Category
Intrafractional variations
Interfractional variations
Systematic
Random
Systematic
Random
Variation of CTV size
Physiological processes
Physiological processes
Tumor reduction or swelling
Physiological processes
Variation of CTV position
Change in treatment position
Physiological processes
Weight loss
Physiological processes
Variation in the patient position relative to the treatment beams
–
Patient movements
Technical errors
Daily set-up
4.3.2 Imaging for SBRT Planning
Imaging for treatment planning in SBRT is based on CT imaging data. Recently, for superposing on CT images, magnetic resonance imaging and positron emission tomography data have also been effectively used for SBRT planning [17]. The variation in CTV size and position depends on respiration motion and organ filling. The magnitude of variation in CTV depends on whether compensation for respiratory motion is adopted during radiotherapy delivery. Particularly, in respiratory motion, the SBRT delivery technique has an impact on the magnitude of motion in tumors and nearby normal tissues. According to an AAPM report on TG101 [18], typical SBRT margins for defining the minimal distance expanding from the CTV to PTV surfaces are 0.5 cm in the axial planes and 1.0 cm in the inferior/superior directions under respiratory depressing conditions.
The magnitude of motion should be analyzed to determine the appropriate internal margin. In general, CTV including the internal margin can be determined by the following CT imaging techniques, (1) four dimensional CT, (2) slow scan CT (e.g. 4 s for a slice), and (3) fast scan CT (multi-slices CT or dynamic scan) under conditions of inspiration and expiration breath-hold [19]. Each CT for considering respiratory motion should be evaluated from the target position between maximum inspiration and expiration, and the repeatability of the target position in the breath hold condition is critical for several CT scans. Note that attention must be paid to the characteristics of each CT acquisition method. For example, the utilization of slow scan CT images may result in under-dosing of the target volume and increased toxicity to surrounding normal tissues in 3D planning calculations [20].
Table 4.2 shows the relationship between the decision on the internal margin and X-ray CT methods for each respiratory condition.
Table 4.2
Summary of the relationship between the internal margin decision and X-ray CT methods for each respiratory condition
Respiratory motion management | Irradiation method | CT method | Internal margin | Magnitude of internal margin | Delivery time |
---|---|---|---|---|---|
Free breathing | Common | Slow scan CT or 4D-CT | Add to range of all respiratory phases in the fluoroscopic image or 4D-CT image | Large | Long |
Shallow breathing or respiratory depression | Medium | Shirt | |||
Free breathing or shallow breathing | Gating | Fast scan CT or 4D-CT under conditions of the irradiation method | Add to deviation of target position repeatability in each corresponding irradiation technique | Medium | Long |
Intercepting | Small | Long | |||
pursuing | Small | Shirt | |||
Breath-hold | Static with breath-hold | Fast scan CT under breath-hold | Add to deviation of breath hold repeatability (several CT scans are recommended) | Small | Medium |
4.4 Relationship Between Energy Selection and Beam Arrangement
When higher photon energy is used, the range of the scattered secondary electron beams becomes wider, and the convergence of the absorbed dose becomes narrower. Secondary electron equilibration is not a measurable condition with high-energy photons and deep depths in small fields. However, the mean energy of photon and electron beams increases in small fields compared with common fields. In particular, this physical phenomenon has an impact on low density regions (e.g. lung). For this reason, photon energies of 6 MV (or close to that) for tumors in the lungs should be used in SBRT [21, 22]. For tumors below the diaphragm (not passing through lung tissue or other low density tissue), photon energies of 6–20 MV are selected for SBRT delivery [23].
The current conventional linear accelerators for SBRT can deliver radiation beams shaped tightly to the target volume and all around the relevant critical structures. The beam arrangements are performed by 5–10 mm MLCs, a wedge filter (including motorized wedges), and customized blocks. Recently, the appearance of 2.5–3 mm MLCs linear accelerators has made a substantial contribution to creating conformal dose distributions in SBRT.
In general, the irradiation method for SBRT applies five to eight coplanar or non-coplanar static conformal beams. Recently, SBRT delivery has been a combination of intensity- modulated radiation techniques and volumetric-modulated arc techniques. The determination of beam orientation in SBRT is important to avoid sensitive organs, mechanical constraints, and a short beam path. In general, a large number of beams yields better target dose conformity and dose fall-off away from the target. When the number of beams is sufficiently high, however, the choice of beam direction becomes less significant [18].
In general, commercially available treatment planning systems are used to plan SBRT delivery. The radiation treatment planning system does not usually have parameters of geometric data for linear accelerators. Therefore, after completing a treatment plan for SBRT, a patient-specific QA should be conducted under the same conditions as the actual treatment conditions. The verification of non-interference between the patient and linear accelerator (e.g. gantry head) is critical, and is required for safe and effective treatment.
