Fig. 1
Arronax is a 70 meV cyclotron with multiparticle capacity. It can accelerate H− ions up to 70 MeV (tunable between 30 and 70 MeV) and extract them through two independent beam lines. Maximum intensity is 2 × 375 μA. Arronax can also accelerate and extract alpha particles (He2+) at a fixed energy of 68 MeV and a maximum intensity of 70 μAe, and H–H+ ions at 17 MeV and a maximum intensity of 50 μA. Particle beams may be directed to six different vaults for radionuclide production or physics and biology experiments. With the addition of energy degradors, Arronax will produce a variety of radionuclides, both for research and industrial applications. A priority list has been set, which include astatine-211 and copper-67 for therapy, as well as copper-64 for imaging and dosimetry. Scandium-44 and scandium-47 are just after in this priority list
Copper-67 can be produced by irradiating enriched 68Zn targets with high energy protons (Mirzadeh et al. 1986). Due to the small cross section (10 mb) of the nuclear reaction, high intensity is also required. The Arronax cyclotron, in Nantes, France, with its high energy (70 MeV) and intensity (> 2 × 200 μA) will allow us to produce the high activities (up to 1.2 Ci EOB/week) required for clinical trials (Fig. 1)
Copper chelation chemistry is very rich (Wadas et al. 2007). A lot of bifunctional chelating agents have been synthesized and conjugated to carrier molecules including peptides and antibodies (Novak-Hofer and Schubiger 2002). The challenge has been the kinetic lability of many copper chelates. The biodistribution and metabolism of an anti-colorectal antibody, as a whole IgG or as the F(ab′)2 fragment, labeled with 67Cu using four different bifunctional chelating agents, have been studied (Rogers et al. 1996). The results showed different levels of kidney and liver uptake according to the studied species (rat or hamster), the form of the immunoconjugate (IgG or fragment) and the nature of the chelator. For the F(ab′)2 fragment, chelate charge and lipophilicity played a large role in kidney retention while, using the whole antibody, transchelation of copper to copper-binding proteins (metallothionein, superoxide dismutase and ceruloplasmin) was a major factor for high liver uptake.
More recently several new ligands have been synthesized and their complexation to Cu2+ ions has been tested. Cross-bridged cyclams and bispidine ligands are now proposed for peptide and antibody labeling (Boswell et al. 2008; Gasser et al. 2008; Juran et al. 2009). For example, hexadentate bispidine ligands have been shown to form stable radiocopper complexes under mild conditions, in almost quantitative yield and with no evidence of transchelation or demetalation (Juran et al. 2009). Indeed there is no doubt that, in the near future, there will be some favorable bifunctional chelators for evaluation in clinical studies. These clinical studies will aim at selecting the most favorable radionuclide for radionuclide therapy among iodine-131, lutetium-177 and copper-67. In the past, a clinical RIT study using an anti-CEA antibody labeled with copper-67 and iodine-125 in patients with colorectal tumors showed that copper-67 was more favorable than iodine-125 due to higher tumor-to-blood ratios despite a problem of nonspecific liver and bowel uptake (Bischo-Delaloye et al. 1997). A copper-67-labeled antibody has also been tested in the treatment of lymphoma (DeNardo et al. 1999). More recently a preclinical RIT using an antibody labeled with copper-67 and lutetium-177 in nude mice bearing a neuroblastoma xenograft concluded that, with the exception of tumor/blood ratios, the tumor/tissue levels of the copper-67 immunoconjugate seemed more favorable than those of the lutetium-177 compound (Grünberg et al. 2005). Today the major impediment to the development of copper-67-labeled radiopharmaceuticals remains copper-67 poor availability.
2.2 Scandium-47
Scandium-47 also has excellent physical properties (Mausner et al. 1998). A relatively long half-life of 3.35 days, low energy beta emission (Emax: 440 keV, Eav: 162 keV) and a gamma emission at 159 keV, which should give excellent imaging. Scandium-44, a positron emitter, could be used for pre-therapeutic imaging and dosimetry calculation. DTPA-substituted antibodies have been labeled with scandium-46 and scandium-47 showing limited labeling stability with significant transfer of scandium to transferrin followed by liver uptake (Anderson and Strand 1985). Scandium is also known to bind to bones and has been considered for bone pain palliation as a zoledronate complex (Majkowska et al. 2009). For targeted radionuclide therapy, better chelation stability is required. Recent studies shows that DOTA is a good chelating agent for scandium, presumably providing enough labeling stability for targeted radionuclide therapy. The reason why scandium-47 has not yet attracted much interest for therapeutic applications is its poor availability. Scandium-47 can be prepared no-carrier-added (0.9 GBq μg−1) by irradiation of titanium targets and ion exchange purification, but the 48Ti(p, 2p) reaction has a relatively low cross-section and require high proton energy (around 25 MeV) (Kolsky et al. 1998).
