© Springer Berlin Heidelberg 2014
Christoph Mulert and Martha E. Shenton (eds.)MRI in Psychiatry10.1007/978-3-642-54542-9_1717. Structural and Functional Brain Imaging in Borderline, Antisocial, and Narcissistic Personality Disorder
(1)
Department of Clinical Psychology and Psychotherapy, Freie Universität Berlin, Berlin, Germany
(2)
Department of Psychiatry and Psychotherapy, Charité Universitätsmedizin Berlin, Berlin, Germany
Abbreviations
ACC
Anterior cingulate cortex
BOLD
Blood oxygenation level dependant
BPD
Borderline personality disorder
CSP
Cavum septum pellucidum
DTI
Diffusion tensor imaging
GM
Gray matter
NPD
Narcissistic personality disorder
OFC
Orbitofrontal cortex
PCL-R
Psychopathy Checklist-Revised
PET
Positron emission tomography
PFC
Prefrontal cortex (PFC)
PPI
Psychopathic Personality Inventory
PTSD
Posttraumatic stress disorder
SPECT
Single-photon emission computed tomography
VBM
Voxel-based morphometry
17.1 Individuals with Borderline Personality Disorder
17.1.1 Introduction
Borderline personality disorder (BPD) is a serious mental disorder that affects up to 3 % of the population in western nations (Lenzenweger et al. 2007; Trull et al. 2010) and is characterized by a pervasive pattern of instability in affect, behavior, interpersonal relationships, and self-identity (DSM-IV-TR 2000). Dissociation, impulsive aggression, self-injury, and chronic suicidal tendencies are the most prominent clinical symptoms (Leichsenring et al. 2011), resulting in pronounced impairments of psychosocial functioning, even in comparison to patients with mood or other personality disorders (e.g., Ansell et al. 2007; Skodol et al. 2002). Due to severe dysfunctions in their daily lives, BPD patients engage in extensive utilization of mental health resources (Ansell et al. 2007; Bender et al. 2001; Zanarini et al. 2001) and are usually overrepresented in psychiatric in- and outpatient samples (Grilo et al. 1998; Korzekwa et al. 2008; Marinangeli et al. 2000). The course of BPD symptomatology was traditionally considered chronic and intractable. However, this pessimistic outlook has fundamentally changed following recent investigations into the long-term course of BPD symptomatology as well as research demonstrating the effectiveness of specialized psychotherapeutic options (e.g., Bateman and Fonagy 2008; Giesen-Bloo et al. 2006; Linehan et al. 2006). The 10-year course of BPD, for instance, is characterized by high rates of remissions and low rates of relapse. However, BPD patients experience persistent disturbance in social functioning (Gunderson et al. 2011).
Factor analytic studies identified three major domains of psychopathological impairments in BPD (Sanislow et al. 2002; Skodol et al. 2002): affective dysregulation, behavioral dysregulation (i.e., impulsivity), and disturbed relatedness.1 Although disturbance in interpersonal relations is highly characteristic of personality disorders in general, disturbed relatedness in BPD is uniquely characterized by turbulent relationships and an excessive fear of perceived or real abandonment. This specific interactional style was, accordingly, proposed to best discriminate BPD from other personality disorders (Gunderson et al. 1995). Disturbed relatedness is presumably a consequence of presence of traits such as marked rejection sensitivity and pronounced difficulties in recognizing others’ intentions and feelings (Gunderson 2007). Impaired mentalization capabilities are consistently observed in BPD (Bland et al. 2004; Levine et al. 1997; Preissler et al. 2010; Ritter et al. 2011; Unoka et al. 2011). Impairments in mentalizing capabilities are particularly prominent in more naturalistic settings (for a review, see Roepke et al. 2012), e.g., when patients have to integrate facial and prosodic information (Minzenberg et al. 2006) or are required to rapidly categorize facial expressions (Dyck et al. 2009). Along with biases in the evaluation of others (e.g., Arntz and Haaf 2012; Arntz and Veen 2001; Barnow et al. 2009), these aberrations contribute significantly to impaired functioning in interpersonal contexts (for reviews, see Dinsdale and Crespi 2013; Domes et al. 2009). Experience sampling studies provide further evidence that perceived rejection triggers anger and aggression (Berenson et al. 2011), states of tension, as well as the urge to engage in self-injurious behavior (Stiglmayr et al. 2005).
Negative emotional states are much more frequent and longer lasting in BPD patients (Ebner-Priemer et al. 2007; Stiglmayr et al. 2005, 2001), who show marked emotional reactions (even in response to presumably neutral stimuli) along with an aberrant variability in affective states (for a review, see Rosenthal et al. 2008). Correspondingly, the diagnostic criterion of affective dysregulation is the most frequent and stable criterion in BPD (Glenn and Klonsky 2009; Gunderson et al. 2011; McGlashan et al. 2005). Abnormalities in the processing and regulation of emotions are therefore widely considered to be “at the core of borderline pathology” (Stiglmayr et al. 2005, p. 372). Importantly, most clinical symptoms in BPD are directly related to affective dysregulation. Self-injurious behavior in BPD, for instance, is reportedly used to escape from undesired or extreme emotions (Chapman et al. 2006; Kleindienst et al. 2008) and, thus, might represent an efficient, but highly dysfunctional means of regulating aversive emotional states (Klonsky 2007; Niedtfeld and Schmahl 2009). Furthermore, affective instability was found to be related to feelings of emptiness, interpersonal problems, and identity disturbances in BPD (e.g., Kleindienst et al. 2008; Klonsky 2007; Koenigsberg et al. 2001; Tragesser et al. 2007).
Impulsive behaviors in BPD such as unsafe sexual practices, gambling, reckless driving, substance abuse, or disordered eating (e.g., Soloff et al. 2000; Trull et al. 2000) might also reflect dysfunctional attempts to cope with negative emotionality (Brown et al. 2002; Yen et al. 2004). Several studies, however, provided evidence that affective and behavioral dysregulation are distinct phenotypic traits of BPD (Tragesser and Robinson 2009; Zanarini et al. 2005). That is, impulse control difficulties predicted unique features of BPD (even when controlling for affective instability). Thus, it was argued that impulsivity must be considered independently from the affective domain to better understand the range of BPD symptoms (for a discussion, see Tragesser and Robinson 2009).
The prominent psychopathological impairments of BPD provided impetus for increased research investigating the neurobiological correlates of BPD symptomatology. The following sections will focus on summarizing available findings from the structural and functional neuroimaging literature (for a review of PET studies, see Lis et al. 2007). First, we will start with a short overview of structural brain abnormalities. Then, findings of functional neuroimaging will be presented and are organized with respect to the major psychopathological domains of BPD: processing and regulation of emotions, self-injurious behavior and pain processing, as well as interpersonal disturbances.
17.1.2 Structural Neuroimaging in BPD
Previous studies on brain structure in BPD used manual tracing methods, which allow the precise detection of small volume differences in a set of a priori-defined brain regions. The majority of these studies were interested in structural properties of the limbic system and found significantly smaller gray matter volume in the amygdala and hippocampus (Driessen et al. 2000; Rusch et al. 2003; Schmahl et al. 2003; Tebartz van Elst et al. 2003), although other studies failed to find group differences in amygdala volume (Brambilla et al. 2004; Zetzsche et al. 2006). Discrepancies regarding structural findings of the limbic system were argued to be partially due to different comorbidities in the investigated BPD samples. Recent meta-analyses aimed to clarify this point and found smaller gray matter volumes in the bilateral amygdala and hippocampus of about 13 and 11 %, respectively (Nunes et al. 2009; Ruocco et al. 2012). Interestingly, abnormalities of gray matter volume in these regions were independent of comorbid disorders or treatment experience. Accordingly, gray matter abnormalities of the limbic system were argued to serve as “candidate endophenotypes” of BPD (Ruocco et al. 2012). This interpretation, however, should be treated with caution as it is based on only a few studies with small sample sizes. Smaller gray matter volumes of the limbic system are, for example, also common in patients with posttraumatic stress disorder (PTSD; for a meta-analysis, see Karl et al. 2006) or in women with a history of traumatization (Dannlowski et al. 2012). Both conditions are highly prevalent in BPD (Lieb et al. 2004) and might contribute to abnormalities in the limbic system of BPD patients. Up until now, just one study evaluated the effects of co-occurring PTSD in BPD patients (Schmahl et al. 2009). In this study, smaller hippocampal volumes were only found in BPD patients with PTSD, thereby highlighting the necessity to disentangle the respective disorders’ effects on structural brain abnormalities.
