Fig. 1.
T 1-weighted MR images and confocal laser scanning microscopy (CLSM) images taken from the carotid artery of mice injected with collagen-targeted paramagnetic micelles. These micelles were equipped with the high-affinity collagen-ligand CNA35 or a low-affinity ligand (mutant-CNA35), as a control. The MR images show a strong increase in signal intensity in the arterial vessel wall 24 h after CNA35 micelles injection (black, solid arrow head) compared to the pre-injection scan. CLSM fluorescence images show the carotid artery (24 h after injection) that can be identified by the elastic laminae (white, solid arrowhead). A strong accumulation of CNA35 micelles inside the vessel wall can be seen (white, double, open arrow heads). To stress the differences in accumulation between CNA35- and mutant-CNA35 micelles, fluorescence images are also shown with triple laser power, revealing nearly no accumulation for the latter group. The white, single, open arrowhead indicates the lumen. Adapted from (10).
Here, a comprehensive protocol is described for the preparation and functionalization of paramagnetic and superparamagnetic micelles. The proposed micelle formulations were proven to be successful in detecting several different disease processes in a non-invasive manner. This protocol should also enable the reader to choose other formulations in a well-motivated manner, depending on the specific study objectives. This protocol therefore lays the basis for the preparation of a class of highly versatile particles for molecular imaging.
2 Materials
2.1 Paramagnetic Micelles
1.
Chloroform (this is a potentially toxic solvent and carries danger of serious damage to health by prolonged exposure through inhalation and if swallowed. Chloroform should be handled in a fume hood).
2.
Methanol (this is highly flammable and toxic by inhalation, in contact with skin and if swallowed. Methanol should be handled in a fume hood).
3.
HEPES-buffered saline (HBS) 1×: 2.38 g/L HEPES, 8.0 g/L NaCl in water, pH 6.7 (see Notes 1 and 2).
4.
Gadolinium-DTPA-bis-stearyl-amide (Gd-DTPA-BSA) (Gateway Chemical Technology, St. Louis, MO, USA).
5.
1,2-Distearoyl-sn-glycero-3-phosphoethanolamine-N-[methoxy(polyethylene glycol)-2000] (ammonium salt) (DSPE-PEG2000) (Lipoid GmbH, Ludwigshafen, Germany).
6.
1,2-Distearoyl-sn-glycero-3-phosphoethanolamine-N-[maleimide(polyethylene glycol)-2000] (ammonium salt) (DSPE-PEG(2000) maleimide) (Avanti Polar Lipids, Alabaster, AL, USA).
7.
1,2-Dipalmitoyl-sn-glycero-3-phosphoethanolamine-N-(lissamine rhodamine B sulfonyl) (ammonium salt) (Rhodamine-PE) (Avanti Polar Lipids, Alabaster, AL, USA).
8.
Pear-shaped round-bottom flask, 250–500 mL (Schott AG, Mainz, Germany).
9.
Parafilm® M (Pechiney Plastic Packaging, Menasha, WI, USA)
10.
Rotary evaporation equipment Rotavapor R-200 with coupled water bath (Büchi AG, Flawil, Switzerland).
2.2 Superparamagnetic Micelles
1.
Chloroform (this is a potentially toxic solvent and carries danger of serious damage to health by prolonged exposure through inhalation and if swallowed. Chloroform should be handled in a fume hood).
2.
HEPES-buffered saline (HBS) 1×: 2.38 g/L HEPES, 8.0 g/L NaCl, pH 6.7 (see Note 2).
3.
Iron oxide particles in hydrophobic solvent, e.g., magnetite in toluene as produced by thermal decomposition (11). Iron oxide particles can also be purchased as powder or dissolved in non-polar organic solvent (e.g., BioPAL, Worcester, MA, USA). Attention should be paid to the hydrophobicity of the coating present on the magnetite core.
4.
1,2-Distearoyl-sn-glycero-3-phosphoethanolamine-N-[methoxy(polyethylene glycol)-2000] (ammonium salt) (DSPE-PEG2000) (Lipoid GmbH, Ludwigshafen, Germany).
5.
1,2-Distearoyl-sn-glycero-3-phosphoethanolamine-N-[maleimide(polyethylene glycol)-2000] (ammonium salt) (DSPE-PEG(2000) maleimide) (Avanti Polar Lipids, Alabaster, AL, USA).