3 Alpha-Particle-Emitting Radionuclides
Beta particles do not efficiently target isolated cells, because of the simple ballistic effect of their range, which is large as compared to the size of a tumor cell. Thus it is logical to select radionuclides that emit higher LET particles to deal with this situation. Then alpha-emitting radionuclides seem to be optimal, with a path length of the emitted particles of less than 100 μm. At the same time, the energy deposited along the short particle track is quite high: from 5 to 8 MeV. It is proposed that alpha particles create highly localized multiple DNA double strand breaks impossible to repair and that results in relative biological efficacy superior to 1, from 3 to 7 depending on which end-point (cell survival, double strand breaks) and experimental conditions are used (Sgouros 2008).
Several such radionuclides may be extracted from nuclear wastes directly or provided in the form of generators prepared from material extracted from nuclear wastes. However, one very attractive candidate for targeted alpha-radionuclide therapy is astatine-211, which is cyclotron produced. Actinium-225 and its daughter bismuth-213 may be produced from thorium-229, but also by cyclotron irradiation of radium-226 and thus will be shortly discussed here. Using alpha-emitting radionuclide for therapy remains a challenge. However, good news about Alpharadin (radium-223 injected systemically for pain palliation and bone metastasis treatment) development and progress in preclinical and early clinical trials raise increasing interest for this approach (Nilsson et al. 2005).
3.1 Astatine-211
Astatine-211 is prepared by cyclotron irradiation of a bismuth-209 target with alpha particles. The energy of the alpha particles must be fine tuned (maximum around 28 MeV on target) to prevent production of astatine-210, which decays in the long half-life alpha emitter polonium-210. A 1:10,000 ratio of astatine-210/astatine-211 is proposed as safe for clinical applications. The number of cyclotrons producing astatine is limited and many can only produce relatively small activities. Thus in spite of promising preclinical studies, only a few trials have been made in human so far.
Astatine may be considered as the heaviest halogen and as such, its chemistry resembles that of iodine, unfortunately with some differences. The absence of any stable or long half-life astatine isotope does not make study of its chemistry very easy. Some irreproducibility and poorly understood behavior of astatine in the extraction and labeling procedures, especially at high activities, that can produce significant radiolysis of solvents and reagents, still stand in the way of routine use of this radionuclide (Pozzi and Zalutsky 2007). It has been shown that direct labeling of tyrosine residues in proteins with astatine is not practical, unlike labeling with iodine, because of poor yield and stability. Alternative routes have been proposed, based on halogen exchange, with the disadvantage of yielding relatively low specific activity products, or based on activated stannylated synthons that are substituted with astatine through an electrophilic substitution. Once labeled, these synthons are coupled to proteins such as antibodies. Two of them have been frequently used. One is succinimidyl-m-astato-benzoate (SAB), obtained by substitution of tri-alkyl-tin of succinimidyl-m-trialkyl-tin-benzoate (Zalutsky and Narula 1988). The other is succinimidyl N-2-(4-astatophenethyl) succinamate (SAPS), also obtained from a trialkyl-tin precursor (Yordanof et al. 2001). This two step labeling procedure may be performed with a relatively good yield, but a one step method could improve the overall labeling yield (Lindegren et al. 2008). Stability of such astatine-labeled products has been questioned though, calling for further development of astatine labeling techniques. Stability of astatine tumor uptake in the case of ligand internalization remains problematic, here again calling for the development of better labeling approaches (Vaidyanathan et al. 2003).
In spite of these difficulties, astatine has attracted much interest because of a longer half-life (7.2 h) than bismuth-213 or bismuth-212. Astatine-211 does not directly decays into a stable daughter. In 58 % of the case, it decays into polonium-211, which has a 0.5 s half life. It has been calculated that polonium-211, in spite of its short half-life, may diffuse away from target cells, thus reducing the delivered radiation dose in the case of isolated target cells. In 42 % of the cases, astatine-211 emits an alpha particles and decays into bismuth-207, which has a 33.2 year half-life and is not thought to contribute significantly to the radiation dose delivered to tissues.