Apart from the limbic system, structural brain abnormalities were also evaluated in the frontal lobe of BPD patients (e.g., Hazlett et al. 2005; Sala et al. 2011; Tebartz van Elst et al. 2003). Particularly the advent of voxel-based morphometry (VBM) in recent years allowed an unbiased quantification of whole-brain structural properties rather than just of a few selected brain regions (Mechelli et al. 2005). Available VBM studies illustrated group differences in gray matter in the anterior cingulate cortex, the dorsolateral prefrontal cortex, as well as the orbitofrontal cortex (e.g., Brunner et al. 2010; Sato et al. 2012; Soloff et al. 2012; Vollm et al. 2009) while replicating smaller gray matter volume of the limbic system (Kuhlmann et al. 2012; Rusch et al. 2003). Prefrontal abnormalities of BPD patients were also related to impulsivity and suicidality (Sala et al. 2011; Soloff et al. 2008, 2012; Vollm et al. 2009).
While the investigation of structural white matter integrity in BPD is still in its infancy, available results highlight abnormalities in anterior parts of the corpus callosum and the bilateral orbitofrontal cortex (Carrasco et al. 2012) as well as in the anterior cingulate cortex, which are characterized by diminished interhemispheric structural connectivity (Rusch et al. 2010). Reduced integrity of inferior frontal white matter circuits was more specifically related to the BPD symptomatology, such as impaired affect regulation and enhanced impulsivity (Grant et al. 2007; Rusch et al. 2007).
In sum, findings of previous morphometric studies point predominantly toward abnormalities in limbic and prefrontal brain regions in BPD, especially in the amygdala and hippocampus, as well as in parts of the anterior cingulate cortex, dorsolateral prefrontal cortex, and orbitofrontal cortex. Structural properties of these regions might serve as biological markers to discriminate patients with BPD from healthy controls on the single-subject level (Sato et al. 2012).
17.1.3 Functional Neuroimaging in BPD
17.1.3.1 Processing and Regulation of Emotions
BPD is characterized by a pervasive pattern of emotional instability that is directly related to many aspects of patients’ psychopathology, e.g., self-injury, interpersonal disturbance, and identity disturbance. Given the pivotal role of emotional instability in BPD symptomatology, this endophenotype is a major target for current treatment approaches, which aim to provide patients with techniques supporting the identification and regulation of negative emotional states. These include mentalization-based therapy (Bateman and Fonagy 2008) and dialectical behavior therapy (for a meta-analysis see Kliem et al. 2010). Emotional instability is theorized to be the result of two factors: (a) an enhanced emotional reactivity and (b) an inability to control intense negative emotional states (Koenigsberg 2010; Minzenberg et al. 2008; Posner et al. 2003). The review of available neuroimaging findings will be accordingly subdivided, although one should keep in mind that the line between emotion processing and emotion regulation is blurry at best as the generation of emotional responses is already subject to (implicit) modulations by regulatory-oriented processes (Gross 1998, 2002).
In accordance with two-factor models, BPD patients described more intense responses to emotionally evocative stimuli and exhibited greater instability of emotions in both laboratory and naturalistic settings (Ebner-Priemer et al. 2007; Herpertz et al. 1997; Rosenthal et al. 2008; Stiglmayr et al. 2005). Experimental studies provided further support for impaired inhibition of negative stimuli (Domes et al. 2006; Silbersweig et al. 2007), deficits in the disengagement of attentional resources from negative facial expression (von Ceumern-Lindenstjerna et al. 2010), and a hypervigilance to emotional cues (Arntz et al. 2000), especially in the presence of BPD-specific cues (Sieswerda et al. 2007).
At the neural level, most studies find support for abnormalities in the emotion processing circuitry of patients with BPD (for a detailed description of brain regions underlying emotion processing, see, e.g., Kober et al. 2008; Murphy et al. 2003; Tsuchiya and Adolphs 2007). An initial study by Herpertz et al. (2001), for instance, presented patients with aversive social scenes and found a link between patients’ experience of intense emotions and enhanced activations of the bilateral amygdala. Stronger neural activity was also found in the bilateral fusiform gyrus, which might reflect an increased vigilance for negative emotional stimuli, as the visual cortex has dense connections to the amygdala (Amaral et al. 2003). Subsequent work found enhanced reactivity of the left amygdala in BPD in response to pictures of facial expressions of emotions, regardless of their specific valence (Donegan et al. 2003), whereas another study demonstrated a more specific pattern of limbic hyperarousal. In this study, enhanced activity of the right amygdala in BPD was only observed in response to fearful compared to neutral faces, whereas attenuated activation of the bilateral amygdala was found during the presentation of angry facial expressions (Minzenberg et al. 2007).
Overall, available evidence in BPD is consistent with the conclusion that limbic and paralimbic hyperreactivity holds particularly for negative and neutral stimuli (e.g., Jacob et al. 2012; Niedtfeld et al. 2010; Schulze et al. 2011), whereas the existing results regarding the processing of positive stimuli are to date still inconclusive. Koenigsberg and colleagues (2009b) found that compared to healthy controls, BPD patients exhibited heightened activity of the limbic system only in response to negative stimuli; they failed to find group differences for positive images. They also observed greater activation of the dorsolateral prefrontal cortex during the presentation of negative stimuli in healthy controls. In contrast, Hazlett et al. (2012), as well as Donegan et al. (2003), found greater amygdala activity in BPD patients across all picture types, including positive stimuli. Additionally, recent findings suggest abnormalities in emotion processing might affect the functioning of the reward circuitry in BPD (i.e., the ventral striatum, the pregenual anterior cingulate, and the ventromedial prefrontal cortex) and the functioning of the cortical midline structures during the anticipation of reward (Enzi et al. 2013).
Although most studies primarily investigated the processing of facial expressions or social scenes, heightened negative emotionality in patients with BPD has been demonstrated across a wide range of different stimuli. For instance, Jacob et al. (2012) reported stronger left-lateralized activation of the amygdala, along with attenuated activation of the subgenual anterior cingulate during an auditory anger induction procedure. Similarly, BPD patients showed stronger activation of the amygdala and insular region while recalling unresolved autobiographic life events (Beblo et al. 2006) or reading negatively valenced words (Silbersweig et al. 2007). Exaggerated and temporally prolonged amygdala responses were also observed in BPD patients during a procedure in which patients were presented with simple squares paired with painful electrodermal stimulation while being scanned (Kamphausen et al. 2013).
