where Co is the initial concentration (mCi/g) of I-131 in the lesion, and T1/2 is the effective half-life of activity in the lesion in hours. Thus, in order to determine the dose in a given case, we need to know how much activity (in absolute units) is contained in a lesion. Lesion mass is another parameter that must be determined for calculation, and can be determined satisfactorily using, for example, high-resolution computed tomography (CT) or ultrasound (US) images.
Reported dose–response relationships differ. Maxon (1999) reported a favorable response in lymph node metastases for a tumor dose of 80 Gy, whereas Flower et al. (1989) reported an inadequate response at 120 Gy. Dorn et al. (2003) observed complete responses for doses ranging from 100 to 150 Gy, although they also encountered a skeletal metastatic focus that did not respond to 480 Gy. These variable responses to treatment are due, in part, to the heterogeneity of radioiodine distribution within metastatic lesions.
A recent report found that I-124 positron emission tomography (PET) provides quantitative dosimetry information on remnant thyroid and metastatic lesions (Eschmann et al. 2002). In this previous study, I-124 75–150 MBq (2–4 mCi) was used to determine radiation and absorptive doses of I-131 administered to individual lesions.
1.3 Maximal Safe Dose Method
The third method, which was first introduced by Benua et al. (1962) in 1962, involves the administration of a maximal safe dose (MSD). The dose-limiting toxicity of I-131 treatment is mainly determined by hematopoietic bone marrow, and the limiting dose (administered activity) was defined in this study to be the dose that delivers 2 Gy (200 rad) to blood (surrogate of bone marrow) or a whole-body retention of <4.44 GBq (<120 mCi) at 48 h post-administration.
The MSD method could be improved for the treatment of residual lesions by combining it with quantitative dosimetry. Dorn et al. (2003) found that doses to metastases were more than 100 Gy for successful treatment. It is clear that optimal doses that deliver sufficient radiation to tumors allow better individual optimization and help prevent unnecessary radiation exposure.
Recombinant human thyroid-stimulating hormone (rhTSH) provides an alternative means of elevating the thyroid-stimulating hormone level without inducing hypothyroidism (Luster et al. 2000), and bone marrow absorbed doses of I-131 are expected to be lower in patients given rhTSH than in patients subjected to L-T4 withdrawal (de Keizer et al. 2004). The main reason for this is that renal clearance is lower for hypothyroid patients than euthyroid patients (Montenegro et al. 1996). Furthermore, Menzel et al. (2003) demonstrated that rhTSH reduces the whole-body effective half-life of I-131.
1.4 The Advantages and Disadvantages of MSD
1.4.1 Comparison with the Fixed Dose Method
The heterogeneity of thyroid cancer patients suggests that empirical dosing may not be associated with optimal results in all patients. Ideal dosing of radioiodine in metastatic thyroid carcinoma requires the delivery of a lethal dose of radiation to metastases while minimizing side effects.
MSD has a theoretical advantage over fixed dose therapy. In general, more radiation increases the likelihood of ablating residual disease and of complete remission (Maxon et al. 1983), although the amounts of I-131 delivered to tumors and the radiosensitivities of individual tumors are highly variable. However, repetitions of low fixed doses of I-131 induce thyroid cancer cell dedifferentiation and reduce the radioactive iodine uptakes of cancer cells (Benua et al. 1962), which are largely due to the slow growth and heterogeneity of thyroid cancer. Thus, initial radioactive iodine therapy is an important consideration. The main strength of the MSD approach is that it allows a higher radiation single dose to be delivered to metastases. Furthermore, it has been reported that as many as 1 in 5 patients have a maximal allowable safe dose of less than the empirical fixed dose (Beierwaltes et al. 1982).
Because of total cumulative dose limitations, high MSD therapy should be considered promptly when a patient is considered to be resistant to conventional fixed dose therapy. MSD can ablate thyroid tumors before the emergence of I-131 resistant tumor cells and also kill resistant tumor cells due to the so-called crossfire effect, which essentially concerns cell damage due to I-131 uptake in adjacent cells.
The MSD approach has several limitations. First, it is more expensive and more inconvenient than the fixed dose method, and second it does not estimate the radiation dose to metastases, and thus the maximal allowable safe dose might not have any therapeutic effect (Van Nostrand et al. 2002).
