Fig. 28.1
Schematic representation of the sentinel lymph node concept. Assuming that lymph drainage from a solid tumor proceeds in an orderly way from lower-echelon to higher-echelon lymph nodes, the first node(s) encountered in such pathway, that is, the sentinel node(s), should be the site where tumor cells migrating through lymphatic channels are most likely to be entrapped and possibly originate metastasis before spreading to higher-echelon lymph nodes. As illustrated in the figure, even in any given lymphatic basin, there can be more than one sentinel lymph node, as lymph can drain from the site of the primary tumor via different lymphatic channels toward the same basin. The diagram also illustrates the complex interconnections that can exist at higher levels, with variable directions of lymph flow at intermediate levels within the general pattern of overall centripetal flow. The pattern of lymph flow is even more complex when considering that lymph from the tumor site can drain to more than one lymphatic basin, each one repeating the basic pattern represented here for a single basin
The term “sentinel lymph node” indicates the first lymph node encountered by lymphatic vessels draining the primary tumor, or the first lymph node upon which a lymph vessel originating in the tumor drains directly. This definition does not always correspond to the lymph node nearest the tumor, as the route of the lymphatic vessels is often tortuous and unpredictable. There may be different lymphatic pathways draining certain tumor sites, leading to different sentinel lymph nodes (Fig. 28.1); each sentinel node should therefore be investigated for the presence of metastasis. Lymphoscintigraphy following interstitial radiocolloid injection is critical to precisely visualize sequential lymphatic drainage of the radiopharmaceutical [15].
The presence or absence of sentinel lymph node metastasis usually has a significant impact on therapeutic strategy. In patients with early cancer, if the sentinel lymph node does not contain metastasis, the surgical approach should aim at removing the primary tumor and avoiding unnecessary regional node dissection. The likelihood that nonsentinel lymph nodes contain metastasis is extremely low, thus making extensive node dissection unnecessary in case the sentinel lymph node is free from metastasis. Patients whose sentinel lymph node contains metastasis usually require dissection of regional lymph nodes [16].
The first scientific evidence of the value of lymphatic mapping, the identification and characterization of lymph node drainage, was highlighted by Cabanas in 1977 with his early studies in patients with penile cancer. Cabanas identified the presence of a sentinel nodal group and showed that metastasis in these nodes significantly affects the prognosis [2]. Starting from Cabanas’s studies, the clinical use of lymphatic mapping with SLNB has gradually expanded. Unlike Cabanas, who identified a nodal basin to radically resect, subsequent investigators identified individual nodes in order to minimize dissection.
Instrumentation for Radioguided Surgery
The so-called gamma probe is used to count radioactivity in the surgical field intraoperatively, usually without producing any scintigraphic image but yielding both a numerical readout and an audible signal proportional to the counting rate. Recently, intraoperative imaging probes have become commercially available. The handheld probe (or detector component) is connected to a counting unit either with a cable or with a wireless system. The detector is usually of limited size, basically a long narrow cylinder with diameter varying from a minimum of about 12 mm up to a maximum of 16–18 mm. It may be slightly angled in order to allow for easier access in specific applications and easier manipulation within the surgical field. The gamma probe can be utilized in the surgical field because its outer surface is made of a material that can be sterilized (usually metal), or it can simply be covered with a sterilized wrapping (such as those used for intraoperative ultrasound probes). Through the digital readout and acoustic signal, the gamma probe enables the surgeon to precisely localize areas of maximum radioactivity accumulation, thus guiding identification and removal of the target tissue.
Different types of gamma probes are commercially available. They can be divided into two main categories: (a) crystal scintillation probes and (b) semiconductor probes (see also Chap. 3 of this book). Choice between these two main types of probes is based on several key operating parameters which may differ depending on the procedure [17–20]. For example, SLNB in patients with breast cancer or melanoma requires different parameters from those that are optimal for radioguided surgery of parathyroid adenomas. In addition, some technical features of the probe vary depending upon whether radiopharmaceuticals are labeled with 99mTc or other radionuclides, including positron-emitting radiopharmaceuticals [21–23]. The probe is connected to a small control unit, usually a laptop PC, with a flexible cable that may also be covered with sterilized wrapping, although Bluetooth-like connections have now become available, permitting the entire instrument’s easy use in the operating room. Besides setting the most effective energy window for detection/counting depending on the radionuclide employed, the control unit can adjust several acquisition parameters such as the time and mode of radioactivity counting and can display the radioactivity counting rate in various digital readout formats. At the same time, the unit usually emits an audible signal, the pitch/tone of which varies with the varying counting rate. The acoustic signal helps the surgeon to explore the surgical field without looking at the control unit display.
The main operating parameters characterizing a gamma probe are (a) sensitivity, (b) energy resolution, (c) spatial resolution, and (d) linearity of counting. Sensitivity is defined as the counting rate per unit of radioactivity and is usually expressed in counts per second (cps) per unit of radioactivity in kBq. KBq is used usually because of the small amount of radioactivity generally detected in radioguided surgery. This parameter is intrinsically linked to the efficiency of the probe in detecting radiation. Energy resolution is instead intrinsically linked to the counting statistics and to the ability to detect “true” counts arising in the target versus secondary scattered radiation.
Spatial resolution can be defined as the ability to identify very close radioactive sources as distinct from each other and depends critically on the collimation of the probe. Linearity of counting relates to the dead time and varies therefore for different detectors. A good gamma probe exhibits a counting linearity up to 4,000–5,000 cps.
As mentioned above, intraoperative probes specifically designed to detect the high-energy gamma rays originated by the annihilation process have become commercially available, thus making it possible to use radioguidance for resection of tumor lesions with high uptake of, for example, [18F]FDG or other tumor-seeking radiopharmaceuticals.
Nevertheless, major advantages in both preoperative and intraoperative localization of target tissue to be surgically resected (either it be the sentinel lymph node or tumor tissue previously labeled by local or systemic administration of suitable radiopharmaceuticals) have been made possible by the use of SPECT/CT and/or of intraoperative imaging probes. In fact, hybrid imaging with SPECT/CT supplies to the surgeon a set of anatomotopographic information that guides resection through the optimal surgical access according to the principle of least invasive surgery. As reviewed recently [24, 25] and as exemplified in Fig. 28.2, this approach is especially useful when planning surgery in complex anatomical regions such as the head and neck or the pelvis [26–33].
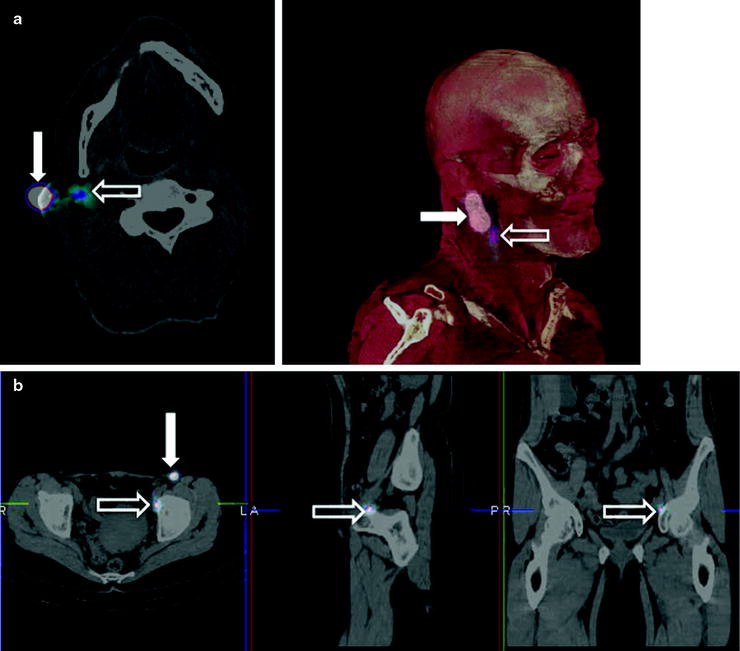
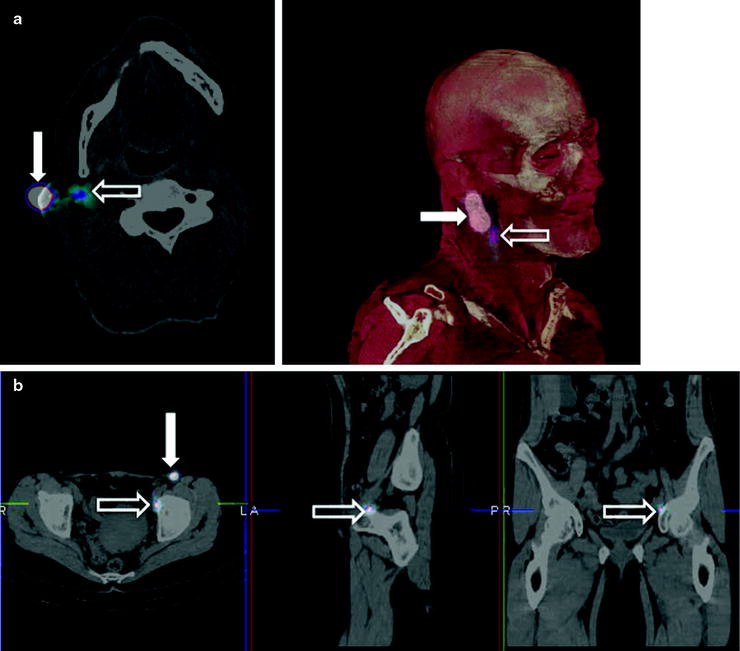
Fig. 28.2
Lymphoscintigraphic mapping with 99mTc-sulfur colloid. (a) SLNB postresection of a cutaneous melanoma in the right malar region. Transaxial-fused SPECT/CT section in left panel demonstrates migration to a deeply seated sentinel lymph node (white open arrow), deeply seated relative to the site of interstitial injection (white closed arrow). In the right panel, 3D surface volume rendering provides topographic coordinates for optimal planning of the most adequate surgical approach. (b) SLNB postresection of a cutaneous melanoma in the anterior left thigh. Fused transaxial SPECT/CT images (left panel) shows a deeply located sentinel lymph node within the pelvis (white open arrow) in addition to a superficial sentinel lymph node (white closed arrow). Additional topographic coordinates of the deeply seated sentinel node are provided by the sagittal and coronal sections (center and right panels, respectively). (From Mariani G, Bruselli L, Kuwert T, Kim EE, Flotats A, Israel O, Dondi M, Watanabe N. A review on the clinical uses of SPECT/CT. Eur J Nucl Med Mol Imag. 2010;37:1959–1985, with permission)
Evidence is also growing on the usefulness of intraoperative imaging probes for radioguided surgery, employing such instrumentation either during conventional open surgery or during laparoscopic surgery [34–36]. Real-time intraoperative imaging provided by this instrumentation improves monitoring of surgical excision of the target tissues (Fig. 28.3).
