Fig. 7.1
Assessment of the left ventricle using the slice summation method (SSM). Short-axis views in end diastole (a) and end systole (b). (c) Parameters determined in assessing left ventricular function are EF ejection fraction, EDV end-diastolic volume, ESV end-systolic volume, SV stroke volume, CO cardiac output, and myocardial mass (at end diastole and average); measures of ventricular ejection and filling rates: peak ejection rate, time to peak ejection rate, peak filling rate, and time from end systole to peak filling rate
Cine sequences also permit visual assessment of ventricular contraction, detection of global or locoregional wall motion abnormalities, and evaluation of valve function (Figs. 7.2 and 7.3).
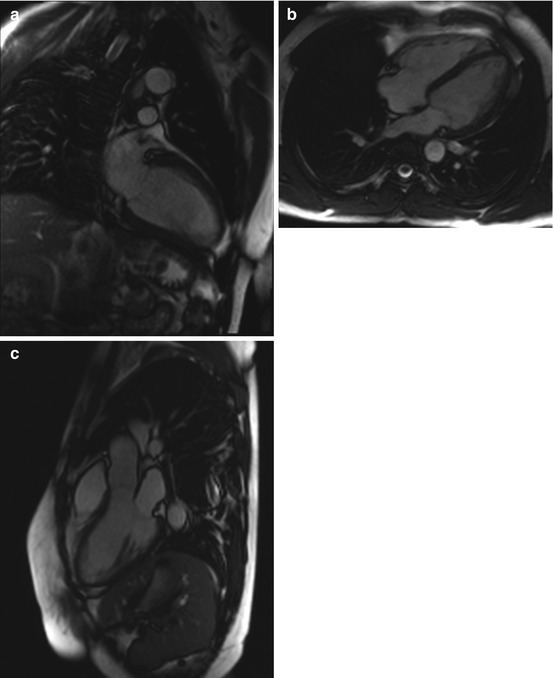
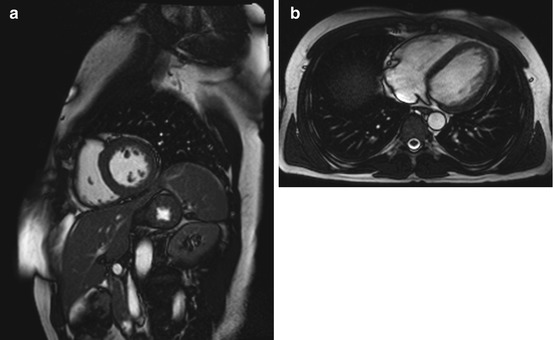
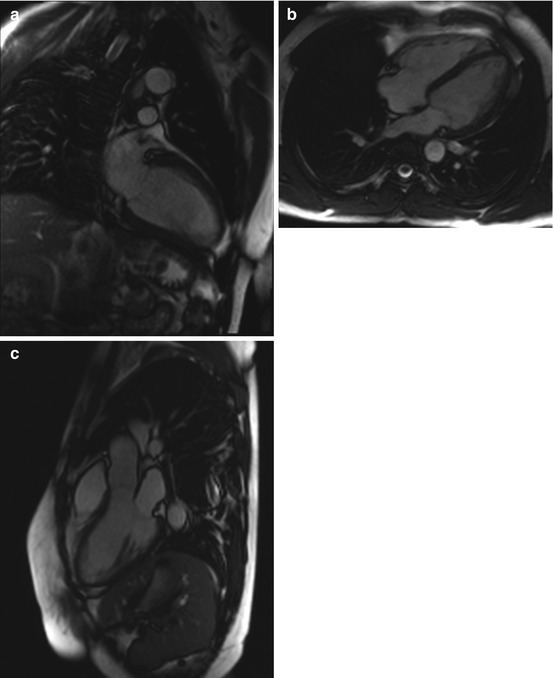
Fig. 7.2
Cine images from a healthy subject. (a) Two-chamber view, (b) four-chamber view, and (c) three-chamber view
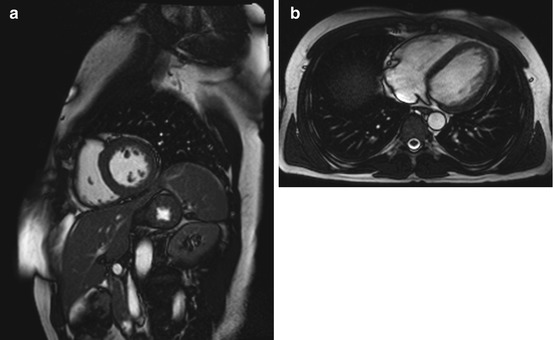
Fig. 7.3
Cine images from a healthy subject. (a) Short-axis view (midventricle) and (b) true axial view (midventricle)
Quantitative estimation of stenosis severity and valve incompetence requires the use of a flow-sensitive imaging technique. Flow across the valve is typically assessed by acquisition perpendicular to the valvular plane and is known as through-plane acquisition.
