Imaging studies play a central role in the diagnosis of spinal trauma. The biomechanics, natural history, and treatment methods differ significantly in cervical and thoracolumbar injuries and are therefore addressed separately in this chapter. Multiple classification systems have been developed and continue to evolve. We will focus on the analyses of injury characteristics and will discuss the classification systems that have been evaluated for validity and reliability.
Vascular injuries of the cervical vessels and aorta, although common in the setting of spinal trauma, are beyond the scope of this chapter. The appropriateness criteria for imaging studies will be reviewed because they are essential for the radiology practice.
Cervical Spine Trauma
Indications
The imaging modalities that are available for the evaluation of spinal trauma include plain radiographs of the spine, computed tomography (CT) of the cervical spine with and without contrast, magnetic resonance imaging (MRI) of the cervical spine with and without contrast, CT post myelography, CT of the head and neck with contrast, magnetic resonance angiography (MRA) of the neck with and without contrast, and cervicovertebral angiography.
The indications for imaging the cervical spine after trauma remain a controversial topic. The 2012 revision of the appropriateness criteria for imaging of suspected cervical spine trauma published by the American College of Radiology (ACR) estimates that over 1 million blunt trauma patients present to U.S. emergency rooms with a potential cervical injury each year. Cervical spinal cord injury occurs in 5 of every 10,000 cases per year and is currently the most commonly missed severe injury with serious implications. To improve utilization of radiographic studies in such instances, an extensive review of the literature that included findings from the National Emergency X-radiography Utilization Study (NEXUS) and the Canadian C-spine Rule (CCR) group established criteria that safely excludes cervical fracture without the use of imaging in low-risk patient groups. To do this, patients were divided into groups and the relevance of each of the imaging modalities was analyzed. In the high-risk patient group, CT remains the preferred initial imaging modality.
NEXUS and CCR criteria provide methods for the referring physician to evaluate the need for imaging studies in suspected cervical lesions. Studies show sensitivities ranging from 0.83 to 1.0 and specificities ranging from 0.01 to 0.77 for the criteria; the CCR has slightly better diagnostic accuracy. The NEXUS criteria, published in 2000, were intended to decrease unnecessary radiographic evaluation of the cervical spine in trauma patients. The criteria recommend cervical spine imaging in patients with one or more of these findings: evidence of intoxication, posterior midline tenderness, focal neurologic deficit, clinically apparent distraction injury, or a decreased level of alertness. There are no data available to establish the reliability of NEXUS criteria in young children, owing to radiation exposure concerns in this population.
The CCR criteria are used to evaluate patients who are alert and in stable condition where spinal injury is suspected; patients are categorized into high- or low-risk groups. High-risk patients are older than 65 years, present with the presence of paresthesia, or have a history of a dangerous mechanism of injury, including falls from an elevation greater than 3 feet or 5 stairs, axial load to the head, motor vehicle collision at speeds greater than 100 km/hour (62 miles/h [mph]) or rollover or ejection, a collision involving a motorized recreational vehicle, or a bicycle collision. Indicators of low risk are simple rear-end motor vehicle collision, a patient in the sitting position in the emergency department, ambulation at any time, delayed onset of neck pain, and absence of midcervical tenderness. No imaging is necessary for patients fulfilling low risk-factor criteria who are able to actively rotate the neck 45 degrees from left to right. Similarly, meta-analysis of 14 studies including NEXUS and CCR criteria indicates that asymptomatic patients without a distracting injury who maintain a full range of motion of the cervical spine can be cleared without radiographic evaluation, with a sensitivity of 98% and negative predictive value of 99.8%.
Imaging Modalities
Plain Radiographs
Three-view plain radiographs—anteroposterior (AP), cross-table lateral, and odontoid views—offer a fast and low-cost imaging option but should only be used when the suspicion of injury is low. Plain radiographs may fail to demonstrate lesions visible in other cross-sectional imaging modalities; missed injury rates of 25% to 60% have been demonstrated in plain film radiography. In a study of 10,496 cervical spine injuries conducted by Mower et al., plain radiographs detected 932 injuries in 498 patients and missed 564 injuries in 320 patients. Evidence does not support the use of additional oblique views, and although dynamic views (flexion and extension) have been shown to demonstrate unstable cervical lesions, successful evaluation requires an adequate range of motion, which can be limited in the acute setting. Dynamic supervised views are best used for follow-up of symptomatic patients. Radiography is recommended in children younger than 14 years.
Computed Tomography
CT is the current study of choice for suspected cervical spine injury. CT outperforms plain radiography for evaluation of blunt trauma, with measured sensitivities of 98% compared to 52% for plain films in a meta-analysis of 7 studies of high-risk patients. Newer multidetector CT imaging of the spine with submillimeter acquisitions allows the generation of high-quality multiplanar reformatted (MPR) sagittal and coronal reconstructions without the stairstep artifacts seen with single-detector studies. Although this improves interpretations of CT scans in trauma patients, particularly the ability to differentiate stable from unstable fractures, it also increases the volume of images radiologists are expected to read. Therefore the ACR criteria recommend a slice thickness of 1.25 mm for sagittal and coronal reconstructions.