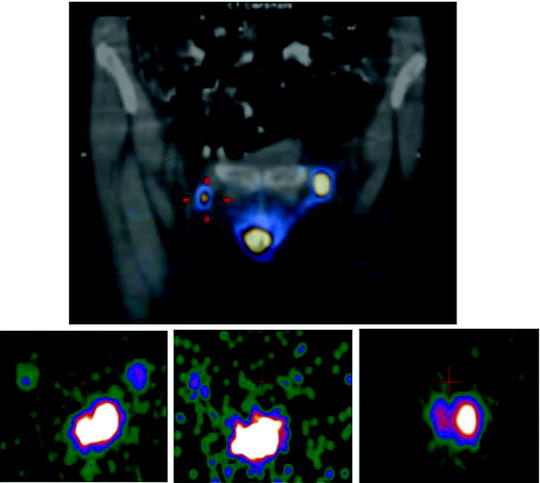
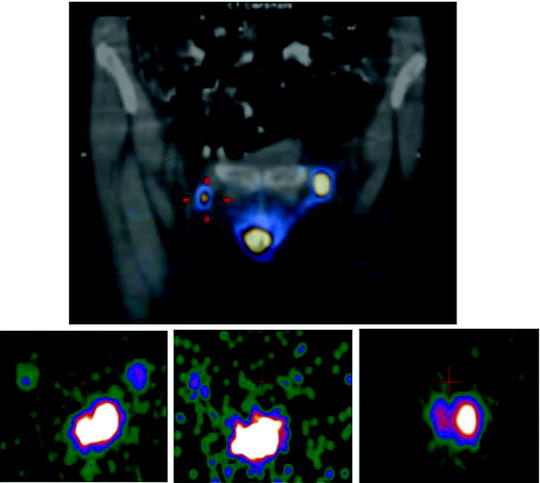
Fig. 28.3
Intraoperative imaging for radioguided SLNB in a patient with vulvar cancer. The upper panel shows the fused coronal SPECT/CT section obtained preoperatively, demonstrating migration of 99mTc-nanocolloidal albumin to both right and left inguinal sentinel lymph nodes; intense radioactivity accumulation in the middle lower field of view corresponds to the injection site, while sentinel node visualization is more intense in the left than in the right groin. Sequential real-time intraoperative images are shown in the bottom row, obtained respectively at the beginning of surgery (left panel, where both the injection site and the right and left sentinel nodes are clearly visualized), after harvesting the left inguinal node (center panel) and after removing also the right inguinal sentinel lymph node (right panel). (Courtesy of Dr. Renato A. Valdés Olmos, Nuclear Medicine, The Netherlands Cancer Institute, Amsterdam, The Netherlands)
A most recent interesting development in radioguided surgery is the system so-called freehand SPECT, in which a continuous positioning system installed in the operating room is based on a fixed pointing device, on the patient’s body, and respectively, on the handheld gamma counting probe. Position of the gamma probe relative to the fix device is tracked by infrared positioning technology, and the output of the intraoperative gamma probe is spatially coregistered in the surgical field (depicted by a video camera) and displayed on a monitor in which the surgeon can easily check location and depth of the foci of radioactivity accumulation to be resected [37]. An example of application of this novel approach is given in Fig. 28.4.
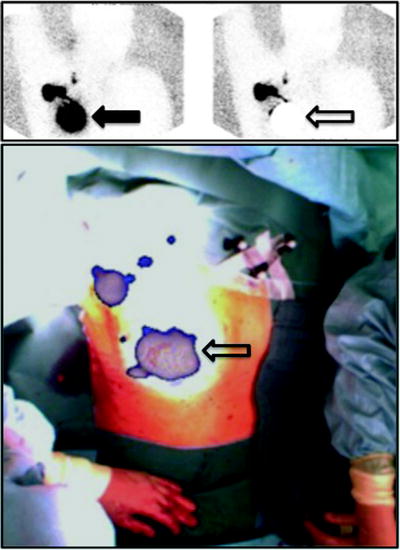
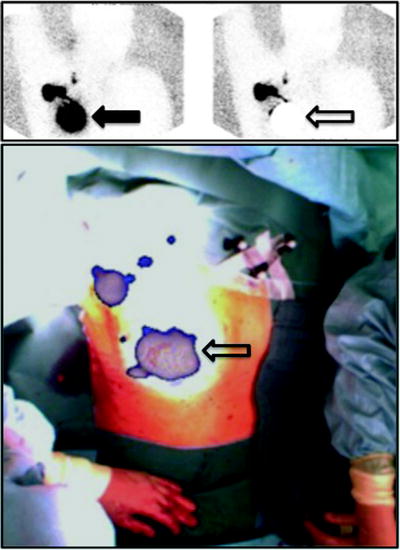
Fig. 28.4
Upper panel: planar scintigraphy (with flood phantom for body contour, see further below) obtained in a breast cancer patient about 10 min after injecting 99mTc-nanocolloidal albumin in 4 periareolar spots (closed arrow). Besides intense radioactivity remaining at the injection site (closed arrow), left image shows migration of the radiocolloid to at least 3 sentinel lymph nodes located in all 3 levels according to Berg. Right image was taken using a lead plate to cover the injection site (open arrow). Lower panel shows overlay of the freehand SPECT 3D image on the intraoperative video image of the same patient, for easier anatomical correlation. The sentinel lymph nodes located at all 3 levels according to Berg are clearly visualized relative to the anatomic landmarks of the patient. (Courtesy of Prof. Marion Kiechle, Women’s Hospital, and Prof. Markus Schwaiger, Nuclear Medicine Department, Klinikum rechts der Isar, Technische Universität München, Munich, Germany)
Radiopharmaceuticals for Radioguided SLNB
Lymphoscintigraphy is critical to most cases of radioguided SLNB. Imaging agents employed for lymphoscintigraphy are radiocolloids that, after their interstitial administration, allow the scintigraphic visualization of the lymphatic vessels draining the injection site. These colloids are preferentially retained by the first lymph node encountered along the course of the draining lymphatic vessel(s), by definition the sentinel node(s).
The general term “colloid” indicates a class of macromolecules of micellar size with similar physicochemical and biological behaviors. They are particles varying between about 5 and about 1,000 nm (0.005–1 μm) in size. Physiologic endogenous colloids are heterogeneous in composition, and their presence in circulating fluids can be constituted by fragments of bacteria, or lipidic micellae absorbed in the gastrointestinal tract, or cell debris produced during normal cellular tissue renewal. Endogenous colloids circulating in the lymph or blood share a common fate. In fact, they are removed from the circulation by macrophages, a ubiquitous clearing/purification system located in the bone marrow, spleen, liver, and in the sinusoidal spaces of lymph nodes.
Once within the lymph node through the afferent lymphatic channels draining the injection site, radiocolloids are phagocytized by macrophages lining the lymph node sinusoids. Such retention of radiocolloids in the sentinel lymph node is a physiological process, and it does not indicate presence or absence of metastases in the lymph node.
The speed of lymphatic drainage from the interstitial injection site and the amount retained in the sentinel lymph node depend mainly on the size of the radiocolloids, which may be either an inorganic substance (198Au-colloid, 99mTc-antimony sulfur, 99mTc-colloidal sulfur, 99mTc-stannous fluoride, 99mTc-rhenium sulfur) or derived from biological substances (nano- or microcolloidal human serum albumin). In particular, small size radiocolloids (less than about 100 nm) migrate quite fast from the injection site through the lymphatic system. They allow lymphoscintigraphy to be performed in a relatively short time usually (within 45–60 min after injection). However, they are not efficiently retained in the sentinel node, so that more than one lymph node, often as many as to 3–4, can typically be visualized along a single lymphatic draining channel. This may complicate the identification of the sentinel lymph node unless there are sequential images acquired at short time intervals after interstitial injection. Larger size radiocolloids are retained more efficiently in the sentinel lymph node, so that scintigraphy does not generally visualize more than 1–2 nodes along a single lymphatic draining vessel. The migration of larger particles from the interstitial administration site is very slow, so that a longer time, even 2 h or more, can be required to complete lymphoscintigraphy. The nuclear medicine physicians’ experience and the agent’s commercial availability affect choice of the radiopharmaceutical. 99mTc-albumin nanocolloid (that has a quite narrow range of particle size, with over 90% of the particles being smaller than 80 nm) is commercially available and most widely employed in Europe, while 99mTc-sulfur colloid (with a wide range of particle size between about 20 and 400 nm) is widely employed in the USA. To overcome the problem of heterogeneous particle size distribution, in some nuclear medicine centers, 99mTc-sulfur colloid is filtered immediately after labeling and prior to administration, in order to remove particles with a diameter greater than a certain size, usually 220 nm or other size [38, 39].