Several preclinical studies have shown promising results. First of all, in vivo astatide behaves similarly to iodide, with efficient uptake in NIS-positive cells. It has been proposed that astatide could be used, like radioactive iodide in thyroid cancer, but probably with little therapeutic advantage. However, uptake in NIS-expressing cells explains the biodistribution of free astatide, or of astatide released from catabolized or unstable astatinated radiopharmaceuticals, in the thyroid and in stomach (Lundh 2006). This property has also been exploited in several publications showing efficient killing of cancer cells transfected with the NIS gene, a gene-therapy also proposed for iodine-131 (Petrich et al. 2002). It is expected that radioactivity efflux from the tumor cells will be less problematic with the shorter half-life of astatine-211, as compared to iodine-131.
Small molecules have also been labeled with astatine-211. Iodo-phenylalanine may be labeled with astatine-211 by copper-catalyzed halogen exchange to good specific activities and used to target brain tumors in vitro (Meyer et al. 2010.). Meta-astato-benzyl guanidine (MABG), the astatine analog of MIBG has also been produced and tested. In bladder cancer cells transfected with the adrenaline transporter gene, MABG proved several orders of magnitude more toxic than MIBG (Fullerton et al. 2005).
Monoclonal antibodies, their F(ab′)2 fragments or diabodies, have also been labeled with astatine-211. The astatine-labeled anti-Tac antibody has been tested in NOD/SCID mice inoculated with human T cell leukemia showing significant survival improvement (Zhang et al. 2006). Antibody MX35, which recognizes human ovarian cancer cells, has been used effectively by intraperitoneal injection of its astatine-labeled F(ab′)2 fragment in the treatment of mice inoculated intraperitoneally with human ovarian cancer. Recently, it was shown that repeated treatment with this astatine-labeled antibody fragment made tumor-free a significant fraction of the animals (Elgqvist et al. 2010). The same antibody has been tested in a phase I study, in nine ovarian cancer patients, also by intraperitoneal administration at a concentration of 22.4–101 MBq/L, demonstrating the possibility of delivering therapeutic radiation doses in microscopic tumor clusters without significant toxicity (Andersson et al. 2009).
Glioma is another tumor with very poor prognosis. After surgical removal of the primary tumor, nearly all patients relapse with recurrent disease developing in the tumor resection margins. Thus an astatine-211-labeled anti-tenascin chimeric antibody (81C6) was prepared and administered intra-lesionally in a phase 1 study in 18 patients with recurrent malignant brain tumors (Zalutsky et al. 2008). The patients received single doses of 10 mg of 211At-labeled chimeric 81C6 mAb labeled with escalating doses of 211At (74–370 MBq). It was demonstrated that 97 % of the astatine decays occurred within the tumor cavity. Median survival for the 14 glioblastoma patients and all patients was 52 and 56.5 weeks, respectively, which is considerably better than that obtained with conventional treatments (25–30 weeks).
3.2 Actinium-225 and Bismuth-213
Actinium-225 has generally been obtained from the decay of thorium-229 isolated from nuclear waste, however, due to availability problems, the production of actinium-225 by cyclotron irradiation of radium-226 is now considered. Radium-226 represents almost 100 % of radium found as a decay product in uranium ores. Its long half-life (1602 years) has allowed the preparation of radium-226 cyclotron targets for the production of actinium-225 by bombardment with protons having an energy of 10–20 MeV. This production has been developed at ITU in Karlsruhe (Apostolidis et al. 2005) and is now used at the Technical University of Munich. Technological aspects or this production are covered by a series of patents.
Actinium-225 and bismuth-213 have been proposed years ago for medical applications in targeted radionuclide therapy (Geerlings et al. 1993). Bismuth-213 may indeed be a more favorable isotope than bismuth-212, proposed several years earlier (Kozack et al. 1986) because bismuth-212 emits a proportion of higher energy gammas (727, 785 and 1620 keV for bismuth-212 versus 440 keV for bismuth-213).