However, as outlined above, heightened emotional response represents only one facet of emotional instability in BPD. Impairment in the cognitive control of intense emotional states is argued to be equally important for understanding the disturbance patients experience in their daily lives, as the ability to regulate emotions has important effects on physical and mental health (Davidson 2000; Eftekhari et al. 2009; Gross and John 2003). As a consequence, studies of neural mechanisms associated with emotional regulation have rapidly increased in the last years. These studies have examined several different forms of cognitive control, ranging from attentional control processes, such as (in)voluntary shifts of attentional focus or limited allocation of resources necessary for the processing of external stimuli, to cognitive change via reappraisal (for reviews, see Ochsner and Gross 2005; Ochsner et al. 2012). The impact of cognitive reappraisal on neural activity in the limbic and paralimbic regions is observed across a complex prefrontal network involving multiple neural regions; however, the impact is particularly observable in the dorso- and ventrolateral, orbitofrontal, and anterior cingulate regions (e.g., Banks et al. 2007; Domes et al. 2010; Johnstone et al. 2007; Ochsner et al. 2004; Wager et al. 2008). The variability of prefrontal localizations associated with cognitive reappraisal might be attributable to temporal aspects of the reappraisal process, which first involves implementation and subsequently involves maintenance and monitoring of the effects of reappraisal on emotional responses (Kalisch 2009).
Recent studies of BPD patients investigated the effects of two different strategies of cognitive reappraisal: psychological distancing and reinterpretation of emotional situations. In one of the first neuroimaging studies of this phenomenon conducted by Koenigsberg et al. (2009a), patients were asked to decrease their emotional response to negative visual scenes using psychological distancing strategies. Compared to healthy controls, BPD patients showed difficulties engaging the dorsal anterior cingulate cortex and inferior parietal lobe during conditions of psychological distancing while showing a paradoxical increase of neural activity in the right amygdala. In another study conducted by Schulze et al. (2011), a delayed reappraisal paradigm was used to more clearly distinguish between the initial emotional reactivity to a negative stimulus and the subsequent modulation of emotional responses by cognitive reappraisal (Jackson et al. 2000). Statistical analyses during the initial viewing phase replicated previous results of limbic and paralimbic hyperreactivity during the presentation of negative and neutral stimuli in BPD. With regard to the regulatory phase, BPD patients showed attenuated activations of the left (more lateral) orbitofrontal cortex, along with attenuated reduction of activity in the bilateral insula during attempts to voluntarily decrease their emotional reactions. The orbitofrontal cortex is thought to play an important role in successful reappraisal via altering and updating the context-sensitive relevance of stimuli (Ochsner et al. 2004; Rolls 2000), suggesting specific deficits in BPD patients’ ability to implement new appraisals for the presented stimuli. Overall, the results by Schulze et al. (2011) support two-factor models of emotional instability in BPD, i.e., enhanced emotional responding and deficits in cognitive regulation of emotions. Interestingly, neurofunctional abnormalities in regions associated with the processing or regulation of emotions were only prominent when participants were instructed to attenuate negative emotions, whereas no group differences were found when participants attempted to increase their emotions. Additionally, recent work aimed to disentangle the specific effects of BPD from those of traumatization on the neural correlates of cognitive reappraisal (Lang et al. 2012). In this study, healthy participants without any history of traumatization showed an initial increase in prefrontal activation (e.g., dorsolateral and dorsomedial prefrontal regions, anterior cingulate cortex), whereas trauma-exposed BPD patients and a comparison group of healthy individuals with traumatic experiences showed initial deactivations in those regions. Intriguingly, the group differences in the initial phase of emotion regulation might also indicate specific impairments in the implementation and generation of alternative appraisals (Kalisch 2009). In addition, the results highlight the necessity to more adequately address individual differences in BPD, e.g., trauma exposure, PTSD, or treatment experience.
Several studies examined attentional control (i.e., behavioral inhibition) of BPD patients in the context of negative stimuli (e.g., Domes et al. 2006; Fertuck et al. 2006; Rentrop et al. 2008). At the neural level, deficits in the inhibition of behavioral responses in a linguistic Go/No-Go task, for instance, were related to relatively decreased activity in the ventromedial prefrontal cortex (including orbitofrontal and subgenual anterior cingulate regions) compared to healthy controls (Silbersweig et al. 2007). Attenuated activations of the anterior cingulate cortex during response inhibition were also observed in an emotional Stroop paradigm (Wingenfeld et al. 2009). While these studies assessed inhibition and negative emotions in general, recent work also addressed how performance on an impulse control task is modulated by more specific emotional states (Jacob et al. 2012). Listening to an anger-inducing story, BPD patients showed a stronger activation of the right amygdala and the nucleus subthalamicus, whereas healthy controls showed a stronger recruitment of the subgenual anterior cingulate cortex. The subsequent performance on a Go/No-Go task was accompanied by attenuated activation of the left inferior frontal cortex in BPD.
Finally, two studies investigated the influence of emotional distraction on working memory performance. In both studies, BPD patients showed longer reaction times during emotional distraction along with enhanced activations of the amygdala compared to healthy controls (Krause-Utz et al. 2012; Prehn et al. 2013b). Moreover, limbic and paralimbic activity was negatively correlated with current states of dissociation, suggesting that dissociation has a dampening effect on emotional reactivity (Krause-Utz et al. 2012). Dissociation is considered a regulatory strategy to cope with overwhelming emotions, presumably via prefrontal inhibition of limbic regions (Lanius et al. 2010; Sierra and Berrios 1998). While dissociation represents a highly efficient way to shut down the emotional system (Ebner-Priemer et al. 2005, 2009), such states also prevent patients from learning to implement new strategies in emotionally arousing situations and represent a major challenge for psychotherapeutic treatment of BPD (Kleindienst et al. 2011).
The study by Prehn et al. (2013b) further assessed the role of working memory load on emotion processing. Despite heightened distractibility by highly negative stimuli in general, healthy controls and criminal offenders with BPD showed equivalent declines of limbic activity associated with manipulations affecting working memory load. In other words, the engagement in a secondary cognitively challenging task downregulates amygdala activity, presumably because these tasks draw resources necessary for the processing of emotional stimuli (see also Kanske et al. 2011; Van Dillen et al. 2009). Hence, attentional deployment strategies might be particularly useful for attenuating emotional reactions in BPD.
To summarize, findings of functional neuroimaging studies support two-factor conceptualizations of emotional instability by illustrating abnormalities in the processing and regulation of emotions. Comparing negative emotionality between patients with BPD and healthy controls, enhanced activation of the amygdala and insular region, along with attenuated activation of the rostral part of the anterior cingulate cortex, was consistently reported. As stated initially, the boundary between processing and regulation of emotions is blurry at best (Gross 1998, 2002), and quite congruently, self-reports of emotional regulation capabilities correlate positively with neural activity in limbic and paralimbic regions (Niedtfeld et al. 2010; Schulze et al. 2011). Preliminary results also suggest dialectical behavior therapy—focusing particularly on improving emotion regulation—attenuates neural activity of limbic regions in response to emotionally arousing stimuli (Schnell and Herpertz 2007), providing a promising starting point for assessing the effects of specific treatment modules on affective hyperarousal in BPD. With regard to the cognitive control of emotions, BPD seems to be characterized by an attenuated functioning of the dorsal part of anterior cingulate cortex and medial and lateral parts of the orbitofrontal cortex and deficient activation of the dorsolateral prefrontal cortex. Therefore, dysfunctional behaviors, such as self-injury or dissociation, might be best understood as an attempt to initiate inhibitory control of intense emotions via increased activation of the prefrontal regions, e.g., dorsolateral prefrontal cortex (Krause-Utz et al. 2012; Niedtfeld et al. 2012; see also next paragraph).
17.1.3.2 Self-Injury and Pain Processing
Deliberate self-injurious behavior, such as cutting or burning, is one of the most prominent clinical symptoms observed in BPD patients, with rates as high as 90 % observed in some studies (Skodol et al. 2002; Zanarini et al. 2008). Patients frequently state that they use non-suicidal self-injurious behavior to escape from undesired or extreme negative emotions (Chapman et al. 2006; Kleindienst et al. 2008). They also experience an immediate relief of tension, a decrease of dissociative symptoms, and elevations of mood (Herpertz 1995; Kemperman et al. 1997; Reitz et al. 2012). Self-injury was therefore proposed to represent a dysfunctional attempt to regulate negative emotional states directly corresponding to emotional instability in BPD (Klonsky 2007; Welch et al. 2008).