Radioguided SLNB in Breast Cancer
The sentinel lymph node hypothesis (see Fig. 28.1) is that lymph and tumor cells entering the lymphatic circulation migrate through the lymphatic vessels in an orderly manner to the first lymph node draining the anatomic region. From the breast, especially for superficial and outer quadrant tumors, drainage is toward the axilla but occasionally is toward the internal mammary chain, especially from deeply seated tumors in the inner quadrants. The breast embryologically originates from ectodermal tissue, as a skin appendage, and therefore shares a pattern of lymphatic drainage with the overlying skin. The mammary gland is interposed between the superficial (subdermal) and the deep (subcutaneous) lymphatic plexuses, the two systems being interconnected by a dense network of lymphatic vessels (Fig. 28.5a). Lymphatic vessels surrounding the mammary lobules predominantly drain to the subareolar Sappey’s plexus, which is part of the superficial plexus of the skin. Most of the lymph produced in the breast is therefore drained to the subareolar region, progressing then toward the ipsilateral axillary nodes (Fig. 28.5b). A small fraction of the lymph produced in the breast (about 3%) is instead drained to lymph nodes of internal mammary chain, while an even smaller amount drains to other lymph nodes such as the intercostal, pectoral muscles, contralateral breast, or even abdominal nodes [40].
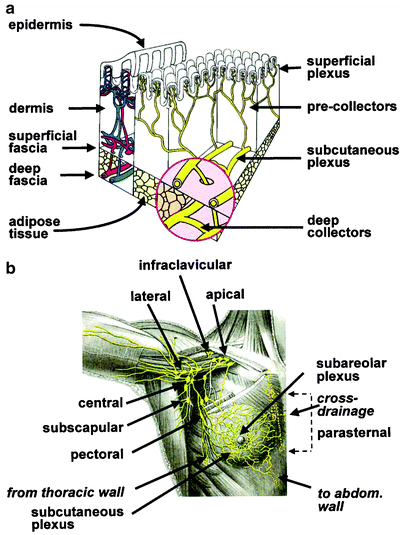
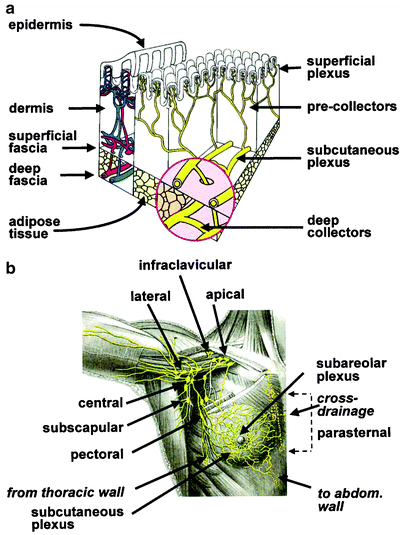
Fig. 28.5
(a) Schematic structure of cutaneous blood and lymph vessels. For easier comprehension, the lymph and blood vessel networks (that are actually embedded in each other) are represented separately, respectively, on the right (yellow) and on the left (red and blue). Due to its embryologic origin in the ectoderm, the mammary gland can be placed in an ideal space between the subcutaneous plexus and the deep lymphatic collectors (magnified insert in the figure). Branches of the periductal plexus drain lymph mostly toward the skin surface (via the subareolar plexus), while a minor component drains toward the deep collectors (that in turn drain toward the internal mammary chain). Radiocolloids injected intradermally over the mammary gland drain to the subcutaneous plexus that also receives most of the lymph draining from the mammary gland. (b) Pathways of the lymphatic vessels and lymph node stations draining the mammary gland. Most of the lymph produced in the breast drains to the subareolar plexus, then merges with the subcutaneous plexus of overlying skin, and flows mostly toward the axilla. Lymph from deeper portion of mammary gland drains either through the same pathway or through deep lymphatics that reach the parasternal, internal mammary chain (and even the contralateral side) abdom = abdominal. (From Mariani G, Moresco L, Viale G, et al. Radioguided sentinel lymph node biopsy in breast cancer surgery. J Nucl Med. 2001;42:1198–1215, with permission)
By following these routes of drainage, lymph and cancer cells entering the lymph space spread initially to the first node, which is the sentinel lymph node. If the sentinel node is free of metastasis, the probability of tumor cells skipping the sentinel node and metastasizing to second- or third-echelon lymph nodes is an exceptionally rare event [41–49].
SLNB has been an important step toward the adoption of less invasive surgical techniques. When the sentinel node is free of metastasis, the patient can be spared an axillary lymph node dissection that until a few years ago was considered the standard staging procedure for breast cancer. Axillary lymph node dissection itself has minimal impact, if any, on survival and therefore has little or no curative purpose; the main goal of this procedure is staging the N parameter in the axilla [50]. Along with other prognostic factors such as hormone receptor status, proliferative index, etc., the N stage is used to stratify patients for optimal postsurgical management. Because of low accuracy of the methods currently available for preoperative axillary nodal assessment, including ultrasound and even [18F]FDG-PET, surgical staging is necessary in most cases [51]. In early breast cancer, the fraction of patients with axillary lymph node metastases is only 20–30%; as many as 70–80% of axillary dissections reveal no metastasis and, therefore, could have been avoided. Axillary lymph node dissection results in a high incidence, about 25–30%, of postoperative complications that can reduce quality of life, such as delayed wound healing, lymphedema, peripheral nerve injury, or even brachial plexus injury. SLNB greatly decreases the number of patients who may experience such complications [52–55], by limiting node dissection to those patients in whom the sentinel node was found to harbor metastasis. Metastasis in sentinel lymph node(s) is usually treated with completion axillary dissection not because of curative intent but mainly to accurately stage the cancer and determine the number of involved lymph nodes and extent of their involvement.
Optimal conditions for deriving maximum benefit from successful SLNB are to be found in early breast cancer patients with clinically negative axilla and negative ultrasound examination. Mammographic screening can detect tumors with a diameter of about 1 cm (T1a-b), where the probability of metastasis in axillary lymph nodes is even <20%. The use of SLNB is also well established for patients with T1c tumors (diameter up to 2 cm), where the probability of lymph node metastasis is about 20–30%. Nevertheless, the procedure can be beneficial also in patients with larger tumors; therefore, contraindications to SLNB in patients with breast cancer are progressively decreasing, and also patients with larger tumors should be considered candidates for SLNB [56–59].
In addition to reducing the need for axillary lymph node dissection in patients with low probability of axillary metastasis (T1 tumors), SLNB is more accurate than conventional axillary dissection in detecting nodal disease [60]. With SLNB, histopathologic examination is limited to only one or very few lymph nodes, on average no more than two per patient, and the pathologist can more carefully examine the entire lymph node(s) using multiple sections and enhanced staining methods. In this manner, it is possible to detect very small metastatic foci <2 mm in size, or micrometastasis, and even smaller, isolated tumor cell clusters that could have been neglected in a conventional histopathologic examination of an entire axillary dissection, when about 15–25 nodes must be analyzed. Such greater accuracy in staging allows optimal planning of postsurgical adjuvant treatment.
Techniques of Radiopharmaceutical Administration
Lymphatic circulation within solid tumors (including breast cancer) is generally grossly abnormal, disrupted, and inefficient. This has relevance for the modality of interstitial radiocolloid injection technique for lymphoscintigraphy, in that intratumoral administration as was originally proposed is less logical than peritumoral injection. In fact, lymphatic circulation in the peritumoral area is normal and actually represents the entrance site to lymph vessels of cancer cells detached from the growing edge of the tumor which eventually give rise to lymph nodal metastasis. Nevertheless, additional radiocolloid injection modalities have been proposed and widely adopted in clinical practice [38].
Three main parameters define an optimal radiocolloid administration technique for lymphoscintigraphy and subsequent radioguided SLNB: injection site, injected volume, and injected activity. A fourth parameter to be taken into account is the time elapsed between injection (with subsequent lymphoscintigraphy) and surgery, as it specifically influences the amount of radioactivity to be injected.
Since the intratumoral space is almost devoid of an efficient lymphatic circulation, intratumoral radiocolloid administration requires the injection of high activities (up to 370 MBq or 10 mCi) and large volumes of the radiocolloid suspension (up to 4 mL). Injection of such large volumes can substantially increase interstitial pressure at the injection site, thus possibly altering the pattern of lymphatic drainage and forcing the radiocolloids to enter drainage routes different from those prevailing in baseline conditions. Furthermore, most of the injected radioactivity is retained at the injection site, often causing interference—the so-called “shine-through” effect—in scintigraphic and intraoperative sentinel lymph node detection. Finally, the slow drainage of radiocolloids from the tumor can cause poor scintigraphic visualization of the lymphatic channels and possibly lead to failure of lymphoscintigraphic imaging and of intraoperative identification of the sentinel lymph node.
All the above considerations explain why, at present, most nuclear medicine centers prefer the peritumoral injection (ultrasound-guided) or intra/subdermal injection over the intratumoral route of radiocolloid administration. The technique of periareolar injection is also increasing in popularity [61–63].
The rationale of intra/subdermal administration stems from the fact that lymph is drained from the intra/subdermal space to the subcutaneous plexus, which is the merging point for lymph originating from the underlying breast parenchyma (see Fig. 28.5a). Thus, a radiocolloid injected intra/subdermally displays the same pathways of lymphatic drainage as the underlying breast gland and of cancer cells entering the lymphatic space. An important advantage of intra/subdermal injection is that radiocolloids migrate much faster from the administration site to lymph nodes compared to peritumoral injection and far more rapidly than the extremely slow migration after intratumoral injection. Furthermore, ultrasound guidance is not required for injection, as is often required for performing peritumoral injection. Similar considerations apply to the periareolar route of radiocolloid administration. The rationale for this technique is the fact that the lymph produced in the breast flows to the periareolar Sappey’s plexus, before draining to axillary lymph nodes (see Fig. 28.5b). An important advantage of this modality of radiocolloid injection is that it can be easily performed even in cases of nonpalpable or multifocal tumors [61, 63].