1.4.2 Comparisons with the Lesion-Based Dosimetric Method
The technical limitations of the lesion-based dosimetric approach are noted in Table 1. Other limitations include; (Seidlin et al. 1946) its higher cost and inconvenience and (Benua et al. 1962) the potentially difficultly of implementing this approach in distant metastatic lesions (Van Nostrand et al. 2002).
A single-exponential model may not accurately reflect the kinetics of radioiodine in lesions |
It assumes instantaneous uptake and a maximum dose at time zero |
Lesion mass estimations are problematic |
It assumes a uniform distribution of I-131 in lesions |
Measurements are prone to errors |
Therapeutic response relative to Dose Rate is different |
Dose reductions are required for lesions of <5 mm in diameter |
2 Calculation of Maximal Safe Dose
In the maximum safe disk (MSD) method, radiation dose to blood is used as a surrogate of the red bone marrow radiation safety level (Maxon et al. 1983). According to the Memorial Sloan-Kettering Cancer Center, I-131 dose should not; (1) exceed 2 Gy to blood, (2) result in a body retention of >120 mCi at 48 h, or (3) result in lung retention of >80 mCi at 48 h in cases of diffuse pulmonary metastasis (Leeper 1985). In their 150 patients over 25 years of follow up, no fatalities related to bone marrow radiation or pulmonary fibrosis have occurred. In initial study, maximum blood radiation exposure was set at 3 Gy. However, at this level, serious adverse effects, such as, bone marrow depression and radiation pneumonitis, developed and four patients died. Accordingly, the blood radiation exposure limit was decreased to a more conservative 2 Gy (Robeson et al. 2002).
After the MSD calculation was initially introduced (Benua et al. 1962; Leeper 1985; Benua and Leeper 1986), several modifications have been implemented, which include; dropping the requirement for urine collection, reducing blood collection, and altering the kinetic modeling procedure (Furhang et al. 1999; Hermanska et al. 2001). Briefly, the Memorial Sloan-Kettering Cancer Center method involves the following. Blood samples (2 mL) are collected at 2, 24, 48, and 72 h after the oral administration of I-131 [0.2 GBq (5 mCi) tracer dose]. Blood samples and a standard source (10 µCi of I-131) are counted using a gamma counter and decays are corrected. Radioactivities of blood samples are converted to μCi/L using standard data. Cumulative radioactivities are calculated over four time periods, namely, 2–24, 24–48, 48–72, and 72 h to infinity. It is assumed that it takes 2 h for orally administered I-131 distribute evenly in 5 L of whole blood. Using λ for biologic clearance from the count at each time, cumulative radioactivities (µCi h/l) are calculated for whole time span. Absorptive doses are converted to Gy (by assuming that average beta energy is 0.2 MeV and that the specific gravity of blood is l kg/L) and represent whole-blood absorption doses per unit tracer dose of I-131. MSDs are then determined by assuming that the absorptive dose to blood should not exceed 2 Gy.
The determination of MSD according to Memorial Sloan-Kettering Cancer Center method is based on many assumptions. Furhang et al. (1999) compared the results of the standard fitting method and the individualized clearance parameter fitting method. Results were similar in 11 of 14 patients (differences were within 10 %). However, the assumption that radioactive iodine distributes instantaneously in 5 L of blood introduced an error of up to 30 %, and when physical decay after last data point does not approach to zero, cumulative activity was up to 50 % smaller which results in up to 50 % larger MSD.
Benua and Leeper (1986) administered an average single therapeutic dose of 11.4 GBq [308 mCi I-131 (range 70–654 mCi)]. Total radioiodine doses exceeded 37 GBq (1 Ci) in six patients, and the largest cumulative dose was 77.7 GBq (2.1 Ci). However, 19 % of Leeper’s patients were treated with doses below 7.4 GBq (200 mCi). In our experience, the average dose delivered during a single MSD session is 12.5 GBq (337.4 mCi), which is close to that reported by the Memorial Sloan-Kettering Cancer Center (Lee et al. 2008). In our study, calculated MSDs were not less than fixed doses in any patient. However, van Nostrand et al. (Beierwaltes et al. 1982) reported that MSD resulted in less than the empirical fixed dose in 20 % of patients. These results suggest that more radiation can be safely administered by MSD than by fixed dose therapy.