6.
1,2-Dipalmitoyl-sn-glycero-3-phosphoethanolamine-N-(lissamine rhodamine B sulfonyl) (ammonium salt) (Rhodamine-PE) (Avanti Polar Lipids, Alabaster, AL, USA).
7.
5 mL chloroform-resistant container and lid.
8.
Glass syringe, Luer lock tip, 5 mL (Poulten & Graf, Wertheim, Germany).
9.
Stainless steel needle, non-coring point, 6 inch, 21 gauges (Sigma Aldrich, Zwijndrecht, the Netherlands).
10.
Syringe infusion pump (Pump 11 Pico Plus, Harvard Apparatus, Holliston, MA, USA).
11.
Ultrasonicator (model 5510, Branson, Danbury, CT, USA).
12.
Vivaspin 10,000 MWCO concentrator, 20 mL (Sartorius Stedim, Göttingen, Germany) (see Note 3).
13.
Ultracentrifuge (Optima L-90 K, Beckman Coulter, Fullerton, CA, USA).
2.3 Coupling Ligands
1.
Succinimidyl S-acetyl-thiol-acetate (SATA) (toxicity of SATA has not been determined; therefore it should be handled in a fume hood).
2.
N,N-Dimethylformamide (DMF) (this compound is harmful by inhalation and contact with skin or eyes, may cause harm to the unborn child. DMF should be handled in a fume hood).
3.
Ligand for targeting, e.g., antibody or peptide.
4.
HEPES-buffered saline 1×, 2.38 g/L HEPES, 8.0 g/L NaCl in water, pH 7.4.
5.
Vivaspin concentrator (Sartorius Stedim, Göttingen, Germany) (see Note 3).
6.
Filter buffer: 2.38 g/L HEPES, 8.0 g/L NaCl, 0.37 g/L ethylenediamine tetraacetic acid (EDTA), pH 7.0.
7.
De-acetylation buffer: add to 50 mL of water, 1.74 g hydroxyl amine (this is harmful when it comes into contact with the skin, use gloves), 5.95 g HEPES, 0.47 g ethylenediamine tetraacetic acid (EDTA), 1 g NaOH, pH 7.0. Prepare fresh.
2.4 Particle Characterization Methods
2.4.1 Particle Size Determination
1.
Dynamic light scattering equipment (Malvern Zetasizer Nano S, Malvern Instruments, Malvern, UK).
2.
Sterile filtered buffer, which has the same composition as the buffer containing the functionalized micelles.
2.4.2 Phosphate Determination
1.
70% perchloric acid (this compound is corrosive, will cause severe burns, and is very harmful by inhalation or ingestion or through skin contact. Perchloric acid should be handled in a fume hood).
2.
Stock solution 50 mM sodiumbiphosphate (NaH2PO4·2H2O) (Fluka). This solution can be stored for several months at room temperature.
3.
Working solution 0.5 mM sodiumbiphosphate (NaH2PO4·2H2O).
4.
Hexa-ammonium molybdate ((NH4)6MO7O24·H2O) (Merck): 1.25% (w/v) solution. This should be prepared fresh.
5.
l-ascorbic acid (Fluka): 5% (w/v) solution. Prepare fresh.
6.
Glass test tubes (common model, 10 mL).
7.
Glass marbles.
8.
Block heater (BT5D-16, Grant, Hillsborough, NJ, USA).
9.
Water bath.
10.
Spectrophotometer (DU 800, Beckman Coulter, Fullerton, CA, USA).
2.4.3 Relaxivity Determination
1.
Paramagnetic or superparamagnetic micelles in appropriate buffer (e.g., HBS buffer, pH 7.4) with a known concentration of contrast-generating atom (see Note 4).
2.
Tabletop NMR spectrometer (Bruker Minispec MQ60 (1.41 T), Bruker, Ettlingen, Germany) and MRI scanner at which in vivo studies are performed.
2.5 In Vivo Use of Micelles
2.5.1 In Vivo Administration of Micelles in Mice
1.
Approval for the use of mice for an in vivo experiment given by the local Animal Care and Use Committee according to local legislation.
2.