Peritumoral radiocolloid administration is usually performed by depositing two aliquots on each side of the tumor, each one of 0.1–0.5 mL containing 37–74 MBq of the radiopharmaceutical. Tumor location and injection sites may be identified by ultrasound and/or x-ray stereotactic guidance.
Intra/subdermal radiocolloid administration is performed on the skin overlying the tumor, in such a way so as to produce a small wheal. The injected volume is approximately 0.1–0.3 mL, containing about 37–74 MBq of radiocolloid, and in some nuclear medicine centers, more than one injection is performed in adjacent sites. Such intra/subdermal route of radiocolloid administration is generally preferred in the case of superficial, easily palpable tumors, especially if located in the outer quadrants of the breast.
Periareolar radiocolloid administration is generally performed with four injections of 25–30 MBq in 0.1–0.3 mL, each one at the edge of the areola at Sappey’s plexus. In some nuclear medicine centers, only two aliquots are injected, approximately on each side of the areola. Periareolar injection is often preferred for nonpalpable tumors.
Regardless of the injection site, after injection, the patient is asked to lightly massage the breast to facilitate lymphatic drainage of radiocolloids.
After intra/subdermal or periareolar radiocolloids administration, lymphatic drainage and subsequent lymph nodes visualization is usually quicker than after peritumoral injection (20–30 min compared to 2–3 h on average). After 15–18 h, during surgery, radiocolloids migrated to lymph nodes represent about 1% of the injected activity after intra/subdermal administration, while it is about 0.1% after peritumoral administration.
From the technical point of view, the easiest modality of radiocolloid injection is either the intra/subdermal or the periareolar route, after which scintigraphic visualization of lymphatic drainage is readily feasible and efficient, including dynamic acquisitions. In the published literature, there are no reports of significant differences in outcome between the intra/subdermal, the periareolar technique, and the peritumoral technique, except for differences in the frequency of visualization of lymph drainage to the internal mammary chain [64, 65]. After peritumoral administration, lymphoscintigraphy shows drainage to the internal mammary chain in 20–30% of the cases, while this fraction is much lower (3–5%) after intra/subdermal or periareolar administration. In some centers, the two different techniques are employed together in the same patient, while in other centers the intra/subdermal or periareolar route injection is preferred for superficial tumors and the peritumoral route for deeply seated tumors.
Lymphoscintigraphy
Lymphoscintigraphy is performed in most nuclear medicine centers as an integral, mandatory step for radioguided SLNB. It is generally performed in the afternoon of the day preceding surgery if the operation is scheduled in the early morning, or on the same day 4–6 h prior to surgery, depending on logistics of the institution. For same day injection, less radiocolloid is generally used (15–20 MBq) compared to prior day injection (37–74 MBq). Lymphoscintigraphy is particularly useful to identify not only axillary sentinel lymph node(s) as a guide to subsequent removal aided by intraoperative gamma probe counting, but also other possible axillary lymph nodes as well as draining nodes in other lymphatic basins, especially the internal mammary chain or even intramammary lymph nodes [66–68].
The gamma camera energy selection peak is centered on the 140 keV of 99mTc (±10% window), and the use of high-resolution collimator(s) and a 256 × 256 acquisition matrix is preferred. A pinhole collimator may occasionally be used to improve spatial resolution.
The patient is positioned supine with her arms raised above the head, and the collimator is placed as close as possible to the axillary region. Anterior, anterior oblique, and lateral images are acquired. A 57Co flood source can be positioned between the patient’s body and the collimator in order to obtain some reference anatomic landmarks in the scintigraphic image (see Figs. 28.4 and 28.6). Alternatively, the body contour can be identified by moving a 57Co point source during scintigraphic acquisition (see Fig. 28.7). The use of SPECT/CT acquisitions obviates the problem of identifying anatomic landmarks as a reference for topographic location of the sentinel lymph node(s) (see Fig. 28.8) [69–71].
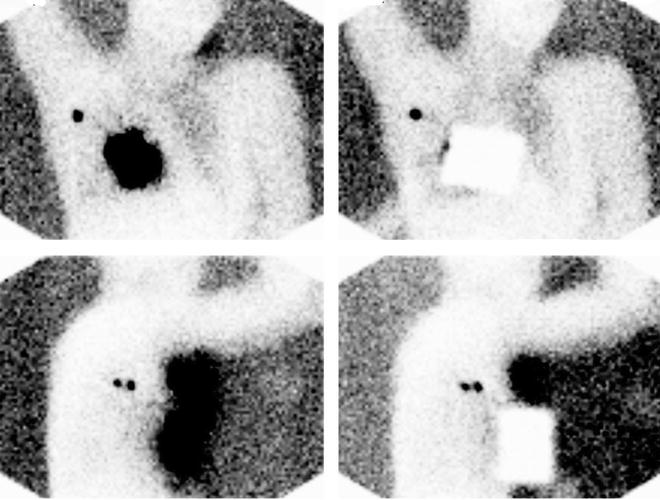
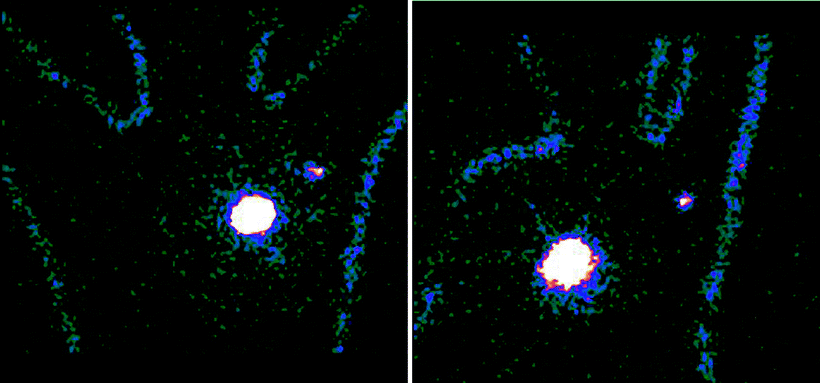
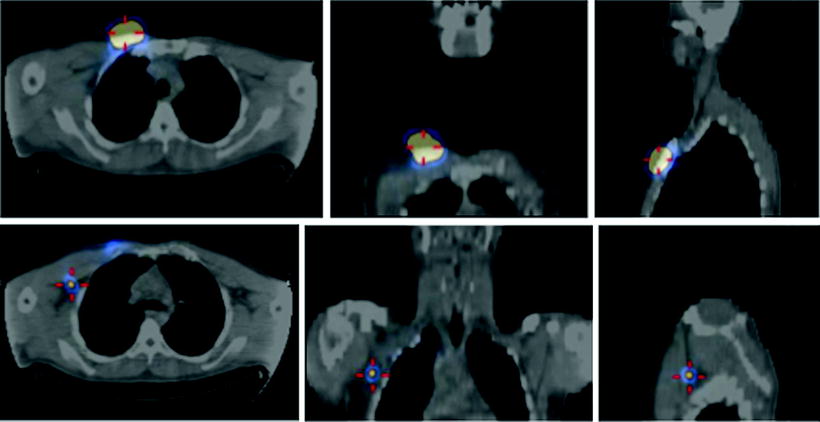
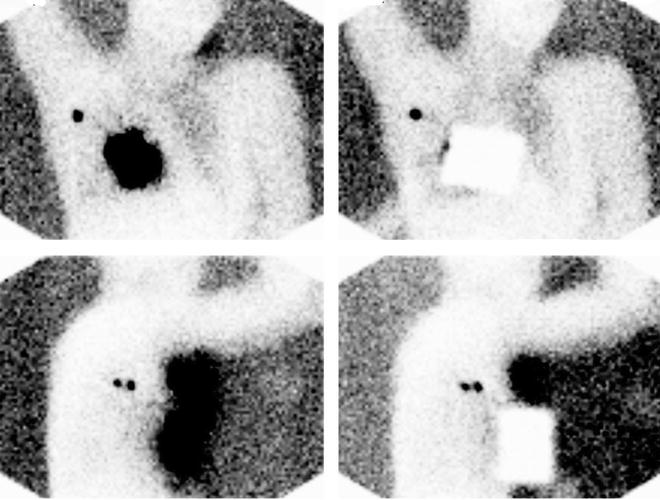
Fig. 28.6
Planar lymphoscintigraphy of breast cancer patient obtained between 10 and 20 min after injecting about 50 MBq of 99mTc-nanocolloidal albumin periareorally in a patient with cancer of right breast. Upper row shows anterior projections and lower row lateral projections. Right images were taken using a lead plate to cover the injection site. All images were acquired using a single-head gamma camera and a high-resolution, low-energy collimator (acquisition time 2 min). The 57Co flood phantom placed opposite to the gamma camera head produces the body contour delineation. While one single axillary sentinel lymph node is visualized in the anterior projection, two nodes are clearly visualized in the lateral projection. (Courtesy of Dr. Andreas K. Buck, from the Nuclear Medicine Department at Klinikum rechts der Isar, Technische Universität München, Munich, Germany)
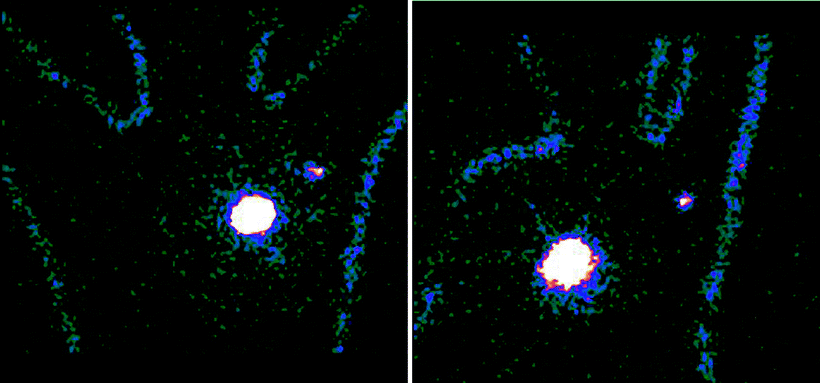
Fig. 28.7
Body contour delineation obtained by moving a 57Co point source along body of the patient during acquisition of the planar scintigraphic images. In this patient with cancer of the left breast, 99mTc-nanocolloidal albumin was injected at 4 spots periareorally. Images acquired both in the anterior projection (upper panel) and in the left anterior oblique projection (lower panel) visualize migration of the radiocolloid to a single sentinel lymph node in the axilla
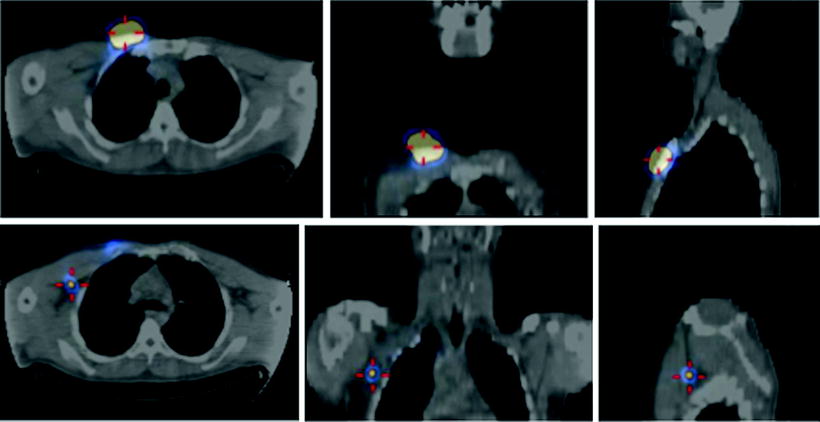
Fig. 28.8
Fused SPECT/CT images obtained in a male patient with cancer of the right breast after periareolar injection of 99mTc-nanocolloidal albumin. Top row: transaxial, coronal, and sagittal sections (left, center, and right panel, respectively) show the site of radiocolloid injection. Bottom row: transaxial, coronal, and sagittal sections (left, center, and right panel, respectively) show lymphatic drainage of the radiocolloid to a sentinel lymph node located deeply in the right axilla