It should be noted that the delivery time should be shortened as much as possible. A short delivery time avoids geometric errors from intra-fractional movements. (The reason is mainly to avoid geometric errors from intra-fractional movements over longer treatment times.) Shortened delivery times can be achieved with a comprehensive delivery system including an immobilization system, localization system, on-board imaging system, and consideration of the dose rate configuration.
4.5 Radiation Delivery Equipment
In Japan, medical service fee regulations introduced reimbursement for respiratory motion management (RMM) from April 2012 [24]. The topic of RMMs is addressed in more detail in other chapters. The strategies for respiratory motion are based on the following six methods in the 2008 Guidelines for Radiotherapy Planning [24].
(i)
inhalation of oxygen
(ii)
abdominal compression
(iii)
learning regular respiratory patterns
(iv)
breath hold technique
(v)
gating with respiration
(vi)
real-time tumor-tracking
A variety of techniques to account for respiratory motion of moving targets in the thorax and abdomen is described and summarized in the AAPM report on TG 76 [19]. The six methods for respiratory motion are mentioned below based on the examples of TG 76.
(i)
Inhalation of oxygen
The inhalation of oxygen method is a shallow breathing technique and it exhibits some improvement in reduction of the respiratory rate and magnitude of breathing [25].
(ii)
Abdominal compression
The abdominal compression method is useful for respiratory motion reduction in moving tumors and nearby normal tissues and generally employs abdominal compression, an abdominal band, body shell [12, 26] and stereotactic body frames. The stereotactic body frames typically make use of vacuum cushions and an attached plate that is pressed against the abdomen. The accuracy and reproducibility of both the body frame and pressure plate have been evaluated with a comprehensive assessment report [27]. However, the usage of the body frame has some problems in term of uncertainty of patient setup, increasing respiratory displacement in the anterior-posterior direction, and physical pain.
(iii)
Learning regular respiratory patterns
This method relies heavily on the ability of the patients to perform constant respiration independently. Therefore, this mode requires attention in the stability and repeatability for the range and position of moving tumors during treatment delivery.
All methods above are motion-encompassing methods, and it is important to estimate the magnitude of motion in tumors and nearby normal tissues during CT imaging.
(iv)
Breath hold technique
Breath-hold techniques are methods of active breath-hold or passive breath-hold during a specific phase of the respiratory cycle. In general, the breath-hold technique is often used with respiratory monitoring. The reproducibility of tumor positions under self breath-hold by the patient’s own estimation after sufficient practice and in the absence of a respiratory monitoring device is satisfactorily accurate, and displacements of tumor positions are small [28]. However the reproducibility of tumor positions under self breath-hold does not show high reliability, so the monitoring of tumor position reproducibility is important when operating.
The breath hold technique with respiratory monitoring devices has been performed using active breathing control (ABC) [29], maximum breath-hold (deep-inspiration and breath hold, DIBH) [30], and patient self-judged breath hold techniques. The ABC apparatus comprises two respiratory flow monitors paired with scissor valves to control inspiratory and expiratory paths in the patient. The DIBH consists of an instruction to perform voluntary breath-hold at the end of deep inspiration to immobilize organ motion. A benefit of breath-holding during deep inspiration is the reduced density of the normal lung and minimized proportion of lung volume receiving high-dose radiation compared to total lung volume [28]. In Japan, as one patient self- breath hold method, a respiratory monitoring device that can determine the respiratory level in a patient by measuring the movement of two contacts on the abdomen and chest wall has been developed [31]. This respiratory monitoring system adopts patient self-breath-holding under constant conditions for expiration or inspiration. This device has been commercialized as Abches (APEX Medical, Inc., Japan).
The reproducibility of breath-hold at the expiration phase is more stable than the inspiration phase, but the reproducibility of breath hold position varies between individuals, so the verification of breath hold reproducibility is recommended when using fluoroscopy.
(v)
Gating with respiration
There are several respiratory gating techniques, and the typical method is gating using an external respiration signal and gating with internal fiducial markers. The Varian real-Time Position ManagementTM (RPM) system is a typical respiratory gating technique using external markers and is also used for the breath-hold method. The RPM system is a respiration-gated intermittent irradiation system, by which the movement of the external fiducial marker is placed on the patient’s anterior abdominal surface. During treatment, once a stable respiration trace has been established and gating thresholds are verified, gated radiation delivery is initiated. The control for gating respiration is that a beam-hold condition automatically occurs if the patient’s breathing level deviates form the intended level.