Because CT scans expose patients to higher mean effective doses of radiation than plain films, these studies should be limited to patients at high risk for cervical injury. The use of multislice CT protocols in modern scanners has contributed to decreases in radiation exposure following CT in recent years.
Magnetic Resonance Imaging
MRI can be used for evaluation of cervical trauma but is typically indicated for suspected myelopathy, treatment planning for the mechanically unstable spine, patients who cannot be clinically evaluated for more than 48 hours, patients with neurologic deficits, or suggested ligamentous injury. The main role of MRI is detection of the type and extent of spinal cord injury, which affects patient management. MRI is better suited for exclusion of spinal cord lesions than detection of ligamentous or other soft tissue injuries. In a 2001 study correlating MRI findings with cadaveric cryosections, more than half of soft tissue injuries were not detected ; however, MRI performs slightly better in detection of clinically significant lesions. No studies compare more current MRI technology with cadaveric studies, but MRI has only a slight advantage in the detection of occult instability injuries not detected on CT. In fact, Pourtaheri et al. found that MRI does not provide any additional clinically relevant information in patients who are alert, neurologically intact, and younger than age 60.
Incidence
The major causes of cervical spine injury in the young population are motor vehicle accidents (MVAs), acts of violence, and sports injuries ; falls are more common in the geriatric population. The cervical spine is the most likely region to fracture following motor vehicle collision ( Fig. 28-1 ). A recent study reviewed the data from 33,015 front-seat occupants sustaining MVAs and found 0.4% had cervical spine fractures, 0.24% had thoracic spine fractures, and 0.3% had lumbar spine fractures. The mean relative collision speed for cervical fractures was 48 km/h (30 mph) compared to 40 km/h (25 mph) for the lower segments. In 2012, the Association for the Advancement of Automotive Medicine reported an increase in incidence of injuries of the thoracolumbar spine in frontal impact crashes, finding burst-type fractures at T12, L1, or L5 and wedge compression deformities commonly at L1. Additionally the elderly are more prone to spinal injury due to MVAs, possibly owing to changes in biomechanical tolerance.
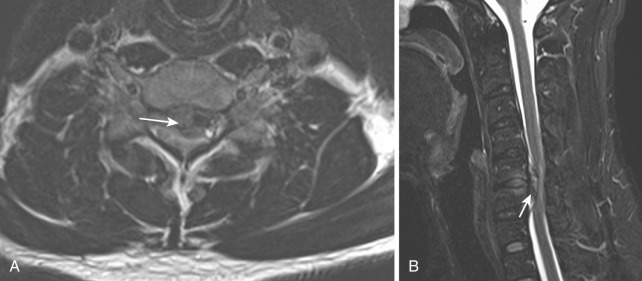
Fractures
The study of cervical spine injuries can be approached based on the mechanism of injury: flexion, extension, compression/axial load, and rotational. Lesions may involve the bony elements, soft tissues (ligaments, joint capsule or peripheral soft tissues), spinal canal, or neural elements including the spinal cord. Injuries of the cervical spine are of great importance owing to the proximity of the spinal cord and other neural structures.
Cervical spine fractures are divided into upper and lower cervical lesions.
Craniocervical Injuries
Upper cervical fractures include structures from the occiput to the second cervical vertebra and may also involve the occipital condyles, the atlas, the odontoid process of C2, the pars interarticularis of C2 (hangman’s facture), and the atlantoaxial joint.
Anatomy of the Craniocervical Junction.
The craniocervical junction (CCJ) has a unique anatomy that makes evaluation of this region challenging. It is crucial to understand the complex morphology to appropriately evaluate potential lesions of this region.
Three bony structures constitute the CCJ: the occiput, the first cervical vertebra (atlas [C1]), and the second cervical vertebra (axis [C2]). These bones articulate in a way that maximizes motion of the spine region while avoiding injury to the neural and vascular components. The structures approximate at two major joints: The atlantooccipital and the atlantoaxial. The biomechanical properties of the atlantooccipital joint depend upon bony structure relationships, whereas the atlantoaxial joint relies on ligamentous relationships.
Bony anatomy.
The occiput has two paired inferior projections called condyles that articulate with the superior articular surfaces of the lateral masses of C1. At the anterior aspect of the base of these condyles, foramina allow the hypoglossal nerve to exit the skull. The next structure, the atlas, is a ring-shaped vertebra with bilateral paired lateral masses fused by an anterior and a posterior arch. It is the only vertebra that does not have a body or a relationship with an intervertebral disk. The posterior aspect of the anterior arch has an articular surface for the odontoid process of C2 (dens). The lateral masses articulate superiorly with the occipital condyles and inferiorly with C2. The superior aspect of the lateral masses has a groove allowing passage of the vertebral artery before it enters the foramen magnum. The final structure is the axis, a unique vertebra that has a superior projection called the dens. The axis has paired superior articular processes to articulate with the lateral masses of the atlas and inferior articular processes to articulate with C3.