When expecting a relatively fast kinetics of lymphatic migration from the injection site, that is, when using a radiocolloid with small size particles such as 99mTc-nanocolloidal albumin and/or the intra/subdermal or periareolar administration route, sets of 3- to 5-min (or 300,000–500,000 k-counts) static images are generally acquired sequentially, about every 10 min, starting about 10 min after radiocolloid injection and up to clear scintigraphic visualization of the sentinel lymph node(s). Dynamic acquisition can occasionally be useful [72], for example, 1 image every 30 or 60 s for 30 min, with matrix 64 × 64 or preferably 128 × 128, in order to better assess the pattern of radiocolloid drainage within the breast; this procedure is especially useful in the learning phase of radioguided SLNB, in order to become more confident with the lymphoscintigraphic technique. Figure 28.9 shows different patterns of lymphatic drainage and distribution of sentinel lymph nodes obtained in preparation for radioguided biopsy.
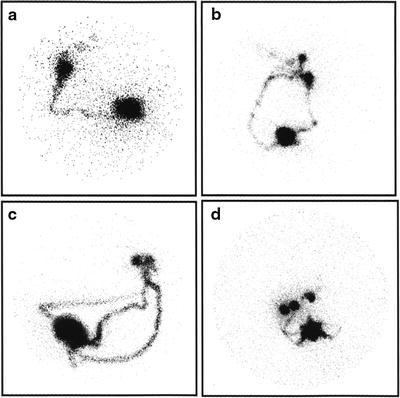
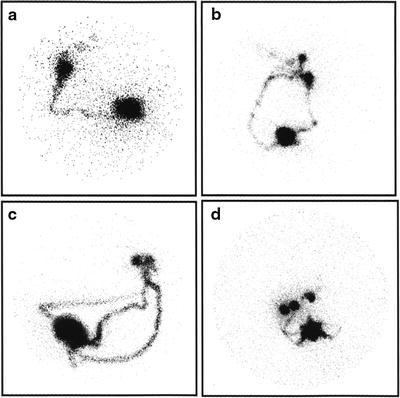
Fig. 28.9
Variable lymphoscintigraphic patterns visualized between 30 and 60 min after intradermal injection of 99mTc-nanocolloidal albumin in patients with breast cancer. (a) Single lymphatic vessel leading to a single sentinel lymph node, with faint visualization of subsequent-tier lymph nodes (right anterior oblique view). (b) Two separate lymphatic vessels widely diverging in their initial pathway, eventually leading to two separate but adjacent sentinel lymph nodes, with faint visualization of subsequent-tier nodes (left anterior oblique view). (c) Three separate lymphatic vessels widely diverging in their initial pathway, eventually leading to two separate but very close sentinel nodes (left anterior oblique view). (d) Multiple lymphatic vessels leading to at least three separate sentinel lymph nodes (right anterior oblique view). (From Mariani G, Moresco L, Viale G, et al. Radioguided sentinel lymph node biopsy in breast cancer surgery. J Nucl Med. 2001;42:1198–1215, with permission)
At the end of lymphoscintigraphy, cutaneous projection of the sentinel lymph node is marked with a dermographic pen. External gamma probe counting can be used to confirm correct localization of the sentinel lymph node(s), taking care to position the patient’s arm in the same position as it will be placed during surgery.
Intraoperative Gamma Probe Counting
Just before starting surgery and with the patient already positioned on the operating table, the gamma probe is initially utilized to scan the axilla or any other region where radiocolloid accumulation has been visualized in order to confirm correct identification and localization of the sentinel lymph node(s). Intraoperatively, the probe is then introduced through the skin incision to explore the presumed location of the sentinel nodes that are usually easily identified on the basis of target/background count rates >10:1. After removing the lymph nodes, the operative field is explored again with the probe to assess residual radioactivity. It is recommended to resect any other radioactive lymph node yielding count rates higher than about 20% (or 10% according to some investigators) of the count rate seen in the first lymph node removed which is usually the most radioactive. The sentinel lymph node and any other nodes so identified are then sent for complete histopathologic analysis. The count rates obtained with the gamma probe during surgery may be recorded in a special form or stored in electronic format. Palpation of the axilla should be performed and any suspicious nodes resected.
Once the learning phase of radioguided SLNB has been completed (see below), the success rate of lymphoscintigraphy and intraoperative gamma probe counting in identifying sentinel lymph node(s) is about 96–97% in experienced centers. This value is greater than that commonly experienced using blue dye alone (75–80%), while combining radioguidance with the blue dye leads to a 98–99% success rate in sentinel lymph node identification [38, 73]. A possible advantage of the combined technique is where macrometastasis in the sentinel lymph node may inhibit radiocolloid accumulation if tumor cells have replaced much of the normal lymph node tissue [74].
Analysis of Sentinel Lymph Nodes
Histopathologic analysis of sentinel lymph nodes is extremely effective and can detect the presence of micrometastasis (≤2 mm) and clusters of several cells or even isolated tumor cells [60]. By focusing on only a few lymph nodes rather than on 15–20 nodes as generally harvested during an axillary dissection, the pathologist can completely dissect and examine at 50–100 μm intervals each sentinel node. Sections may be stained with hematoxylin and eosin (H&E) for conventional light microscopy, and if such examination is negative, adjacent sections may be used for immunohistochemistry usually with antibodies against cytokeratin.
More recently, highly sensitive molecular analysis based on the polymerase chain reaction (PCR) has been introduced to detect the presence of mRNA specific for breast epithelium or cancer in sentinel nodes [75–77]. Although automated methods and instrumentation have already been introduced to the market, molecular analysis of sentinel lymph nodes is not yet routinely performed.
Accurate histopathological examinations, including immunohistochemical analysis, are also possible intraoperatively on frozen sections to exclude or detect metastasis in the sentinel node(s) [60, 78, 79]. A simpler, though less sensitive, technique is imprint cytology [80]. If the sentinel lymph node does not harbor metastasis, no further axillary dissection is necessary. However, if the sentinel node has metastasis, it is possible to perform axillary lymphadenectomy immediately. On the other hand, if complete intraoperative histopathologic evaluation of the sentinel lymph node is not performed, it is necessary to wait for definitive histology usually obtained within a week. If metastases are detected, axillary lymphadenectomy may be performed with a second procedure.
Accuracy of SLNB and Long-Term Prognosis
The fundamental issue concerning radioguided SLNB as the “standard of care” in breast cancer is its reliability as a prognostic factor of the true axillary lymph node status. If SLNB is negative and axillary dissection is not performed, how reliable is the technique to assure with certainty that other axillary lymph nodes do not contain metastases? Different approaches have been employed to address this issue. Historically, the first approach was represented by initial studies in which patients underwent SLNB and completion axillary lymph node dissection in the same surgical session regardless of the result of sentinel node analysis. Sentinel lymph node(s) and the remaining axillary lymph nodes were then analyzed separately. The results of these studies consistently showed that false-negative rates (the detection of metastasis in axillary lymph nodes when the sentinel node was tumor free) only occurred in about 3–7% of cases [38]. False-negative SLNBs are very rare in patients with early breast cancer [81], probably because of the low rate of nodal involvement as well as the accuracy of the procedure.