Patients with BPD display a reduced sensitivity to painful stimulation across different nociceptive modalities (e.g., Cardenas-Morales et al. 2011; Magerl et al. 2012; Russ et al. 1992; Schmahl et al. 2004), which is further decreased under conditions of elevated stress or states of dissociation (Bohus et al. 2000; Ludascher et al. 2007). Conversely, discontinuation of intentional self-injurious behavior results in a normalization of pain perception (Ludascher et al. 2009). The reduced sensitivity to pain, however, is not attributable to impairments in the sensory-discriminative component of pain processing, as BPD patients did not exhibit abnormal performance in the spatial discrimination of nociceptive stimuli or in the detection of painful stimulation (Ludascher et al. 2007; Schmahl et al. 2004). Therefore, BPD patients’ reduced sensitivity might be better explained by abnormalities in the affective-motivational component of pain sensation, which in turn can be modulated by the context and cognitive appraisals of pain. Importantly, recent work by Magerl et al. (2012) found that endogenous antinociception in BPD is best predicted by the recency of self-injurious behavior, whereas psychometric measures of BPD psychopathology were unrelated to pain sensitivity. Thus, the authors suggested reduced pain sensitivity might not be a consequence of BPD psychopathology per se, but rather the result of neurofunctional rearrangement of brain circuits involved in the processing of pain due to BPD-related self-injurious behavior.
Neural models of pain sensation identified two anatomically distinct neural pathways that might be implicated in BPD patients’ self-injurious behavior—sensory discriminative and affective motivational (for a review, see Treede et al. 1999). The sensory-discriminative system, necessary for stimulus localization and perception of pain quality and intensity, projects from the lateral thalamic nuclei to the primary and secondary somatosensory cortices. In contrast, the affective-motivational pathway, which underlies evaluation of pain and subsequent emotional or behavioral reactions, projects from the medial thalamic nuclei to the anterior cingulate cortex and insula, which is in turn reciprocally connected to the brainstem and the amygdala (Augustine 1996; Barbas et al. 2003).
Neurobiological correlates of altered pain processing in BPD were initially investigated by Schmahl and colleagues (2006). In this study, heat stimuli of two different intensities were delivered via a thermode. Participants either received a standardized temperature of 43 °C or an individual temperature adjusted for equal subjective pain in all individuals. Here, BPD patients again showed much higher pain thresholds than healthy controls. In response to individually adjusted heat stimuli, patients showed neural deactivation in the perigenual anterior cingulate cortex and the amygdala, while greater BOLD responses were found in the dorsolateral prefrontal cortex. Therefore, antinociceptive mechanisms might be attributable to increased top-down control closely associated with deactivations of the limbic system, particularly in patients with comorbid posttraumatic stress disorder (Kraus et al. 2009). Subsequent work combined thermal sensory stimulation with the prior induction of negative affect to further elucidate the potential role of pain with respect to regulation of affective states in BPD (Niedtfeld et al. 2010). In line with previous work, the BPD sample showed enhanced activations of the amygdala, insula, and anterior cingulate cortex in response to negative (but also to neutral) stimuli. The subsequent administration of heat stimulation again served to suppress neural activation of the amygdala and anterior cingulate cortex. Interestingly, there was only limited support for a BPD-specific role of pain in affect regulation, as both groups showed comparably attenuated activity in the amygdala. As a result, the authors proposed a general mechanism to explain the soothing effects of pain. In particular, they argued that painful sensory stimulation might draw resources from the processing of negative emotions, similar to cognitive strategies of “attentional deployment” (Gross 2002; Ochsner and Gross 2005). Additional connectivity analyses indicated that inhibitory influence on limbic regions during painful stimulation is particularly attributable to activation of the medial and dorsolateral parts of the prefrontal cortex (Niedtfeld et al. 2012).
In a different study, participants were presented with an audiotape describing an act of self-injury comprising different aspects of the situation, such as the respective trigger, related cognitive and emotional reactions, the act of self-harming behavior, and the subsequent relaxation (Kraus et al. 2010). While listening to emotional reactions, BPD patients displayed attenuated activation of the orbitofrontal cortex in comparison to healthy controls, presumably due to impaired ability to inhibit or modulate the elicited emotions. The description of the self-injurious act itself was again accompanied by decreased activity of the mid-cingulate cortex in BPD.
Alternative accounts of self-injury highlight the importance of the endogenous opioid system (for reviews, see Bandelow et al. 2010; New and Stanley 2010; Stanley and Siever 2010). The human opioid system consists of β-endorphin, met-enkephalin, and dynorphin, with all three neurotransmitters broadly involved in antinociception and stress-induced analgesic states (Fields 2004; Flor et al. 2002). More specifically, it is suggested that several forms of dysfunctional behavior in BPD (e.g., self-injury, risk-seeking behavior, promiscuity, substance abuse) might reflect attempts to raise relatively low levels of opioids (Bandelow et al. 2010; New and Stanley 2010). Reduced availability of dynorphin might also explain feelings of chronic emptiness and dysphoria (Land et al. 2008; for a discussion, see Stanley and Siever 2010). An initial investigation into this phenomenon found lower levels of cerebrospinal fluid β-endorphins and met-enkephalins in individuals with a history of self-injury, mainly patients with BPD (Stanley et al. 2010). These findings were paralleled by a PET study measuring μ-opioid receptor availability during neutral and dysphoric states (Prossin et al. 2010). At baseline, greater μ-opioid receptor availability in BPD was found in the orbitofrontal cortex, caudate nucleus, nucleus accumbens, and amygdala, presumably reflecting lower levels of endogenous opioids. The induction of sad affect led to a greater activation of the opioid system in the anterior cingulate cortex, orbitofrontal cortex, ventral pallidum, and amygdala, indicating a compensatory response to basal levels of μ-opioid in BPD.
To summarize, theoretical conceptualizations propose that self-injury is a dysfunctional, but highly efficient strategy for the regulation of negative emotional states. Higher pain thresholds in BPD are not attributable to impairment in the sensory-discriminative component of pain processing, but rather to abnormalities in affective-motivational components. The results of imaging studies further bolster such conceptualizations. In response to pain, patients with BPD show enhanced activation of the prefrontal cortex, along with attenuated activation of the anterior cingulate cortex and limbic system, whereas no functional abnormalities are found in somatosensory cortices. Therefore, the soothing effects of self-harm might be best explained by an increased top-down control of limbic regions through medial and dorsolateral prefrontal regions. Further emphasis must be placed on understanding associations between self-injury and the opioid system. Given that recent findings indicate that BPD patients exhibit baseline deficits in endogenous opioids, this area might hold some promise as a target for pharmacotherapy in BPD.