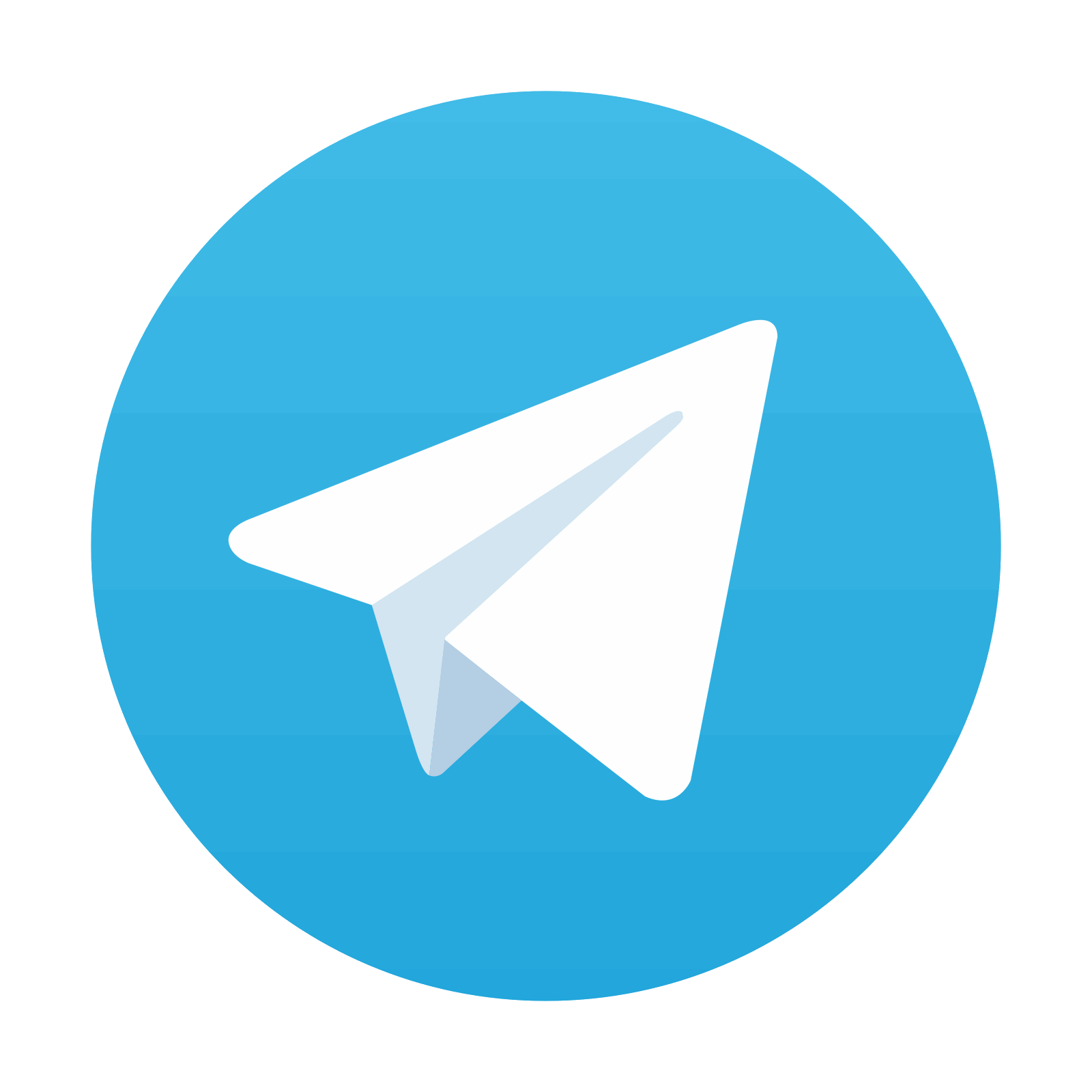
Stay updated, free articles. Join our Telegram channel

Full access? Get Clinical Tree
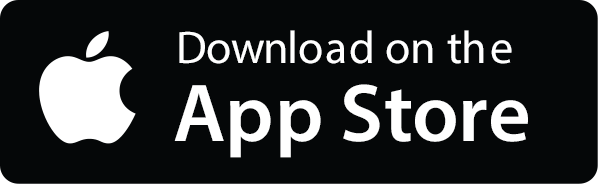
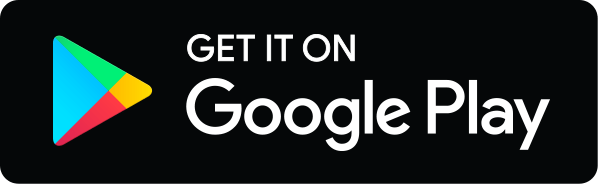