Long-term follow-up of patients whose SLNB is negative and who do not undergo axillary lymph node dissection is even more encouraging. Based on the results mentioned above, one would expect that about 3–7% of patients would develop axillary lymph node metastasis during follow-up after a negative SLND. Instead, results of a recent meta-analysis based on 48 studies involving almost 15,000 patients with a median follow-up of 34 months show that only 0.3% of the patients develop axillary metastasis when axillary lymph node dissection had not been performed after SLNB was negative [82]. It is also interesting to note that, in addition to the routine use of H&E staining and immunohistochemistry analysis, intra/subdermal injection of the radiopharmaceutical turned out to be a significant factor predicting the reliability SLNB. These results support the use of SLNB routinely in the clinical management of patients with early breast cancer [82–88].
Contraindications to SLNB in Breast Cancer
Contraindications to SLNB in breast cancer have progressively decreased. Some of the contraindications that were considered valid until recently are:
1.
Palpable axillary lymph nodes suspicious for metastasis, or metastatic involvement otherwise identified by noninvasive techniques during preoperative staging (US, CT, MRI, or [18F]FDG-PET).
2.
Tumor greater than T2, as these patients have a very high probability of presenting with axillary lymph node metastasis at diagnosis.
3.
Multifocal or multicentric cancer (although in these cases periareolar administration of the radiocolloid can circumvent the problem of multiple tumor location) or patients at high risk of developing tumor recurrence within 10 years.
4.
Prior axillary surgery that may alter the pattern of lymphatic drainage.
5.
Some other contraindications to SLNB in patients with breast cancer are still debated, for instance patients previously treated with wide excisional biopsy or patients undergoing neoadjuvant chemotherapy for locally advanced cancer. In these patients, the potential benefit of SLNB should be considered on a case by case basis [15 56, 89–96].
Learning Phase
Since radioguided SLNB requires close integration between different specialists (nuclear physician, oncologic surgeon, medical oncologist, pathologist, medical physicist), an initial learning phase is recommended to harmonize and optimize interaction between these different components involved, in order to merge into real multidisciplinary “team work”.
The most important parameters to test such a multidisciplinary team are (a) percentage of SLNBs successfully identified and (b) percentage of false negatives (with a preliminary phase during which all patients still undergo axillary lymph node dissection in addition to SLNB). It is often considered that 20–40 procedures with completion axillary dissection are sufficient in order to implement radioguided SLNB into the routine clinical practice of a given hospital. These numbers, however, are highly variable, and SLNB should only be introduced to clinical practice where the team demonstrates high identification rate and accuracy [56, 81].
Radioprotection Aspects
Since radiopharmaceutical activity necessary to perform lymphoscintigraphy does not cause significant radiation burden to the patient, radioprotection issues are more related to the level of radiation exposure for staff in the operating room. The amount of 99mTc-colloid injected into the patient is very low (about 3–5% of the radioactivity administered in most diagnostic test such as bone scintigraphy). In two-day protocols, surgery is performed after about 2–3 half-lives of radioactivity, thus making the intraoperative dose even lower. Repeated measurements have clearly demonstrated that the cumulative radiation dose to personnel involved in radioguided SLNB (surgeon, nurse, pathologist) for 100 procedures corresponds to about 1% of the yearly limit allowed for the general population in most countries [97–101]. Furthermore, contamination with residual radioactivity of material from the operating room (surgical gauzes, liquids, and biological tissues of the patient) is minimal already at the time of surgery. Due to the fast physical decay of 99mTc, it is sufficient to wait only a few hours before disposal of operating room material to ensure an almost nonexistent radioactivity exposure to personnel.
Radioguided SLNB in Cutaneous Melanoma
Cutaneous melanoma can manifest differently from patient to patient. It is often highly aggressive and usually fatal if diagnosed in advanced stages. Better prognosis is observed in the early stages, when the probability of distant metastasis is low and resection of the primary tumor and regional lymph nodes may be curative. Five-year mortality is approximately 20% in stages I–II but higher in stage III (35%) and stage IV (90%) [102, 103].
In the very early stages of disease (with Breslow thickness of 0.76–1.5 mm), the incidence of metastatic involvement of the sentinel lymph node(s) is only about 5%, but it increases up to 20% in melanomas with thickness between 1.5 and 4 mm. With Breslow thickness >4 mm, the incidence of metastases in sentinel lymph node rises to about 50% [104]. The 5-year mortality is around 70–75% with nodal metastasis, while it is much lower (about 30%) for patients without regional metastasis [104]. Radioguided SLNB probably has maximum clinical impact in the early-to-intermediate stages of disease (Breslow thickness <4 mm) [105–108].
While in breast cancer axillary lymphadenectomy performed in case of positive sentinel node is mainly used to better stratify patients for prognostic purposes, in cutaneous melanoma locoregional lymphadenectomy represents immediately an effective therapy for patients with metastases in sentinel lymph nodes. In fact, lymphadenectomy reduces mortality from about 70% to about 50% in these patients. The status of the sentinel lymph node is included in the latest version of TNM staging for cutaneous melanoma reflecting its importance for staging and treatment [109, 110].
Similarly as for breast cancer patients, for patients with melanoma, the presence of a sentinel lymph node free from metastasis obviates the need for lymphadenectomy, while the presence of metastasis mandates it [111, 112].
The Cutaneous Lymphatic System
While the epidermis is devoid of any lymph vessels, the dermis is particularly rich with lymphatic circulation, and there are close connections between the lymphatics of the dermis and those of the subcutaneous layers, even of the deep perifascial tissues.
Lymph from extremity lesions flows from the site of the primary tumor, predictably to the ipsilateral axilla or to the ipsilateral inguinal lymphatic basins. On the contrary, patterns and direction of lymphatic drainage are very variable and often unpredictable for melanomas in the median and paramedian regions of the trunk, as well as in the head and neck region. In these locations, lymphatic drainage is often ambiguous with drainage possible to both axillary and inguinal regions or to the contralateral neck (Fig. 28.10) [113–117].
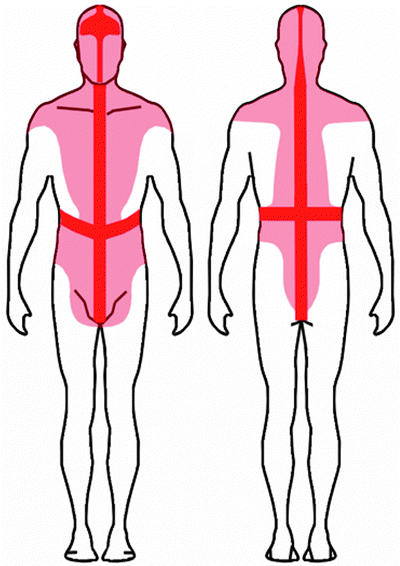
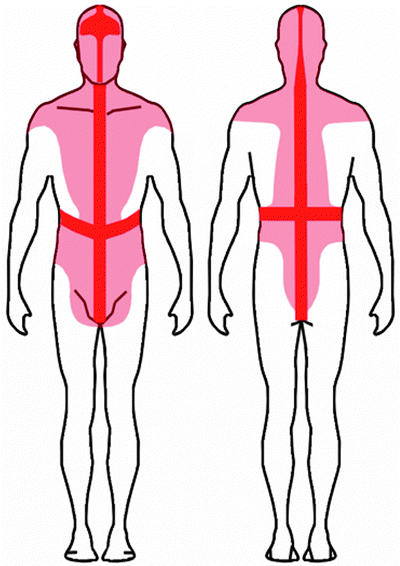
Fig. 28.10
Recent lymphoscintigraphic studies with 99mTc-radiocolloid in patients with cutaneous melanoma demonstrate that ambiguous lymphatic drainage (i.e., possibility of draining toward either left- or right-sided lymph nodes) from skin regions around the body midline can extend to a quite wide area that includes virtually the entire head, the neck, and the shoulders, as well as a relevant area around the waist (shown in pink color in the schematic representation of the body in the anterior and posterior views). This pattern is in contrast with classic anatomic notions (Sappey’s concept, shown in red color), according to which ambiguous lymphatic drainage corresponds to a restricted area extending only approximately 5 cm on each side of midline of the body. (From Mariani G, Gipponi M, Moresco L, Villa G, Bartolomei M, Mazzarol G, Bagnara MC, et al. Radioguided sentinel lymph node biopsy in malignant cutaneous melanoma. J Nucl Med. 2002;43:811–827, with permission)
Radiopharmaceuticals
The considerations already noted above concerning breast cancer also apply to melanoma.
Technique of Radiocolloid Administration
Because of the anatomical and physiological parameters previously mentioned with respect to the lymphatic system of the skin, there is general consensus that the optimal radiocolloid administration in patients with melanoma is intra/subdermal injection. This is usually performed as multiple administrations around the primary tumor or around the recent surgical scar, which is the most common occurrence since the diagnosis and thickness of melanomas is usually determined by excisional biopsy. In order not to alter the lymphatic drainage pattern or kinetics, as could be caused by an excessive increase in interstitial pressure, it is generally recommended to inject small volumes of radiocolloid, about 0.05–0.2 mL per aliquot, each containing about 5 MBq of 99mTc-radiocolloid. The total administered activity is generally higher, usually between 74 and 100 MBq, than that used for breast cancer [118, 119].
For melanomas of the extremities, a single injection could be performed perilesionally on the side toward the axilla or groin (Fig. 28.11). Often, the radiocolloid is injected laterally to the tumor or surgical scar as well. For lesions located on the median/paramedian regions of the trunk, it is best to make at least four injections around the primary lesion and even more for large surgical scars.