Ligamentous anatomy.
The broad complex of ligaments involved in the CCJ can be divided into intrinsic and extrinsic ligaments ( Fig. 28-2 ).
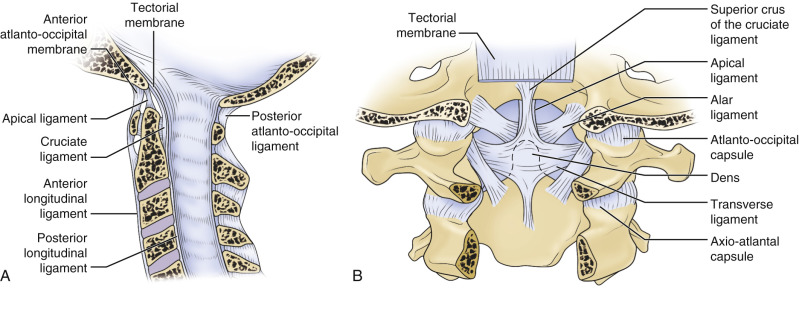
The intrinsic ligaments form three layers anterior to the dura matter and include the tectorial membrane, the cruciate ligament, and the odontoid ligaments. The tectorial membrane is a continuation of the posterior longitudinal ligament that runs posterior to the cruciform ligament. It attaches to the occipital bone and is in intimate contact with the dura matter. The cruciate ligament is composed of the transverse ligament and superior and inferior limbs. The transverse ligament is located in the atlas and traverses the posterior aspect of the dens, fixating it to the posterior aspect of the anterior arch of the atlas. This ligament divides the ring of the atlas into two compartments: an anterior compartment including the dens and a posterior compartment that houses the thecal sac and its contents. The odontoid ligaments comprise the alar and apical ligaments. The alar ligaments are paired structures that attach the base of the skull and the axis at the posterolateral aspect of the odontoid process; they act as stabilizers of the CCJ and limit axial rotation. The apical ligament, also known as the suspensory ligament, joins the tip of the dens with the basion.
The extrinsic ligaments are fibroelastic membranes that replace the anterior longitudinal ligament and the ligamentum flavum. The nuchal ligament is a continuation of the interspinous ligament that attaches to the occipital bone, restricting hyperflexion.
Ligamentous function.
The atlantooccipital and atlantoaxial joints are both necessary for full range of motion. The atlantooccipital permits flexion and extension while limiting rotation, and the atlantoaxial allows rotation while prohibiting other movements. The anatomic morphology of the ligaments in these joints explains the range of motion. Flexion at the atlantooccipital joint is limited by the relationship of the tip of the dens with the basion; extension is limited by the tectal membrane. The alar ligaments at this level minimize axial rotation. Therefore CCJ hyperextension injuries often result in tectal membrane injuries and also can encompass alar ligament injury when rotational force factors into the trauma. The rotational range of motion at the atlantoaxial joint is permitted by the functional relationship of the transverse ligament, alar ligaments, and capsular ligaments.
Special attention has been paid to evaluation of the ligamentous structures of the CCJ using MRI. Thin-slice MRI in three orthogonal planes provides good visualization of clinically relevant ligamentous structures. However, controversy still exists over the value of the imaging findings. Recent studies revealed that MRI signal changes in the alar and transverse ligaments did not correlate with whiplash injuries.
Craniometry.
The normal landmarks of the CCJ are best evaluated in sagittal and coronal cross sections. For accurate craniometrics, the basion, hard palate, and tuberculum sella, which are often excluded from exams, must be visible. However, in the setting of trauma, the usefulness of these measurements is questionable. Interobserver agreement demonstrates that the best correlation between plain film and cross-sectional imaging measurements is the McRae line, extending from the basion to the opisthion. Changes in craniometry secondary to flexion and extension, as well as irregularity of the clivus as seen in cross-sectional imaging, might explain this controversy.
Types of Craniocervical Injury
Atlantooccipital dissociation.
Atlantooccipital dissociation (AOD) can be life threatening, typically resulting from high-energy trauma. Although this is an uncommon injury, in fatal traffic accidents the incidence is as high as 8% to 31%. The high fatality rate results from concomitant brainstem or vascular injury. Three types of AOD have been described; the most common is ventral dislocation of the occiput—type I; the most unstable is axial distraction—type II; and the third is dorsal dislocation of the occiput—type III. The most frequent mechanism of injury that produces AOD includes extreme hyperextension of the neck along with some degree of rotation ( Figs. 28-3 and 28-4 ). There is also an association with AOD and the presence of condylar fractures. Clinically these patients present with neurologic symptoms suggesting brainstem injury, and urgent reduction of the dissociation has been shown to improve long-term survival rates. A higher incidence has been reported in the pediatric population.