17.1.3.3 Interpersonal Disturbances
Disturbed relatedness was acknowledged in earliest descriptions of BPD (Kernberg 1967), and patients’ difficulties in interpersonal contexts are currently considered the most unique and discriminant feature of BPD psychopathology (Gunderson et al. 1995). Up until the present, studies in this area have focused predominantly on abnormalities in the perception and recognition of others’ mental and emotional states as a social-cognitive factor contributing to disturbance in BPD patients’ social interactions (for a review of social cognition in BPD, see Roepke et al. 2012). Consistent with this notion, most behavioral studies found that BPD patients exhibit impairment in basic emotion recognition (e.g., Bland et al. 2004; Levine et al. 1997; Merkl et al. 2010; Ritter et al. 2011; Unoka et al. 2011) as well as a bias to interpret ambiguous stimuli more negatively (Domes et al. 2008; Meyer et al. 2004; Wagner and Linehan 1999), although a number of studies also reported superior attribution and detection of mental states in BPD (Fertuck et al. 2009; Lynch et al. 2006; Schulze et al. 2013a). Deficits in the recognition of emotions were particularly prominent in more complex and naturalistic settings (for a discussion of the role of ecological validity, see Roepke et al. 2012), e.g., when subjects have to integrate facial and prosodic information (Minzenberg et al. 2006), are required to rapidly discriminate facial expressions (Dyck et al. 2009), or have to judge video-based social interactions (Preissler et al. 2010; Sharp et al. 2011). In sum, these studies point predominantly toward impairments in BPD patients’ capacity to infer the emotional states of other individuals.
However, cognitive empathy represents only one facet of social cognition (Decety and Meyer 2008; Singer 2006); a second component represents the emotional response to the observed emotional state of another person, i.e., emotional empathy (Eisenberg and Miller 1987; Mehrabian and Epstein 1972). The few experimental studies available on the subject found that BPD patients exhibit a reduced capacity to experience empathic concern for other people’s observed distress (Dziobek et al. 2011; Ritter et al. 2011). In combination with aforementioned findings of deficits in cognitive empathy, these findings suggest that misperceptions of others’ emotional states might lead to dysfunctional emotional responses in social interactions (New et al. 2008).
Consequently, recent neuroimaging studies have aimed to identify functional brain abnormalities associated with impaired cognitive empathy in BPD. An initial study by Guitart-Masip et al. (2009) investigated patients’ brain responses while discriminating emotional facial expressions from neutral expressions. Compared to healthy controls, BPD patients made more mistakes in discriminating between negative and neutral facial expressions. More specifically, patients less accurately discriminated between disgusted and neutral expressions and showed a trend for similar difficulties in distinguishing between fearful and neutral faces. These behavioral impairments in BPD were accompanied by greater activation of the left inferior and middle temporal cortex (including parts of the superior temporal gyrus), possibly reflecting abnormalities in the perceptual processing of visual stimuli.
Subsequently, Dziobek et al. (2011) used a more ecologically valid paradigm to measure functional neural correlates of social cognition in BPD. The Multifaceted Empathy Test (MET; Dziobek et al. 2008) depicts people in emotionally charged situations and allows a separate assessment of cognitive and emotional aspects of state empathy. Participants are required to either infer the mental states of the depicted person (cognitive empathy) or to rate their level of empathic concern for the displayed individual (emotional empathy). Behaviorally, patients showed impairments in cognitive and emotional aspects of empathy compared to healthy controls. At the neural level, both groups activated a similar neural network during empathic processes, which comprised the temporal pole, the temporoparietal junction, the orbitofrontal cortex, and the superior temporal gyrus for cognitive empathy (for a review, see Frith and Frith 2005) and, in addition, the insula and medial prefrontal cortex for emotional facets of empathy. Subsequent group comparisons revealed significantly reduced activation of the left superior temporal sulcus and gyrus of BPD patients while inferring others’ mental states. Moreover, this reduction was related to symptoms of intrusion in the BPD group. In contrast, enhanced activation of the right mid-insula was found when patients were emotionally attuned to another person. This brain abnormality was additionally associated with emotional arousal, as assessed by skin conductance responses.
Abnormalities in the left superior temporal sulcus of BPD patients were also found by Mier et al. and by Frick et al. (2012). In the former study, neural responses were measured while participants performed basic or more complex social-cognitive tasks. Healthy controls showed stronger activation of the superior temporal sulcus in response to greater demands on social-cognitive processes; such a pattern, however, was not seen in BPD patients. Hypo-activation of this brain region was consequently particularly prominent when patients performed complex social-cognitive tasks, i.e., attributing others’ intentions. Furthermore, the authors found that BPD patients exhibited hyperresponsiveness of the limbic system independent of the specific social-cognitive process investigated. In line with these findings, Frick and colleagues (2012) also observed attenuated activation of the superior temporal sulcus and enhanced activation of the right amygdala during a mental state discrimination task (based on the eye region of individuals only). In this study, patients with BPD were more accurate in their ability to discern others’ intentions than nonclinical controls (see also Scott et al. 2011).
Taken together, available studies illustrate abnormalities in BPD patients’ ability to infer others’ mental and emotional states, with traumatization being a negative predictor of social-cognitive abilities in BPD (Dyck et al. 2009; Preissler et al. 2010). Neuroimaging studies of mentalization found consistently altered functioning of the superior temporal sulcus in BPD. The superior temporal sulcus might be particularly vulnerable to the impact of traumatic experiences, as this brain region matures rather late in adolescence (Paus 2005). Alternative accounts propose that emotional hyperreactivity in concert with deficient functioning of the prefrontal cortex might interfere with social-cognitive processes (for a discussion, see Domes et al. 2009). The data provided by Dziobek et al. (2011) support the hypothesis that emotional arousal interferes with empathic processes in BPD. Thus, abnormalities related to the processing and regulation of emotions might be linked to altered social-cognitive processes in BPD patients.
As illustrated above, up until now, studies investigating social cognition in BPD primarily focused on the ability of BPD patients to accurately recognize others’ emotions and intentions, i.e., cognitive empathy. Such abilities, however, only represent a small part of successful interpersonal interactions, which are characterized by an ongoing exchange of social signals between individuals (cf. Dziobek 2012). For that reason, recent studies adopted multiplayer economic exchange games to model interactional behavior in BPD (see also Seres et al. 2009). In multi-round trust games without any feedback about the back transfer of the other partner, BPD patients transferred smaller amounts of monetary units compared to nonclinical controls or patients with major depression (Unoka et al. 2009). Impaired trust during these sequential interactions was also related to individual difficulties in interpersonal relationships.
Despite the prominence of interpersonal disturbances in BPD, there is to date only one study available that examined the underlying neurofunctional correlates. King-Casas and colleagues (2008) used a multi-round economic exchange game with functional neuroimaging to investigate interactional behavior in patients with BPD. Their findings suggested that interpersonal disturbances in BPD are related to difficulties in maintaining cooperation with a healthy partner as well as an impaired capacity to restore broken cooperation. Neurologically, abnormal activation patterns of the bilateral anterior insula differentiated healthy controls from individuals with BPD. While activation of the insula in healthy controls tracked changes in cooperative behavior, in patients with BPD, activity of the anterior insula was unrelated to the magnitude of offers received (input) in BPD. Importantly, no insular abnormalities were found to be related to repayment of partners (output). Thus, the attenuated activity observed in the anterior insula during input led the authors to propose that “anomalous [perception of] social ‘input’ … (rather than ‘output’) is a potential mechanism of interpersonal dysfunction” (King-Casas and Chiu 2012; p. 121), particularly with regard to disturbed perception of social norms and an inability to recognize cues signaling waning trust of partners.
17.1.4 Summary
To recapitulate, structural brain imaging findings consistently illustrate smaller gray matter volume in the amygdala and hippocampus of BPD patients. Furthermore, available evidence suggests that BPD patients exhibit structural abnormalities in prefrontal brain regions, such as the anterior cingulate cortex, dorsolateral prefrontal cortex, and orbitofrontal regions.
Functional neuroimaging studies also support frontolimbic abnormalities across a variety of tasks. Processing of emotionally arousing stimuli, for instance, was associated with enhanced activation of the amygdala. BPD patients’ attempts to cognitively control affective states were found to be less effective than those of healthy controls, which is presumably the result of attenuated functioning of prefrontal regions, specifically the dorsolateral prefrontal cortex, as well as medial and lateral regions of the orbitofrontal cortex. Interestingly, dysfunctional behaviors, such as self-injury or dissociation, were found to increase activation of prefrontal regions. Consequently, the soothing effects of self-harm on intense affective states might be best explained by an increased top-down control of limbic regions by medial and dorsolateral prefrontal regions.