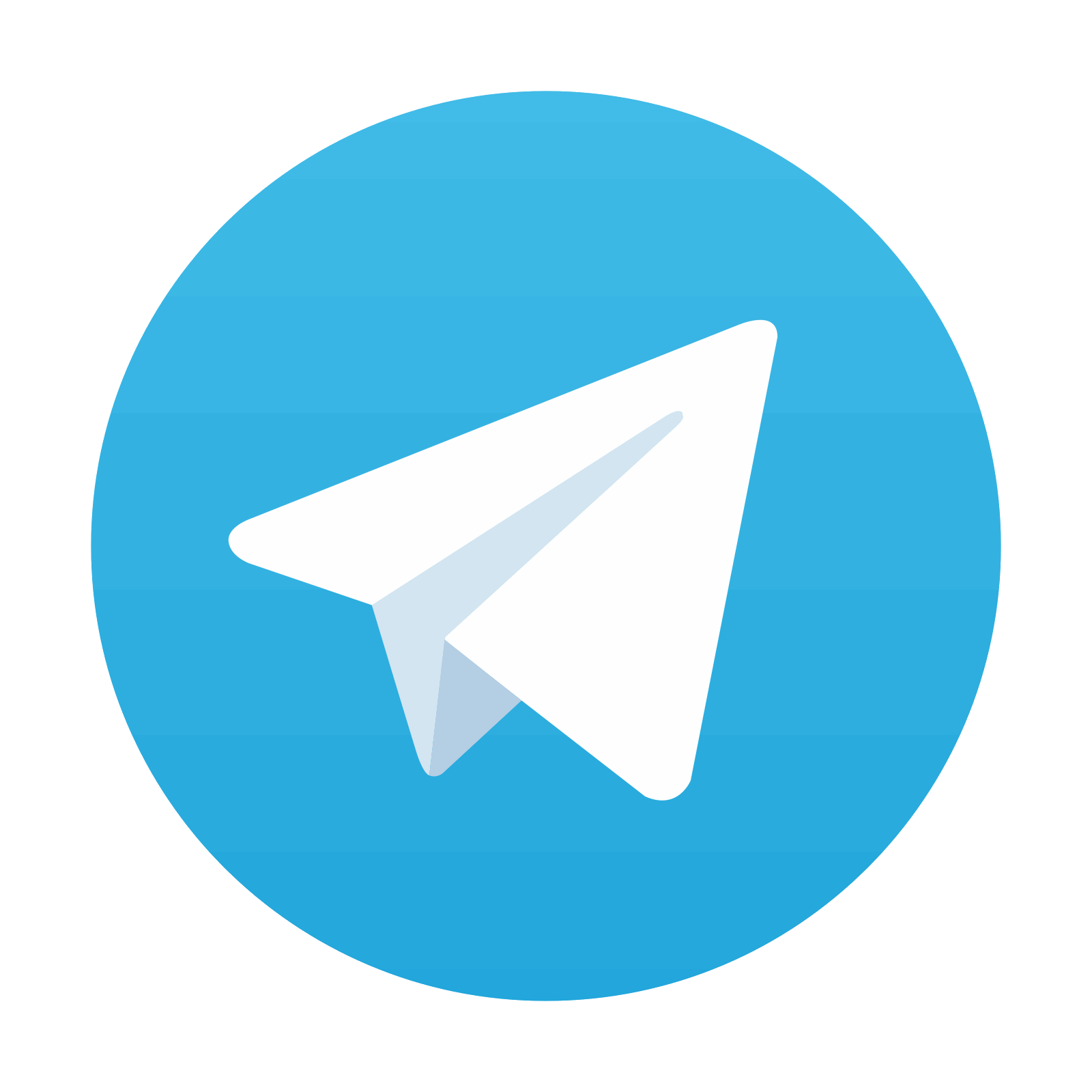
Stay updated, free articles. Join our Telegram channel

Full access? Get Clinical Tree
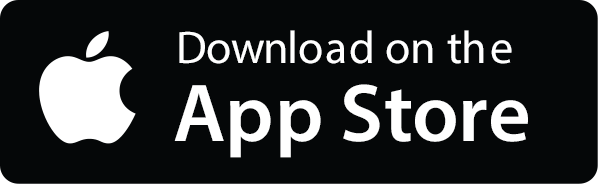
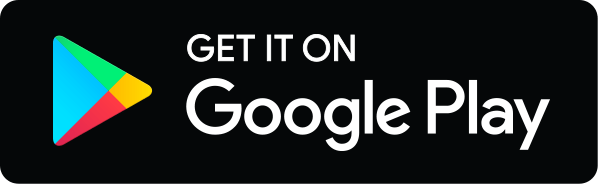