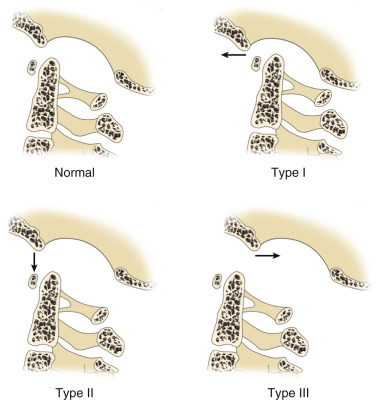
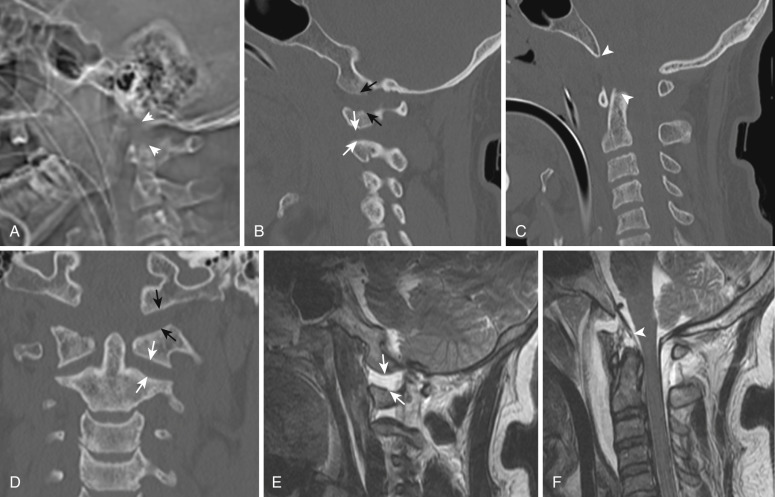
As discussed in previous sections, plain radiographs have limited usefulness in this situation, and MPR CT images are ideally needed for proper visualization of the craniocervical articulation. MRI can be used to identify major ligamentous disruptions and evaluate lesions to the spinal cord. A displacement greater than 10 mm between the basion and the tip of the dens (basion-dens interval) is a reliable method to measure AOD. In a comparison of basion-dens interval, condylar gap, Harris method (basion-atlas index), and the BC : OA Powers’ ratio, Dziurzynki et al. found the basion-dens interval to have the highest sensitivity and specificity for determination of AOD.
Occipital condyle fractures.
Occipital condyle fractures are uncommon lesions in blunt trauma, generally resulting from compression mechanisms. They can be classified into three types: comminuted—type I; extension of a linear skull fracture—type II; and avulsion injury—type III. Condylar fractures may be bilateral in up to 50% of cases. Special attention should be paid to type III condylar fractures because they can be associated with injury of the alar ligament, causing instability of the CCJ ( Figs. 28-5 and 28-6 ).
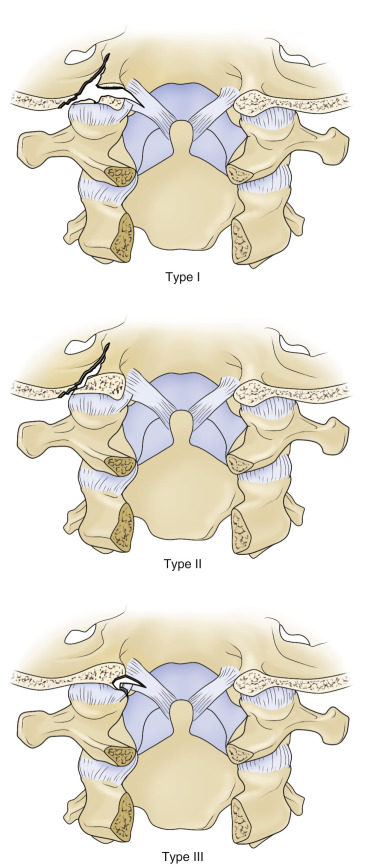
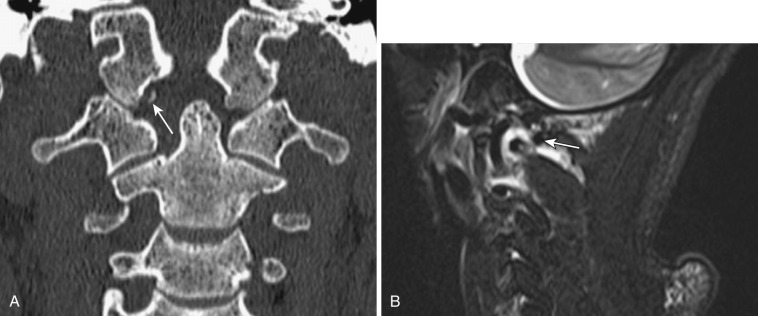
Plain films have a very low sensitivity to detect these lesions; CT is the best diagnostic tool to identify them. MRI can be helpful in identifying ligamentous involvement. With this injury, cranial nerve damage, particularly of the hypoglossal nerve, is often a concern. Close attention should be paid to the other cervical segments when encountering this fracture, because other associated lesions such as type II odontoid, axis, and subaxial fractures may also be encountered. Symptoms tend to improve with cervical traction, the initial treatment of choice.
