles. Join our Telegram channel

Full access? Get Clinical Tree
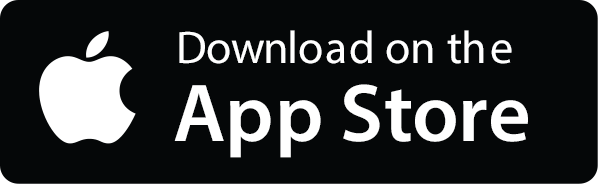
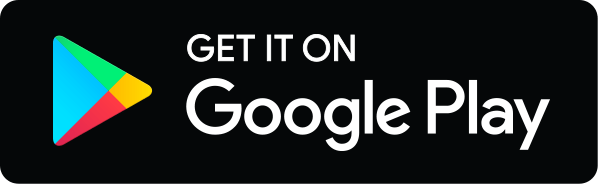