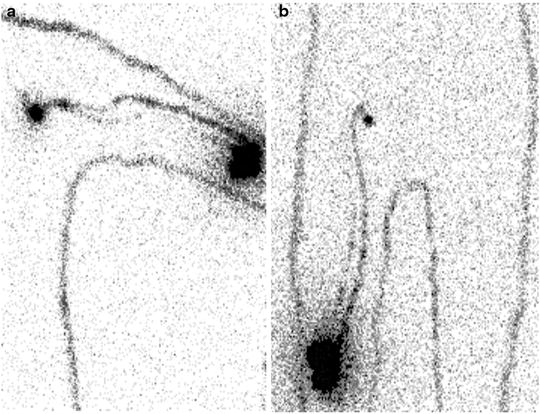
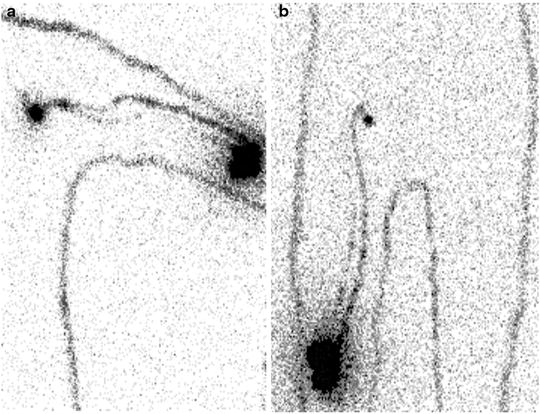
Fig. 28.11
Typical patterns of lymphatic drainage in patients with primary melanomas located in the extremities, as visualized between 30 and 60 min after intra/subdermal injection of 99mTc-nanocolloidal albumin. (a) Patient with recent surgical excision of cutaneous melanoma on left elbow: lymphoscintigraphy (anterior view) shows lymphatic drainage through at least two lymphatic vessels merging into a single sentinel lymph node in the left axilla. (b) Patient with recent surgical excision of cutaneous melanoma on right thigh (just above the knee): lymphoscintigraphy shows lymphatic drainage through at least two lymphatic vessels merging into a single sentinel lymph node in the right groin
Lymphoscintigraphy
Lymphoscintigraphy is generally performed using a wide field of view gamma camera, high-resolution collimator, and energy window centered on the 140 keV peak of 99mTc (±10%). Unlike lymphoscintigraphy for breast cancer, dynamic scintigraphic acquisition, preferably with a 128 × 128 matrix, should always be performed for melanoma [118, 120]. The dynamic images (1 every 30 or 60 s) are usually acquired during the first 20–30 min from injection, after which static images of the lymphatic basin reached are usually acquired. Planar static images (typically acquired for 300 s, with matrix 256 × 256) are acquired in different projections (anterior, posterior, lateral, various oblique views), depending on the pattern of lymphatic drainage and location of the sentinel lymph node(s). Lymphoscintigraphy frequently shows more than one sentinel lymph node (Fig. 28.12). The most radioactive node may not be the first one visualized along the pathway(s) of lymphatic drainage, that is, the sentinel node/s. Dynamic acquisition is especially useful to highlight lymphatic drainage and to visualize the correct progressive order of lymph nodes along the drainage pathway.
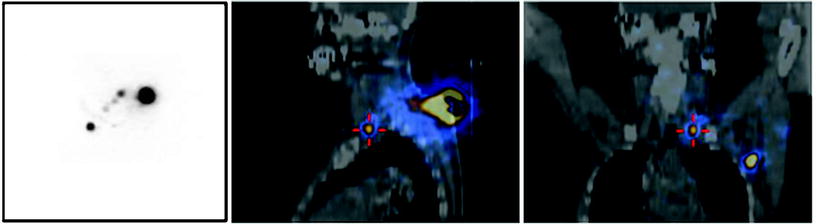
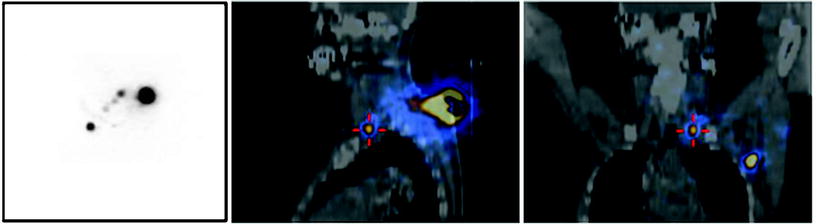
Fig. 28.12
Lymphoscintigraphic images obtained after intra/subdermal injection of 99mTc-nanocolloidal albumin around the surgical scar in a patient recently submitted to excision of a melanoma located in the back, along the left paramedial line in the subcervical region. Planar imaging (posterior view in the left panel) demonstrates lymphatic drainage to more than one node. Topographic location of the sentinel lymph nodes is more clearly visualized (in the left axilla and in the left supraclavicular region, respectively) by the fused SPECT/CT sections (sagittal and coronal in center and right panel, respectively)