Fractures of the atlas and transverse ligament rupture.
Fractures of the atlas account for 2% to 13% of acute cervical fractures. Also referred to as Jefferson’s fracture, they typically occur after application of an axial load to the skull, resulting in a burst fracture of the atlas at its weakest points, the anterior and posterior arches. These fractures may be isolated or associated with other lesions such as transverse ligament injury or odontoid fractures ( Figs. 28-7 and 28-8 ). Evaluation of the anterior transverse ligament is essential for the prognosis. Rupture of this structure destabilizes the atlantodental relationship and can result in posterior displacement of the dens in relation to the atlas. If the atlas is considered as a ring, the presence of single fractures is unusual; typically at least two fracture sites will be present. The most common fractures will be (1) posterior arch—associated with hyperextension (2) burst—associated with axial load, and (3) lateral mass fracture—associated with lateral flexion (see Fig. 28-7 ). Isolated C1 fractures tend to not have neurologic compromise, but when displacement of the lateral masses occurs, concomitant rupture of the anterior transverse ligament is possible.
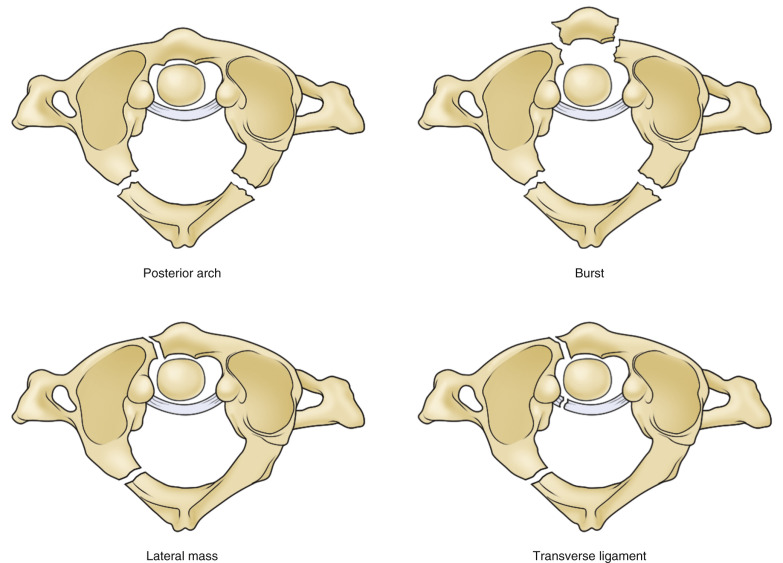
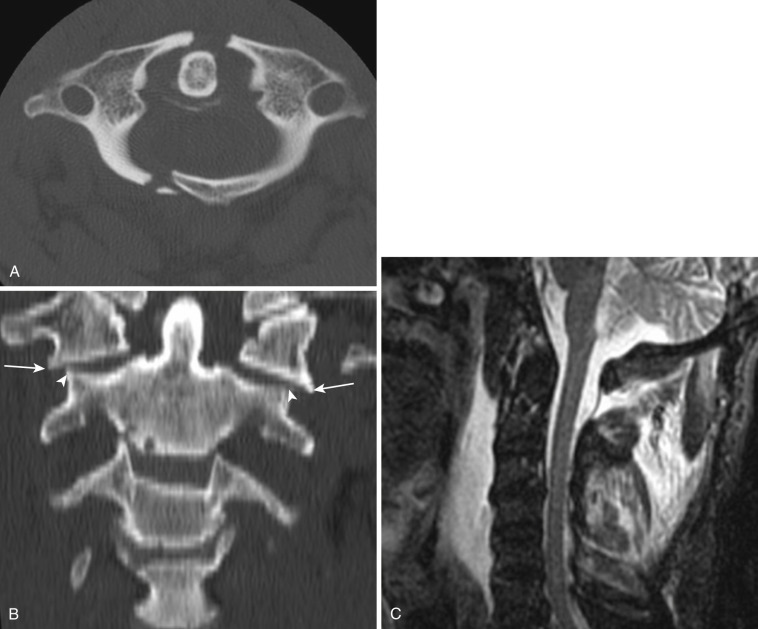
Disruption of the anterior transverse ligament without a fracture of the C1 ring can also occur; these lesions usually are accompanied by disruption of the alar ligaments. They have been classified as type I (lesions of the ligament alone) and type II (presence of avulsed bone fragments).