Finally, several studies addressed interpersonal disturbances in BPD patients and investigated neural processes associated with inferring others’ mental and emotional states. Findings highlight abnormal functioning of the superior temporal sulcus and additionally suggest that patients’ heightened emotional arousal interferes with social-cognitive processes.
17.2 Individuals with Antisocial Behavior and Psychopathic Traits
17.2.1 Introduction
Empirical research on antisocial behavior has identified a number of genetic, environmental, psychological, and social pathways that potentially lead to these behaviors (Holmes et al. 2001; Moffitt 2005; Murray and Farrington 2010; Raine 2002; Vermeiren et al. 2002). Additionally, a growing body of evidence has linked antisocial behavior to functional and structural brain abnormalities. Any attempt to summarize this literature has several significant limitations. Most important of which is the lack of one common and coherent underlying psychopathological construct. Several studies are based on one of two official classification systems, the DSM and the ICD, which already show little overlap in constructs that capture antisocial behavior. For example, antisocial personality disorder in DSM-IV-TR and dissocial personality disorder in ICD-10 were found to be least concordant, when compared to all other personality disorders (Ottosson et al. 2002). However, studies in child or adolescent populations are mainly based on a different DSM-IV-TR construct; conduct disorder, often with an additional specifier for callous; and unemotional traits (Viding et al. 2012). Other studies focus on very specific aspects of antisocial behavior, e.g., violence (Raine et al. 1997; Volkow et al. 1995), aggressive and impulsive behavior (Dolan et al. 2002), or pathological lying (Yang et al. 2005). The most developed construct capturing antisocial behavior is psychopathy (Hare 2006). Psychopathy is characterized by a cluster of interpersonal, affective, and behavioral characteristics including impulsivity, callousness, and persistent antisocial behavior with profound lack of guilt or remorse (Hare 2006). A further limitation associated with summarizing the current literature is the diverse characteristics of study samples used to research antisocial behaviors. Included populations range from university or community samples with self-reported psychopathic traits (e.g., assessed with the Psychopathic Personality Inventory, PPI; Lilienfeld and Andrews 1996) to nonclinical temporary agency employees to incarcerated men with full criteria psychopathy (e.g., assessed with Psychopathy Checklist-Revised, PCL-R, Hare 2003). Further, study populations vary widely in sample size, gender ratio, education level, cognitive abilities, age, and comorbid conditions, e.g., duration of substance use disorder. A further limitation is that a number of publications refer to the same study population.
Possibly due to these limitations, functional and structural brain findings do not present a consistent picture underlining antisocial behavior and trait psychopathy (Koenigs et al. 2011; featured review). Although most fMRI studies assessed the processing of social or emotional information (e.g., within decision making, conditioning, or reward designs), differences in activation patterns are widespread across all four lobes of the cortex and subcortical structures (Koenigs et al. 2011; review). Also, structural brain findings have not yet provided robust replicable data. Because of these inconsistencies in current data, in this chapter, we focus on specific brain structures and functional networks that are theory driven and have been associated with antisocial behavior and trait psychopathy, presenting promising starting points for both understanding the neural mechanisms underlying these constructs and guiding future research.
17.2.2 Amygdala
Very early studies in individuals with psychopathic traits revealed that these patients reported decreased fear and showed decreased autonomic responses to aversive stimuli, results which both point toward involvement of the amygdala (Lykken 1957; Patrick et al. 1993). Indeed, one of the most robust functional MRI findings across a variety of tasks is attenuated activity of the amygdala. Thus, during an affective memory task, criminal psychopaths (N = 8, PCL-R ≥ 28) from a maximum security prison showed significantly less affect-related activity in the amygdala compared to controls (Kiehl et al. 2001). In an aversive delay conditioning paradigm, criminal psychopaths (N = 4, mean PCL-R = 25) showed attenuated amygdala response compared to control groups (Veit et al. 2002). While performing an affect recognition task within a group of male college students (N = 20), those with high PPI scores (mean split) displayed lower amygdala activation compared to the low PPI group. In later studies, lower amygdala activation has been confirmed in a number of conditions, e.g., by a group of prison inmates (N = 16, PCL-R ≥ 30) viewing pictures depicting moral norm violations (Harenski et al. 2010), during acquisition in a fear-conditioning paradigm in a group of 10 criminal psychopaths (mean PCL-R = 25, Birbaumer et al. 2005), and by a group of schizophrenic patients with trait psychopathy from a secure psychiatric inpatient facility (group composition determined by a mean split of the PCL screening version score, Hart et al. 1995) while viewing fearful faces (Dolan and Fullam 2009). Further, in nonclinical samples, psychopathy score (PPI) correlated negatively with amygdala activation during a prisoner’s dilemma task (Rilling et al. 2007) and while viewing pictures of aversive stimuli (N = 10 females, psychopathy assessed with the PPI; Harenski et al. 2009). Lower amygdala activity during passive avoidance learning was also found in youth (N = 15) with psychopathic traits (PCL youth version (YV) ≥ 20, Finger et al. 2011). Glenn et al. (2009) found that reduced activity in the amygdala during an emotional moral decision-making task correlated with a higher psychopathy score in a sample of 17 community participants with varying degrees of psychopathy (PCL-R = 7.4–32). Also in youth with callous unemotional traits (N = 12, PCL:YV ≥ 20), amygdala activation was reduced compared to control groups while processing fearful faces (Marsh et al. 2008). Further, Jones et al. (2009) found reduced amygdala activation while viewing fearful faces in boys (N = 17) with callous unemotional traits (assessed with the antisocial process screening device, Frick and Hare (2001)) compared to controls.
Nevertheless, there are also contradictory findings. Müller et al. (2003), for instance, found increased amygdala activation in criminal psychopaths from a high-security psychiatric facility (N = 6, PCL-R > 30) while viewing pictures with negative content. Convergent evidence for involvement of the amygdala in antisocial behavior is provided by studies looking for structural alterations in the brains of antisocial individuals. For instance, reduced amygdala volume has been found in unsuccessful psychopaths (defined as PCL-R ≥ 23 and history of prosecution for criminal acts, N = 16; Yang et al. 2010) recruited from temporary employment agencies compared to controls. An additional study with 27 individuals recruited from temporary employment agencies with high psychopathy scores (PCL-R ≥ 23) also confirmed amygdala volume reduction in the high psychopathy group compared to a control group; however, this reduction was more associated with affective and interpersonal facets of psychopathy than the impulsive and behavioral symptoms (Yang and Raine 2009). Further, this study assessed amygdala deformation, which was most prominent in basolateral, lateral, cortical, and central nuclei in participants with psychopathy (Yang and Raine 2009). Finally, in a study including a large number of male prison inmates (N = 296), reduced volume in the amygdala was associated with psychopathy score (measured with the PCL-R; Ermer et al. 2012).
In contrast to the results of reduced amygdala volume, a study of 26 violent offenders from forensic psychiatric hospitals meeting criteria for antisocial and dissocial personality disorder revealed larger amygdala volume compared to a control group (Boccardi et al. 2011). Volume reduction in psychopaths was only found in the basolateral amygdala (a region with reciprocal connections to the orbitofrontal cortex—OFC). The central and lateral nuclei, in contrast, were enlarged (presenting components of the threat circuit that is involved in fear conditioning, Boccardi et al. 2011). A limitation of these results is that all offenders in this sample met the criteria for substance abuse (Boccardi et al. 2011).