Tomographic imaging with hybrid SPECT/CT equipment is especially useful when performing lymphoscintigraphy for patients with cutaneous melanoma, as it allows better evaluation of the anatomotopographic coordinates of the sentinel lymph node and the surrounding structures. When available, this method constitutes a valuable aid to the surgeon for more rapid localization of the sentinel lymph node [25, 28, 121]. It is useful by providing important additional information over planar imaging, especially when the sentinel node is located in unusual locations requiring unusual surgical access (see Figs. 28.2, 28.13, 28.14, and 28.15).
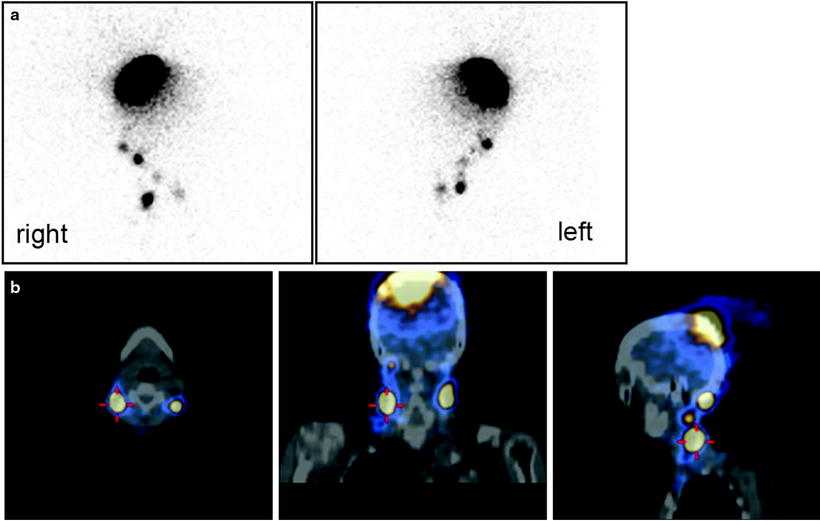
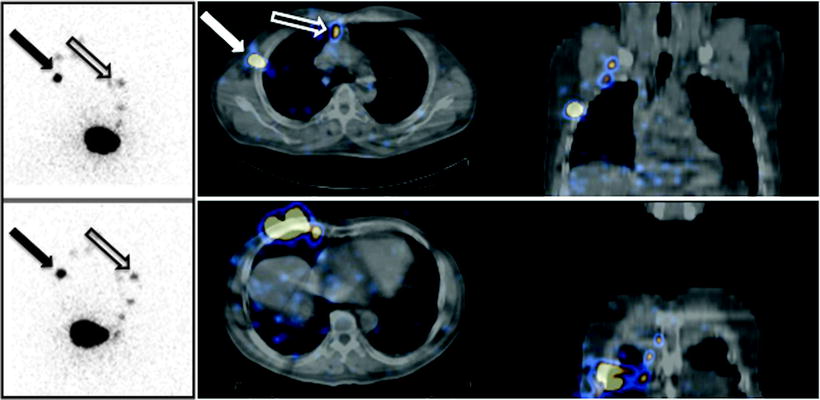
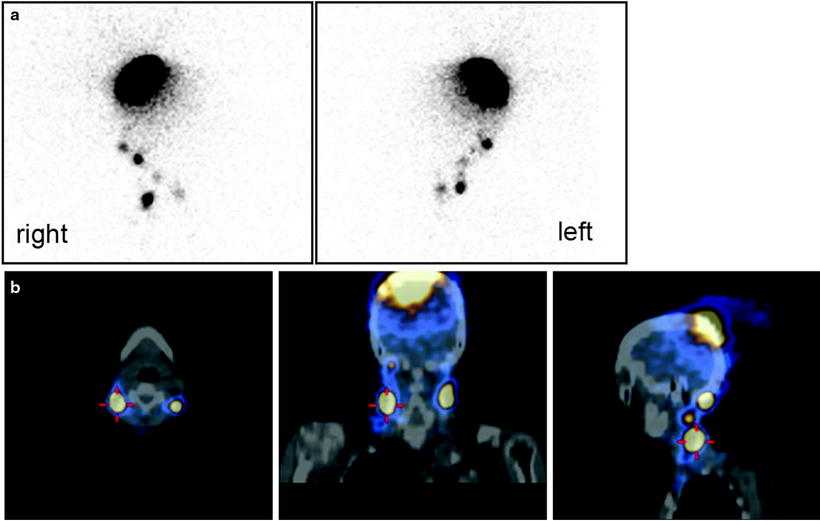
Fig. 28.13
Lymphoscintigraphy with 99mTc-nanocolloidal albumin obtained in a patient recently submitted to surgical excision of a cutaneous melanoma located about the vertex. (a) Planar images (right and left lateral views) showing lymphatic drainage to both right-side and left-side lymph nodes, the exact topographic location of which is difficult based on planar imaging alone. (b) Fused SPECT/CT transaxial, coronal, and sagittal sections (left, center, and right panels, respectively) showing the anatomic coordinates of various lymph nodes along the main lymphatic pathways on the right and left sides
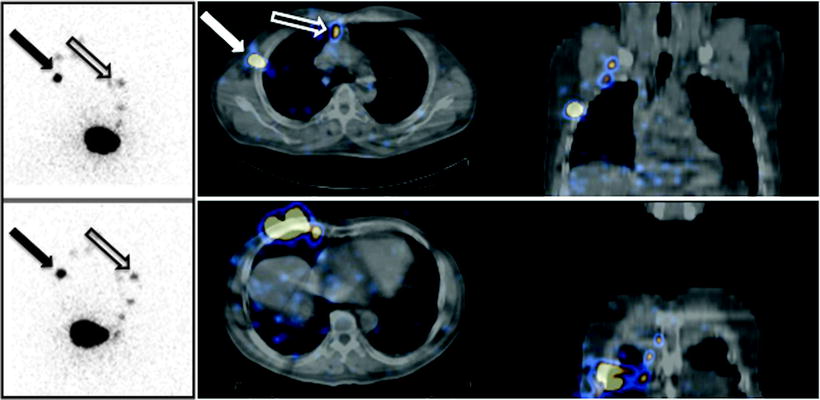
Fig. 28.14
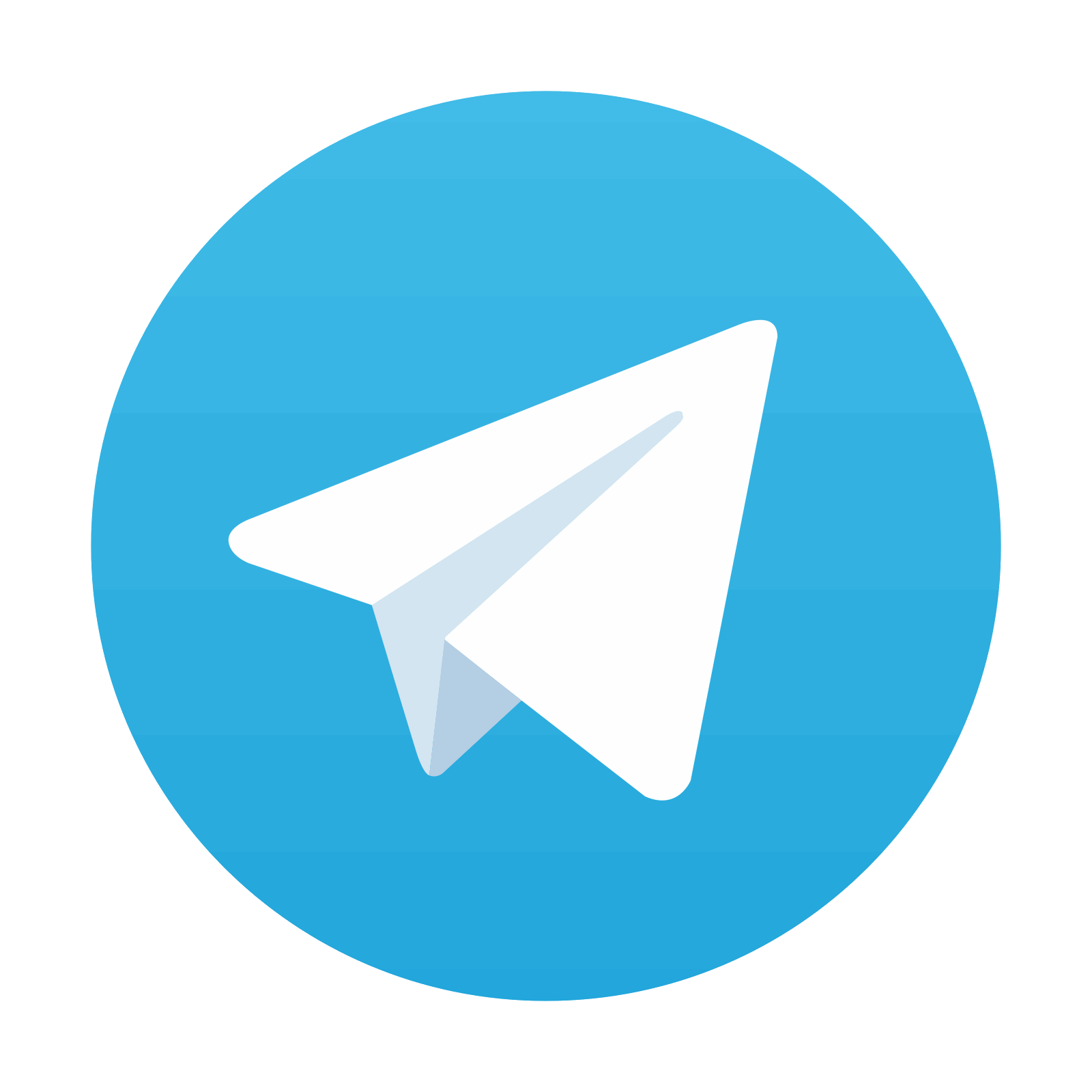
Planar images (on the left) and fused SPECT/CT sections (on the right) obtained after intra/subdermal injection of 99mTc-nanocolloidal albumin in a patient with cutaneous melanoma located in the right mammary region. Planar imaging (anterior view on the top, right anterior oblique view on the bottom) shows lymphatic drainage of the radiocolloid both to axilla (closed black arrow) and to the internal mammary chain (open black arrow). SPECT/CT images (transaxial and coronal sections at two different levels) demonstrate topographic localization of sentinel lymph nodes relative to surrounding anatomic structures in the axilla (closed white arrow) and in the parasternal space (open white arrow)
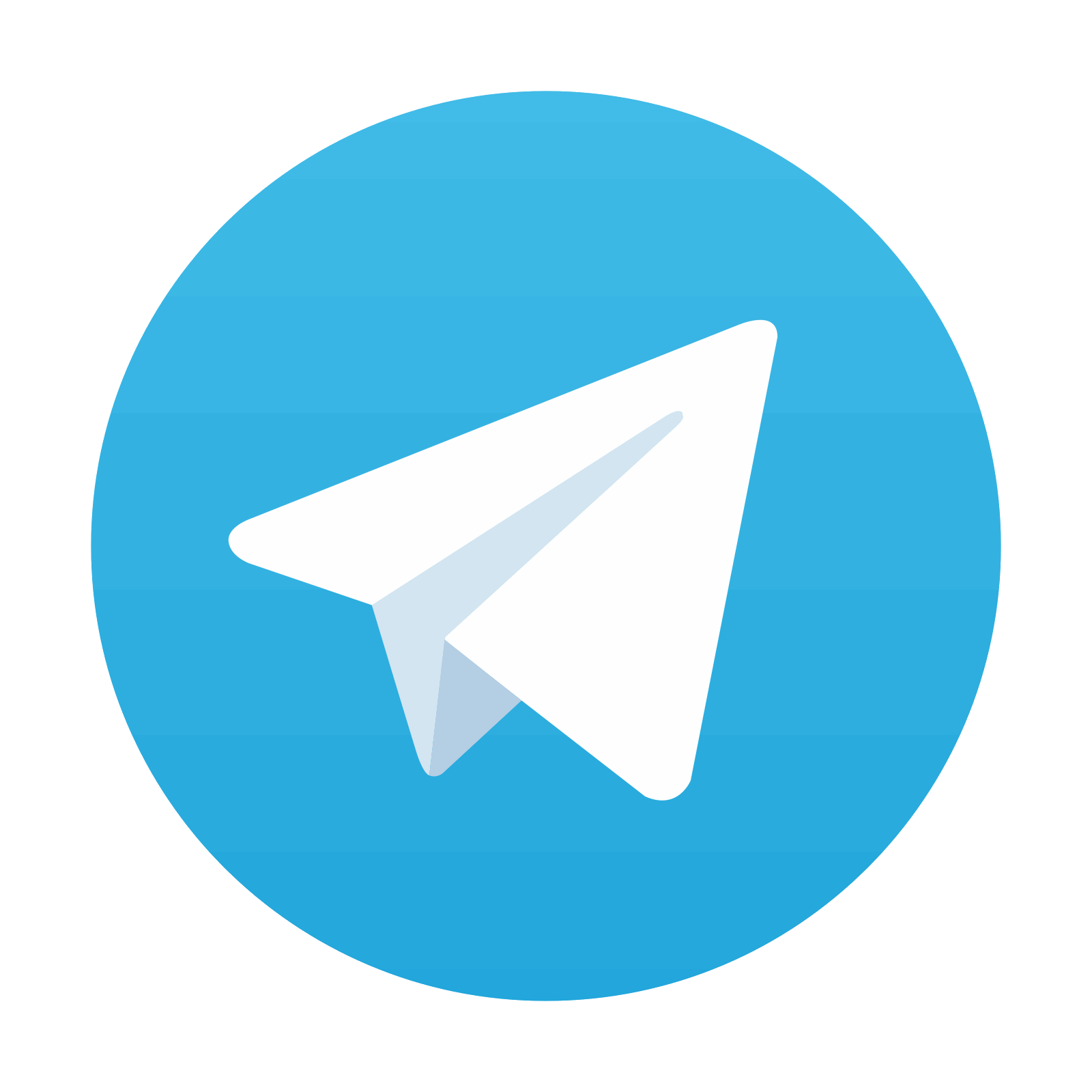
Stay updated, free articles. Join our Telegram channel

Full access? Get Clinical Tree
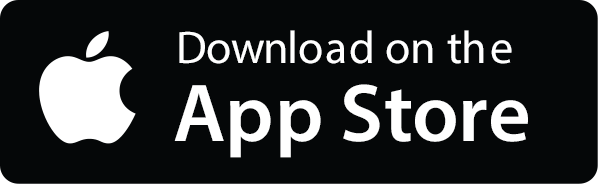
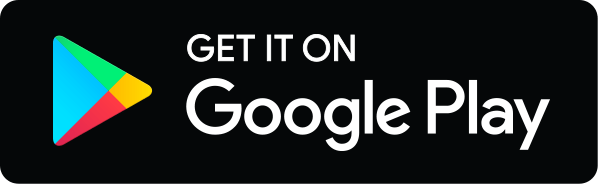