3.2.1 Bismuth-213
Bismuth-213 has been used in several preclinical and a few clinical trials (Jurcic et al. 2002) as a source of alpha particle for cancer ablation. Actinium-225 generators may be eluted every other hour providing a convenient source of bismuth-213. However, the short half-life (46 min) requires fast targeting. Several possibilities are being explored. Target cells may be readily accessible from (or in) the circulation. This corresponds to many cases of hematological tumors (e.g. treatment of AML with bismuth-213-labeled antibody: Jurcic et al. 2002), cells present in bone marrow (multiple myeloma: Supiot et al. 2002), cells constituting the blood vessels (angiogenic endothelial cells: Davis et al. 1999) and possibly residual disease in the adjuvant setting for many cancers. Technically, targeting can be made faster by the use of small molecular weight carrier of the radionuclide, such as peptides (Wild et al. 2011), small antibody fragments or pretargeted small molecular weight carrier molecules (biotin, haptens) (Zhang et al. 2002). Finally, efficient targeting may be achieved by loco-regional administration as demonstrated in several very elegant animal models (gastric cancer: Beck et al. 2007, bladder cancer: Pfost et al. 2009). However, tumor burden must also be quite small, as treatment efficacy is dramatically reduced when the delay between tumor inoculation and treatment is increased. This effect may be explained by the formation of larger, vascularized, tumor nodules that are not accessible to the radiolabeled product injected in the cavity, but, with short half-life and highly toxic bismuth-213, the number of injected radioactive atoms may no longer be large as compared to the number of target cells, even if a small number of alpha-particle tracks hitting the cell nucleus has been demonstrated to be enough to kill a cell. This reason has been advocated to explain the low efficacy of the treatment of AML in high tumor burden patients. In this study of patients with acute myelogenous leukemia (AML) and chronic myelogenous leukemia (CML) a humanized monoclonal anti-CD33-antibody, HuM195, was labeled with bismuth-213 (Jurcic et al. 2002). This study was the first proof-of-concept where a human being was treated with a systemic radioimmunotherapy comprising an α- emitter. Eighteen patients were treated with 10.4–37.0 MBq/kg bismuth-213-labeled antibody. All 17 evaluable patients developed myelosuppression, but recovered. The antibody rapidly localized to areas of leukemic involvement. Fourteen (93 %) of 15 evaluable patients had reductions in circulating blasts, and 14 (78 %) of 18 patients had reductions in the percentage of bone marrow blasts. Tumor burden as large as 1012 cells (equivalent to a 1 kg tumor) was observed in this trial. Since the maximum injected activity was about 3 GBq, which corresponds to about 12×1012 bismuth-213 atoms, only 12 atoms per tumor cell were injected. Tumor debulking prior to alpha-radiotherapy should thus be the rule.
3.2.2 Actinium-225
Actinium-225 has been presented as an “atomic nanogenerator” of a cascade of radioactive daughters (francium-221, astatine-217, bismuth-213/polonium-213) with a total emission of four alpha particles per actinium-225 atom (McDevitt et al. 2001). The long half-life of actinium-225 being compatible with highly effective targeting agents such as intact IgG antibodies, actinium-225 coupled to internalizing monoclonal antibodies injected as single doses at kBq levels into mice bearing prostate carcinoma or human lymphoma induced tumor regression and prolonged survival in a substantial fraction of animals. If all consecutive disintegrations occur in the same cell, one actinium-225 atom could deliver more than 25 MeV. However, after disintegration, francium-221 is released from the actinium-225 targeting agent and, with a 4.8 min half-life, it may diffuse away. The problem is even worse with bismuth-213 and its 46 min half-life (Jaggi et al. 2005). Targeting internalizing antigens or receptors is claimed to maintain the daughters of actinium-225 secluded within the target cell. However, many disintegrations of actinium-225 occur outside the target cells, as targeting is never 100 % efficient and relatively long-lived bismuth-213 is released that reach the kidneys and bind to it. Solutions to reduce kidney uptake have been proposed but certainly there is a difficulty here. Locoregional treatment, such as intrathecal administration for the treatment of neuroblastoma meningeal carcinomatosis, should not face the same problem (Miederer et al. 2004).
3.3 Terbium-149
Recently terbium-149 has been presented as an alpha particle-emitting radionuclide candidate for alpha-immunotherapy. This radiolanthanide has a half-life of 4.118 h and emits alpha particles with a relatively short range of 28 μm in tissue. It has been produced at ISOLDE-CERN by on-line mass separation of spallation products released from a tantalum target irradiated with 1 GeV protons. As a radiolanthanide, terbium-149 can be easily complexed by the commonly used CHX-A-DTPA chelating agent.