Paramagnetic or superparamagnetic micelles in appropriate buffer (e.g., HBS buffer, pH 7.4) with a known concentration of contrast-generating atom (see Note 4).
3.
Needles for intravenous injection, 27 G (0.4 mm) with Luer lock (Terumo Europe N.V., Leuven, Belgium) or intravenous injection catheter, 24 G (Abbocath®-T 24 G, Hospira Inc, Lake Forest, Illinois, USA).
4.
Polyethylene catheter, inner diameter 0.4 mm (SIMS Portex, Hythe, UK).
5.
Syringe, 1 mL (Terumo Europe N.V., Leuven, Belgium).
6.
Warm water pad (T/Pump, Gaymar Industries, Orchard Park, NY, USA).
7.
Anesthesia equipment (Sigma Delta Vaporizer, Penlon Ltd., Abingdon, UK).
8.
Equipment for additional, local heat administration, e.g., gel pack, infrared light.
2.5.2 MRI Sequences for Micelle Detection
1.
Experimental animal MRI scanner.
2.
NMR/MRI acquisition software (e.g., ParaVision 5.0, Bruker Biospin GmbH, Ettlingen, Germany).
3 Methods
Anhydrous amphiphilic lipids need to be handled with care. Avoid contact with any water during the handling of the micelle constituents to prevent oxidation and hydration until the actual hydration step is carried out (Fig. 2). Lipid containers should therefore be thawed to room temperature before opening and stored at –25°C directly after use under a protective N2 gas atmosphere.
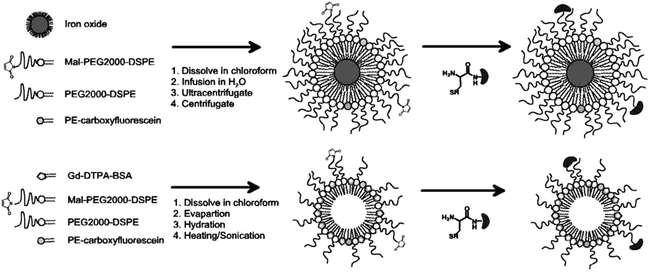
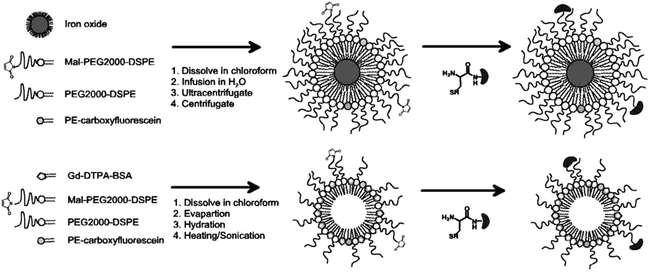
Fig. 2.
Schematic presentations of preparation steps and coupling of the ligand (dark grey kidney shaped component with reactive thiol group). The top row depicts the formation of superparamagnetic iron oxide-based micelles, while the bottom row highlights the preparation of gadolinium-containing paramagnetic micelles. The products to the right show the configuration which is formed by the amphiphlic phospholipids through self-assembly.
The formation of micelles using amphiphilic molecules is primarily driven by the hydrophobic associative interactions of the non-polar hydrocarbon chains and the repulsive interactions between the hydrophilic headgroups (12). This leads to the self-assembly of well-defined structures in water in such a way that the hydrophilic headgroups face the water while the hydrophobic parts cluster together on the inside of the micelle (see Fig. 2). Whether micelles or other structures are formed depends on the chain length of the lipids used, the number of non-polar chains per molecule, and the size of the headgroup in relation to the cross-sectional area of the hydrocarbon chains (12, 13). For micelles, a relatively large headgroup is needed (see Note 5).
Because the self-assembly itself cannot be controlled, preparative steps should be carried out with precision to gain maximum control over this process. Close attention should be paid to those details that can reveal information on the self-assembly process. This will be discussed, especially in relation to the preparation of superparamagnetic micelles where unwanted products are more readily formed in case of a poorly controlled preparation. This is because the hydrophobic character of the iron oxide core provokes aggregation of the iron oxide particles upon dispersion in water and this has to be prevented by using an excess of coating lipids, thereby maximizing the preparation of single-layered micelles containing one iron oxide particle.