Evaluation of the atlas typically requires an open-mouth view for a frontal radiograph. A discontinuity of the lateral line that extends from the lateral masses of C1 to C2 would be present. Displacement of more than 7 mm indicates anterior transverse ligamentous injuries. Dynamic (flexion and extension) radiographs can be used as a screening tool. Widening of the atlantoodontoid space would suggest anterior transverse ligament disruption in the trauma setting, but if instability is suspected, dynamic views should be avoided.
CT is helpful for delineating the fracture lines in the atlas. Coronal multiplanar images can demonstrate the discontinuity of C1 and C2 in a similar way to open-mouth radiographs ( Fig. 28-9 ). MRI may be beneficial to study the ligaments, but its usefulness is limited because typical cervical spine MRIs do not cover the atlas in axial views, which hinders evaluation of the anterior transverse ligament. In a normal study the ligaments should be low intensity and homogeneous on gradient recalled echo sequences. Lesions appear as discontinuity of the ligament or significant signal change. MRI has limited use in the initial evaluation of ligamentous involvement of high cervical injuries as well.
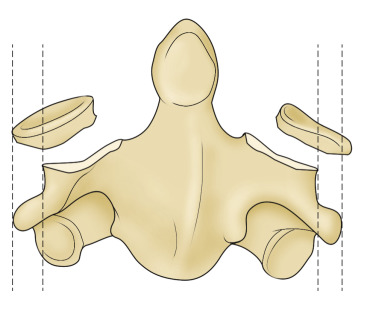
Atlantoaxial rotatory deformity.
Atlantoaxial subluxations are rare events that are usually nontraumatic and can be caused by an incomplete odontoid process or an incomplete transverse ligament; they are highly associated with rheumatoid arthritis. The atlantoaxial articulation is the most mobile portion of the spine, with articular facets that are mostly flat, allowing ample movement in multiple directions. Dislocation of the joint can result in compression of the thecal sac and neural structures. Traumatic rotatory subluxation occurs secondary to flexion and rotation force with lesions of the alar ligament; it is more frequent in children than in adults.
Odontoid fractures.
Odontoid fractures are a common injury in the cervical spine, accounting for 5% to 15% of cervical fractures. They can occur in hyperextension or hyperflexion injuries.
The classification of odontoid fractures by Anderson and D’Alonzo links the location of the fracture line with prognosis. Type I fractures compromise the upper part of the dens; type II fractures involve the base of the odontoid process; type III fractures affect the body of the axis ( Figs. 28-10 and 28-11 ). However, a limitation of the Anderson-D’Alonzo classification is the lack of descriptive terms for fracture lines. Roy-Camille et al. proposed an alternate classification that defines the angle of the fracture and the potential of dens displacement: type 1—oblique linear fracture in which its line slopes forward, with dens displacement in an anterior direction; type 2—oblique linear fracture in which its line slopes backward, with dens displacement in a posterior direction; and type 3—horizontal fracture line, with either anterior or posterior dens displacement.