In sum, most functional MRI studies point toward a deficit in amygdala activation in trait psychopathy compared to controls, Further, amygdala volume seems to be reduced, at least in some subnuclei, in individuals with trait psychopathy. However, to date there is a lack of studies combining functional and structural analyses.
17.2.3 Prefrontal Cortex
Early findings of antisocial behavior in individuals with prefrontal brain damage pointed to the potential involvement of this brain structure (Anderson et al. 1999; Blumer and Benson 1975; Harlow 1868). Initial fMRI data confirmed this hypothesis and revealed that the orbitofrontal and ventromedial (OFC/vm) and prefrontal cortex (PFC) were particularly implicated in psychopathy (for review see Anderson and Kiehl 2012). In particular, the aforementioned study by Rilling et al. (2007) revealed lower OFC activity in participants with higher psychopathy scores when choosing to cooperate in a prisoner’s dilemma task. Also, the previously mentioned Finger et al. (2011) study showed reduced OFC activity in youth with callous and unemotional traits in response to early stimulus-reinforcement exposure and to reward in a passive avoidance learning task. Criminal offenders (N = 10) with dissocial personality disorder (PCL-R > 28) in a forensic setting also showed decreased prefrontal cortex activation while engaged in a task designed to assess the influence of emotion on cognitive processes (Muller et al. 2008). Results from a study in which ten male psychopathic patients (mean PCL:SV = 16) from a forensic setting and healthy controls engaged in a competitive reinforcement fMRI task revealed that psychopathic patients had lower activation in the mPFC during retaliation, but increased activation in the mPFC when seeing an opponent being punished compared to controls (Veit et al. 2010). Further, reduced activity of the mPFC has been observed when psychopaths (22 men with history of severe criminal offense and PCL-R > 20) are engaged in moral reasoning compared to controls (Pujol et al. 2012). In addition, one study of ten nonclinical female adults (psychopathy measured with PPI) found a negative correlation between psychopathy score and mPFC activation while engaged in fMRI tasks presenting pictures of moral norm violation (Harenski et al. 2009). In line with these findings, lack of vmPFC activation while engaged in a moral norm violation paradigm has been found in 16 male prison inmates with psychopathy (PCL-R ≥ 30) compared to controls (Harenski et al. 2010).
Decreased prefrontal activity was also found during a decision-making task in 12 offenders with antisocial personality disorder and emotional hypo-reaction recruited from a high-security forensic facility (Prehn et al. 2013a). Finally, Birbaumer et al. (2005) also found reduced OFC activation in schizophrenic patients with trait psychopathy during the acquisition phase in a fear-conditioning paradigm. Notably, not all fMRI experiments revealed reduced PFC activation in individuals with antisocial behavior and trait psychopathy. For instance, an fMRI study by Sommer et al. (2010) using a theory of mind task revealed increased activation of OFC and mPFC in 14 criminal patients from a forensic setting with antisocial personality disorder and PCL-R score > 28 compared to controls.
Additionally, structural data support the argument that volume reduction in PFC structures is linked to antisocial behavior. In a study by Raine et al. (2000), 21 men with antisocial personality disorder recruited from temporary employment agencies showed reduced gray matter volume compared to controls. Boccardi et al. (2011) found a reduction in OFC gray matter. Tiihonen et al. (2008) also found OFC gray matter atrophy in 26 forensic patients fulfilling criteria for antisocial and dissocial personality disorder. Also, the study by de Oliveira-Souza et al. (2008) found gray matter reduction in the OFC in patients with antisocial personality disorder compared to controls. Further, volume reduction in individuals with antisocial behavior and trait psychopathy has been found in the anterior frontopolar region of the PFC (de Oliveira-Souza et al. 2008; Gregory et al. 2012; Tiihonen et al. 2008). Assessing cortical thickness in 27 nonclinical psychopaths (recruited from temporary employment agencies, PCL-R ≥ 23) revealed a higher inverse correlation between cortical thickness in the OFC and response perseveration in psychopaths compared to controls (Yang et al. 2011). A recent meta-analysis of 43 structural and functional brain imaging studies (also including PET and SPECT studies) of antisocial, violent, and psychopathic individuals revealed prefrontal structural and functional reduction, especially in the right OFC and left dlPFC compared to controls (Yang and Raine 2009).
Despite the large number of consistent findings, a study by Schiffer et al. (2011) revealed that reduced gray matter volumes in the PFC/OFC were more associated with substance use disorder than with violent behavior. Consistent with this finding, a study by Laakso et al. (2002) found that volume reduction in the dlPFC, OFC, and mPFC in 24 forensic patients with antisocial personality disorder was better explained by duration of alcohol consumption than by trait psychopathy.
17.2.4 Frontolimbic Circuitry
Based on the distinction between reactive and instrumental aggression (Berkowitz 1993), one prominent model (Blair 2010) integrates amygdala and PFC findings in individuals with antisocial behavior and trait psychopathy. Within the framework of this model, reactive aggression follows perceived frustrating or threatening events and is defined as an unplanned and enraged attack against these triggers. Instrumental aggression, on the other hand, is more goal directed and purposeful. Instrumental aggression is thus conceived as a premeditated means of obtaining a goal, other than harming the victim, and being proactive rather than reactive. Psychopathic individuals can show both reactive and instrumental aggression (Anderson et al. 1999). Individuals who predominately engage in reactive aggression are hypothesized to exhibit increased responsiveness of the amygdala-hypothalamus-periaqueductal gray threat system, which might be accompanied by reduced frontal regulatory activity. Findings in patients with predominately reactive aggression confirmed the hypothesis of increased amygdala activity in response to threat stimuli compared to controls (e.g., individuals with impulsive aggression in Coccaro et al. 2007; spouse abusers in Lee et al. 2008). Also, individuals with borderline personality disorder (BPD) show more reactive aggression, and fMRI studies of BPD patients confirm the expected corresponding hyperresponsiveness of the amygdala in this population (Donegan et al. 2003; Herpertz et al. 2001; Koenigsberg et al. 2009a; Minzenberg et al. 2007). In line with the theory of reactive and instrumental aggression, Prehn et al. (2013b) found increased amygdala activity in a study investigating the influence of emotional stimuli on working memory in 15 male criminal offenders from a forensic setting with antisocial personality and comorbid BPD, all meeting the affective instability and lack of anger control criteria. However, it must be pointed out that the cited fMRI studies in individuals with predominately reactive aggression did not consistently show reduced frontal regulatory activity.