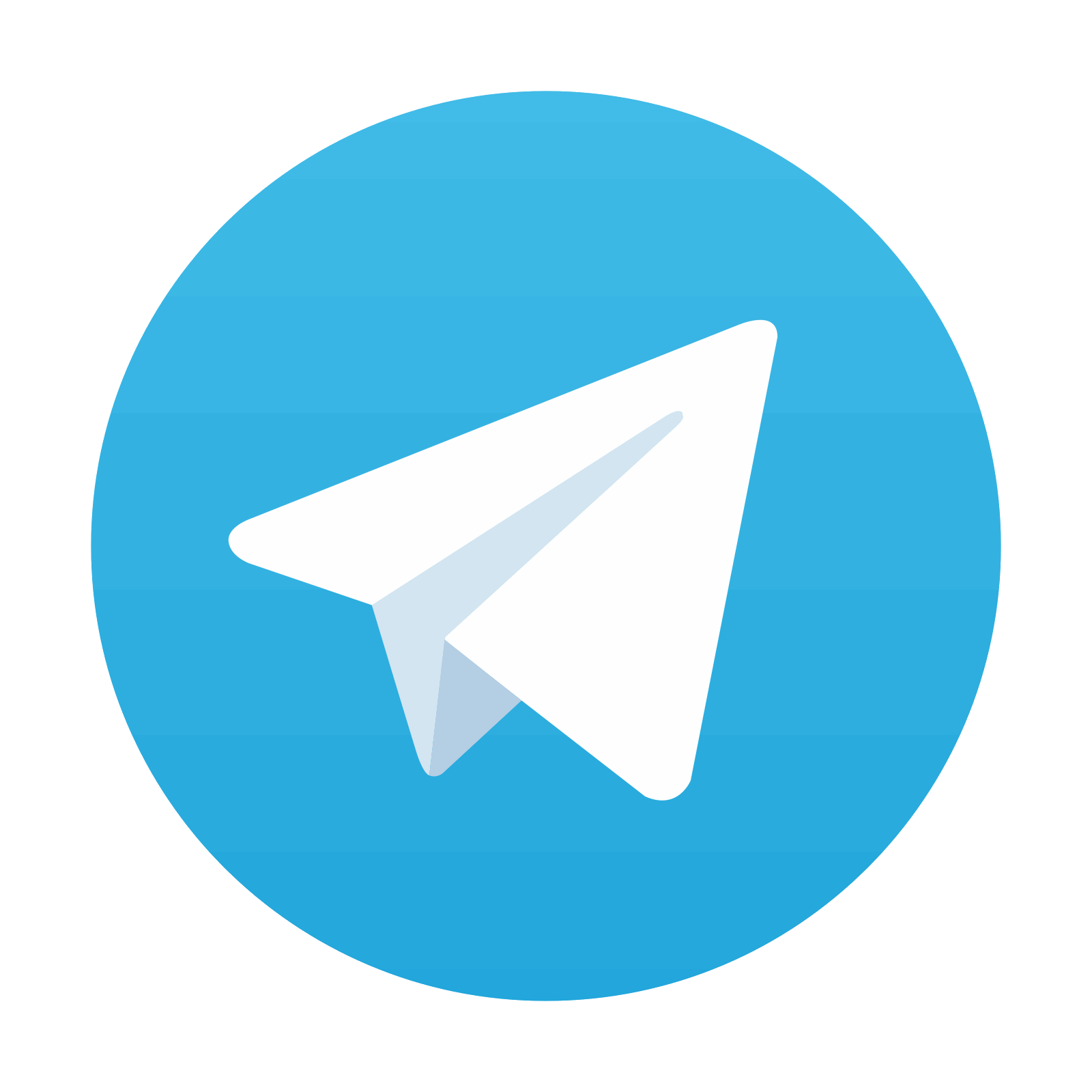
Stay updated, free articles. Join our Telegram channel

Full access? Get Clinical Tree
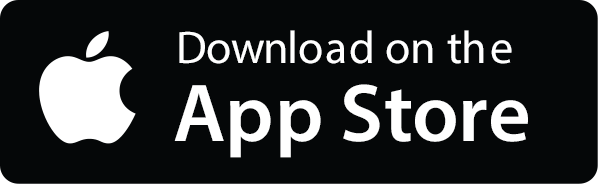
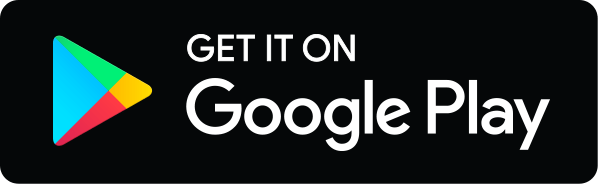