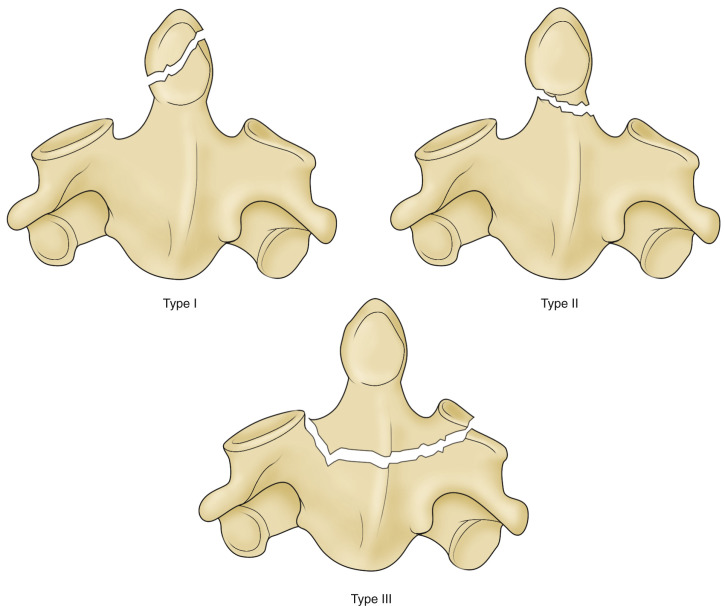
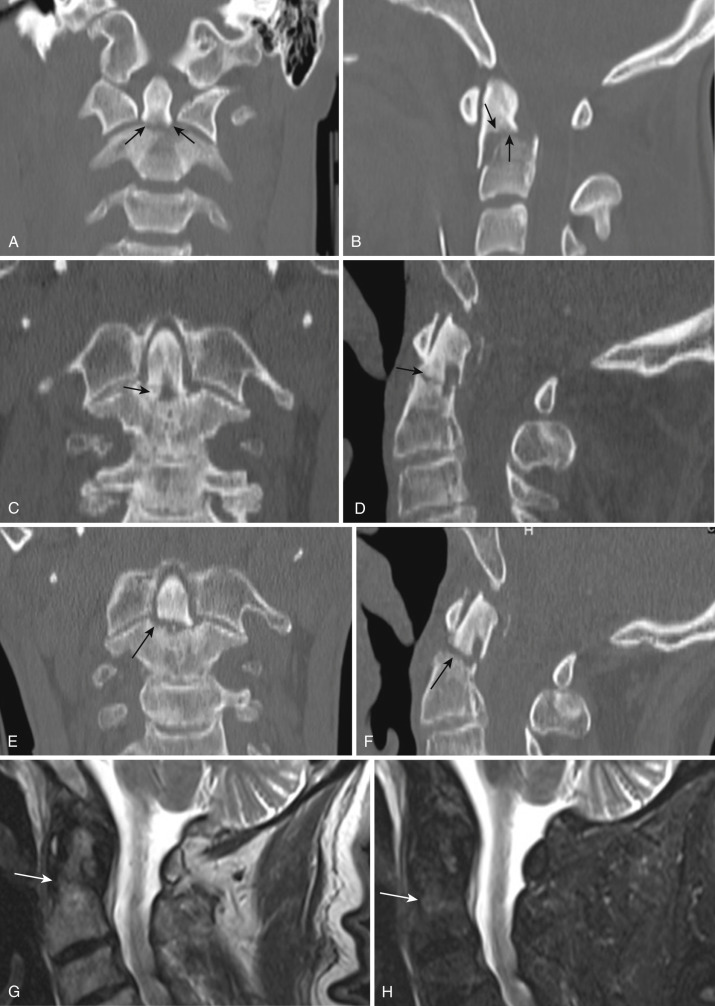
Treatment varies with type of injury. Type II fractures have the lowest potential for healing, especially in the elderly, and there is controversy regarding their management. These fractures may be life threatening if secondary spinal cord lesions occur, but this event is extremely rare. Type II fractures have a nonunion rate ranging from 10% to 77%; risk factors for nonunion include displacement greater than 5 mm, inability to maintain reduction, early initial treatment, and advanced patient age.
Evaluation of nondisplaced odontoid fractures with x-rays is limited. High-resolution CT with multiplanar reformats allows proper visualization of the fracture lines and eases classification, which will be critical for treatment decision making. Most odontoid fractures are treated with halo vest immobilization.
Traumatic spondylolisthesis of the axis.
Traumatic spondylolisthesis of the axis, representing around 15% of cervical spine fractures, is caused by hyperflexion of the cervical spine, producing bilateral fractures of the pars articularis of the axis. This fracture typically occurs in the setting of an MVA and tends to decompress the neural axis; patients are typically stable and have a good prognosis. Traumatic spondylolisthesis is similar in appearance to judicial hanging, which occurs after hyperextension of the neck, fracturing the posterior elements of C2 and causing severe spinal cord compression. The term hangman’s fracture is used loosely in the literature to describe traumatic pars articularis defects of the axis.
The classification of C2 spondylolisthesis takes into account the angulation and translation between C2 and C3. It describes three types: type I—fracture through the neural arch with no deviation; type II—fracture with significant displacement over angulation; type IIA—oblique fractures with angulation, with minimal translation; and type III—fracture of the posterior arch of C2, with unilateral or bilateral facet dislocation ( Figs. 28-12 and 28-13 ). These fractures are rare in the pediatric population and if present, child abuse must be suspected. Difficulty evaluating these children arises because of anatomic variation seen as a line connecting the spinous processes of C1 and C3. CT and MRI are recommended if the diagnosis is not clear.