The total process of preparation and functionalization typically takes one and a half days (see Fig. 3, section numbers in the text correspond with the numbers mentioned in Fig. 3). Some steps have to be carried out consecutively without delay, while other steps in the process can be paused or carried out overnight.


Fig. 3.
Timeline for preparation and functionalization of paramagnetic or superparamagnetic micelles. If ligand conjugation is needed and thus DSPE-PEG2000-maleimide is used, Sections 3.1 until 3.3.2.4 should be performed in direct consecutive order without delay. It is advised to start the phosphate determination (3.4.2) during the hydration step (3.1.14) or the ultracentrifugation steps (3.2.14). O.N., overnight.
3.1 Preparation of Paramagnetic Micelles
2.
Let the lipids warm to RT at least 15 min before opening the vials.
3.
Thoroughly clean a 250 mL round-bottom flask. Rinse sequentially three times with demineralized water, two times with ethanol, and two times with acetone. Dry thoroughly with nitrogen.
4.
Weigh the lipids and put them in the round-bottom flask.
5.
To prevent oxidation and hydration of the stock lipids, carefully blow N2 with minimal flow in the stock vials with lipids to replace air. Store lipids in freezer/dissicator.
6.
Dissolve the lipids in 5–10 mL chloroform:methanol mixture (4:1 ratio). Stir flask until all lipids are completely dissolved.
7.
Evaporate all chloroform and methanol using the rotary evaporation equipment. Set temperature of the coupled water bath to 30°C, pressure at 150 mbar (15 kPa; 2.17 psi) and the rotation speed between 100 and 140 rpm (see Note 7).
8.
After all visible solvent has been evaporated, the film must be left on the rotary evaporation equipment for an extra 15 min at 0.0 mbar.
9.
Put the flask with lipid film under N2 flow for 1 h or preferably overnight to remove any residual chloroform and methanol.
10.
Heat HBS buffer at 65°C for 10 min (see Note 2).
11.
Heat the round-bottom flask with the dried lipid film in the water bath at 65°C for 3 min in a vertical position. Prevent any contact of the lipid film with water.
12.
Hydrate the lipid film with a few milliliters of the heated HBS. The volume of the hydrating HBS buffer depends on the desired final concentration of lipids (see Note 8).
13.
Seal the round-bottom flask with Parafilm® M and connect it onto the rotary evaporation equipment, thus preventing evaporation of the hydration buffer.
14.
Heat the hydrated lipid film in the round-bottom flask at 65°C in the warm water bath, while slowly rotating between 60 and 100 rpm for 1 h (see Note 9). When the DSPE-PEG2000-maleimide will not be functionalized by ligand coupling, the paramagnetic micelles are ready and can be stored at 4°C without further preparation steps. It is important not to use the unfunctionalized micelles within 1 week after preparation to prevent the reactive maleimide group from undesired binding to other substances in vivo or even cause immunogenic responses.
15.
16.
For functionalizing of the micelles, proceed without delay with coupling of the desired ligand (see Section 3.3), as the maleimide groups are subject to hydrolysis.
Table 1
Amounts of lipids needed and their ratios for preparation of paramagnetic and superparamagnetic micelles
A | Paramagnetic micelles | Superparamagnetic micelles | ||||
Needed | Amounts | Needed | Amounts | |||
In vivo | 50–100 μmol Gd/kg BW | In vivo | 10–50 mg Fe/kg BW | |||
In vitro | 0.1–1 mM Gd | In vitro | 10–500 μM Fe | |||
Starter example | Starter example | |||||
Gd | 7.5 μmol | Fe | 2 mg | |||
Total lipids | 30 μmol | Total lipids | 15 μmol | |||
Yield | 80% | Yield | 50% | |||
End product | 6 μmol Gd | End product | 1 mg Fe | |||
B | Paramagnetic micelles | Superparamagnetic micelles | ||||
Molar ratio | MW | Molar ratio | MW | |||
Gd-DTPA-BSA | 0.50 | 1,050 | DSPE-PEG2000 | 0.89 | 2,806 | |
DSPE-PEG2000 | 0.39 | 2,806 | DSPE-PEG2000-maleimide
![]() Stay updated, free articles. Join our Telegram channel![]() Full access? Get Clinical Tree![]() ![]() ![]() |