Individuals who engage in predominantly instrumental aggression are hypothesized to show specific impairment of the amygdala-dependent stimulus-reinforcement learning and impairment of the prefrontal cortex-related representation of reinforcement expectancies (for a review, see Crowe and Blair 2008). As outlined above, a number of fMRI studies confirmed reduced amygdala and OFC/vmPFC activation in individuals with psychopathic traits or antisocial behavior. Nevertheless, some inconsistent findings must be noted (e.g., lack of differences between psychopaths and controls in OFC activation while viewing emotional faces, Finger et al. 2008; Gordon et al. 2004
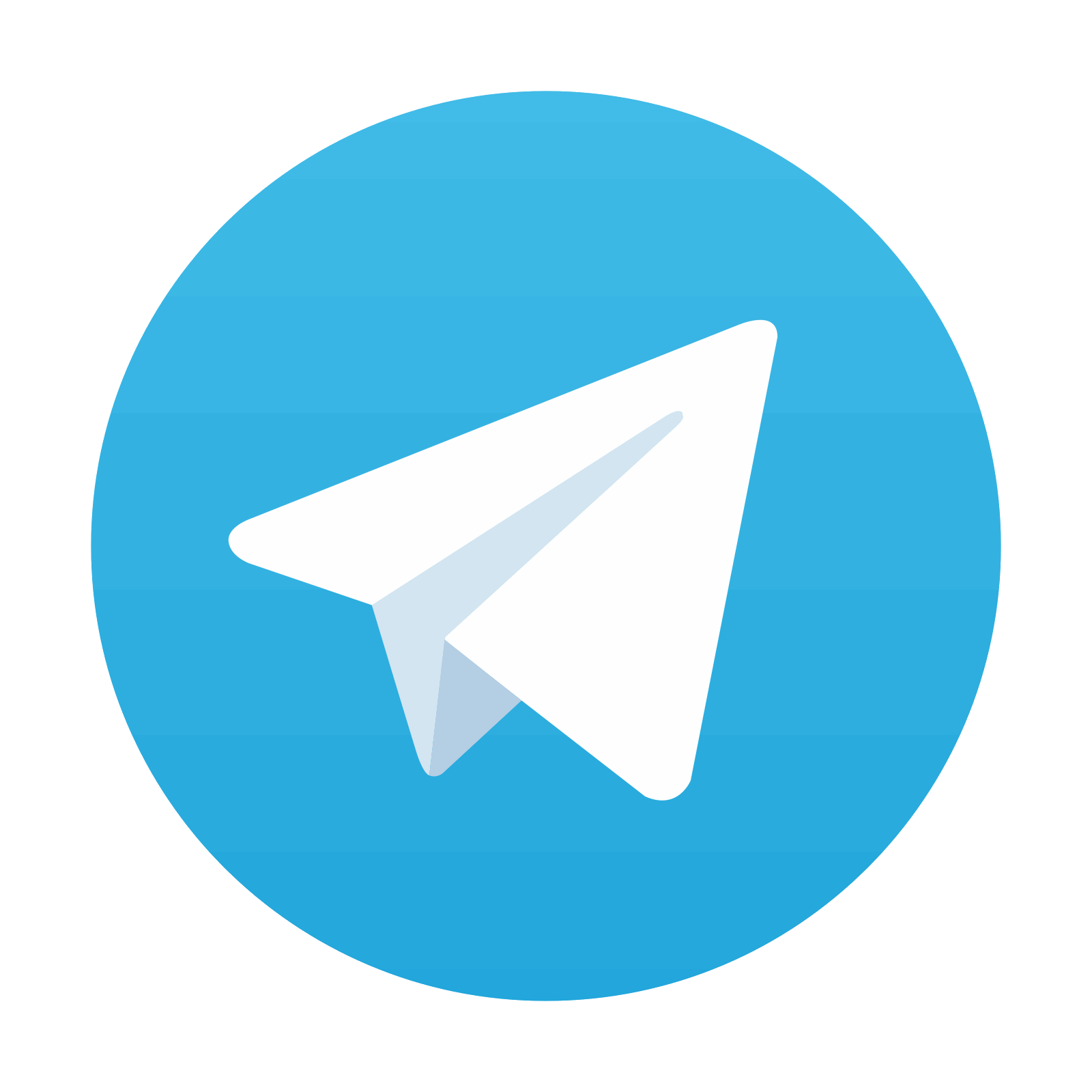
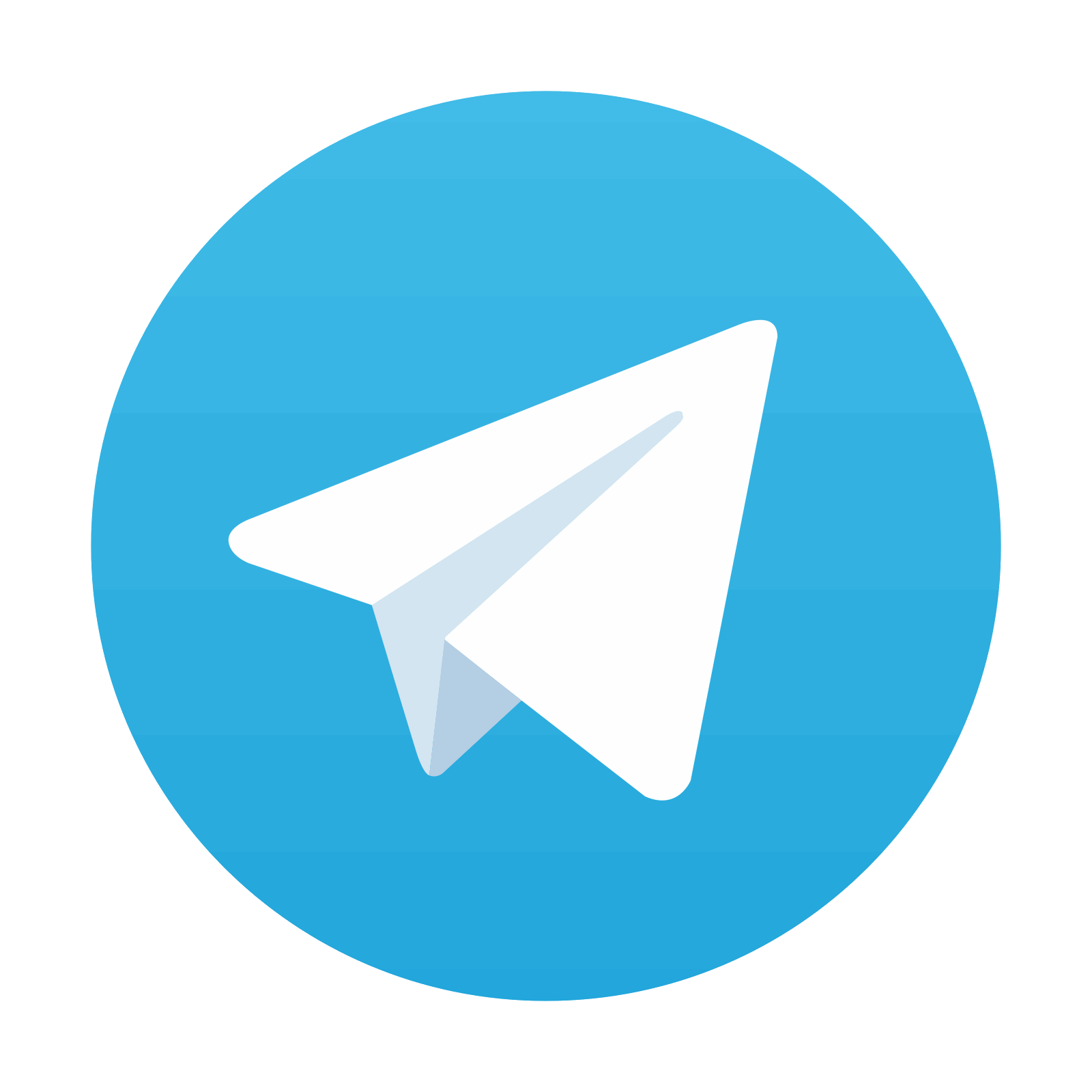
Stay updated, free articles. Join our Telegram channel

Full access? Get Clinical Tree
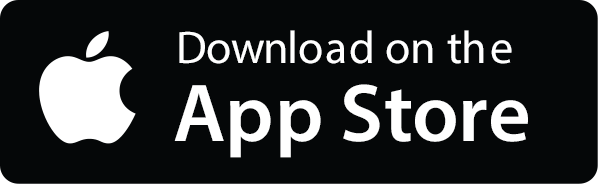
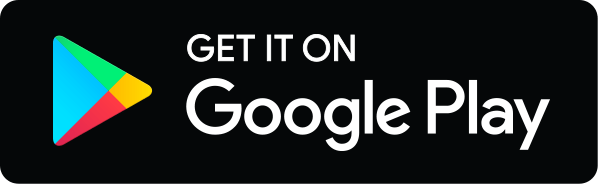