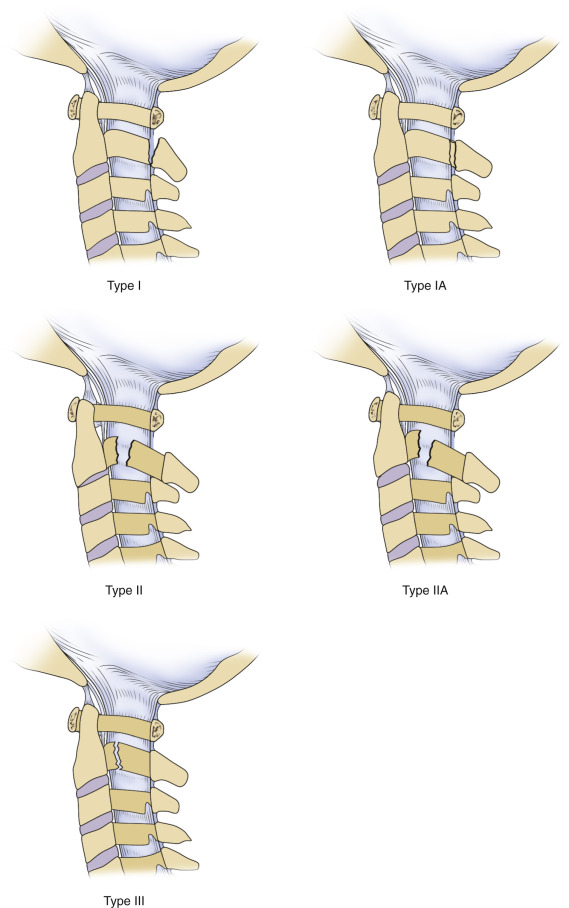
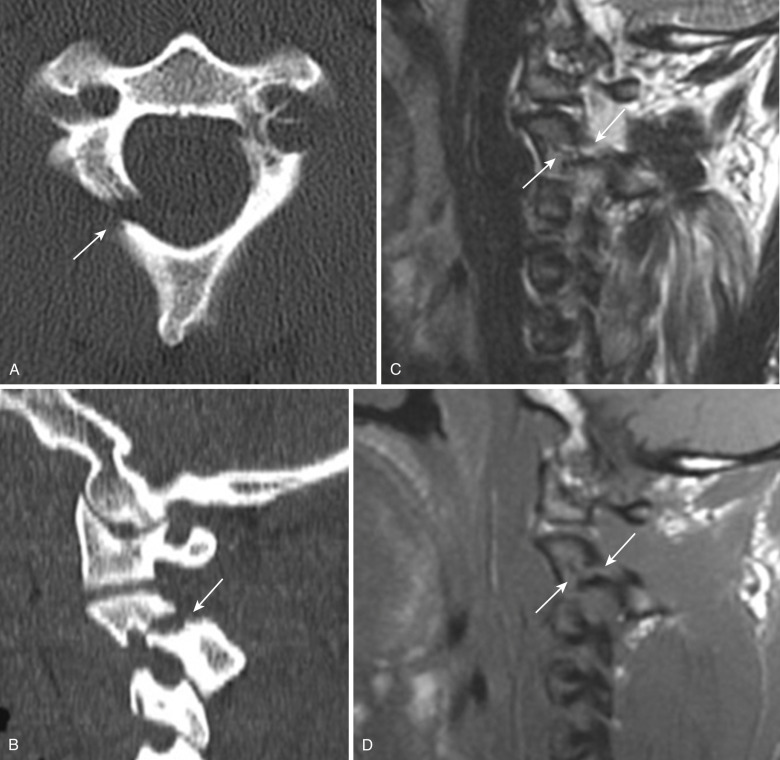
Pain radiographs have demonstrated great reproducibility in characterizing translation and angulation of hangman’s fractures. MRI is helpful to evaluate spinal cord compromise if the fracture is suspected to be secondary to hyperextension. Vertebral artery lesions tend to be more prominent in comminuted C2 fractures that affect the foramen transversarum.
Subaxial Cervical Spine Trauma
The subaxial cervical vertebrae articulate with one another via intervertebral disks between each vertebral body and bilateral zygapophyseal joint facets, which are both flat and obliquely angulated, allowing subluxation. The spinous processes are typically bifid. Each transverse process is pierced by the foramen transversarium. The vertebral arteries enter the foramen transversarium between C7 and C6 and ascend the remaining cervical vertebrae protected within this structure. Ligaments provide structural support in the space between vertebrae, maintaining proper alignment; the ligamentum flavum connects adjacent lamina, and the thick interspinous ligament connects adjacent spinous processes. The neural foramen, at the center of all of these structures, is oriented obliquely and anteriorly.
Greater than 60% of all cervical spine fractures and more than three fourths of the dislocations are subaxial. Different classification systems have been developed, and there is controversy regarding their accuracy and impact on clinical outcome. Most imagers have resorted to using descriptive terminology to characterize patterns of injury. The Allen and Fergusson classification has prevailed as the system most often used for description of fractures. This system groups cervical fractures based on mechanism of injury: compressive flexion, vertical compression, distractive flexion, compressive extension, and lateral flexion. Different stages exist within each group for a total of 21 different possible types. This system does not quantify the severity of a lesion or guide treatment, which is considered a limitation. This classification was expanded in the mid-1980s to include a combination of description and mechanism of injury. Today this expansion is rarely used in clinical practice. Recent efforts to further classify subaxial lesions have been made, giving rise to the subaxial ligamentous injury classification (SLIC; Box 28-1 ) and the cervical spinal injury severity score (CSISS).