7.1.2 Edema Imaging
A clinical cardiac MRI protocol used in patients with suspected myocarditis or cardiomyopathy should always include a fat-saturated T2-weighted sequence (Carbone and Friedrich 2012). Because it suppresses signal from fat and flowing blood, this pulse sequence is highly sensitive for detecting fluids in tissues. It has approx. 80 % sensitivity in identifying myocardial edema and is also well suited for detecting concomitant pericardial effusion. Myocardial edema imaging using newer pulse sequences with much improved image quality provides useful additional diagnostic and prognostic information, allowing identification of acute or recent myocardial ischemic injury in patients with acute chest pain, distinguish acute from chronic myocardial infarction and contributing to the determination of myocardium at risk (Eitel and Friedrich 2011). Despite these advances, cardiac MRI is only a supplementary tool and not the first-line imaging modality in patients undergoing imaging for evaluation of myocarditis or cardiomyopathy.
Inflammation damages the cell membrane, resulting in a larger distribution volume of contrast medium in injured myocardium. This is one of the mechanisms underlying late enhancement in cardiac imaging.
However, the presence of late enhancement alone does not allow discrimination between inflammatory processes and scar tissue. Areas of late enhancement resulting from retention of contrast medium are seen in a variety of disorders associated with myocardial injury and edema, necrosis, or fibrosis (Manrique et al. 2009). Some additional features such as distribution and location of delayed enhancement may help the radiologist in narrowing the differential diagnosis among ischemic and nonischemic disorders. For instance, when contrast medium accumulates in the epicardium, a myocardial scar of ischemic origin is unlikely because such a scar is typically seen in a subendocardial location. Furthermore, delayed enhancement occurring in coronary artery territories can contribute to the differentiation between scar and edema. In unclear cases, acquisition of the delayed enhancement sequence can be repeated after 15–20 min. At this point in time, a scar still has high signal intensity due to delayed washout of contrast medium from injured myocardium, while edema has low signal intensity (Abdel-Aty and Schulz-Menger 2007; Aletras et al. 2008).
7.1.3 Ischemia Imaging
Imaging of ischemic myocardial injury is an important component of a cardiac MRI protocol and is done by assessing late enhancement, typically 12–15 min after administration of a Gd-DPTA-based contrast agent (e.g., gadobutrol as in the SHIP). Differential enhancement of infarcted myocardium is primarily due to diffusion and delayed clearance of contrast medium from the zone of infarction. In addition, myocardial injury shortens longitudinal relaxation (T1). Compared with other imaging methods, contrast-enhanced cardiac MRI is more sensitive in detecting even small subendocardial infarcts. Furthermore, the extent of delayed enhancement on MRI closely correlates with the size of myocardial injury determined by established methods (Kim et al. 1999; Saraste et al. 2008). In contrast, PET and SPECT usually detect large transmural infarcts or nearly transmural defects but may miss smaller, subendocardial infarcts (Wagner et al. 2003).
Cardiac MRI may also detect complications of acute myocardial infarction such as the development of left ventricular pseudoaneurysm (Jiji and Kramer 2011).
Single-shot sequences are widely used for delayed enhancement imaging, offering the advantage that all data can be acquired in a single cardiac cycle. This comes at the cost of a loss of image sharpness.
After administration of gadolinium-based contrast medium, infarcted myocardium exhibits delayed enhancement and can be identified using an inversion recovery (IR) sequence, which greatly improves contrast between viable myocardium and infarction. When magnitude reconstruction is used, IR delayed enhancement imaging is highly sensitive to the inversion time (TI) selected (Kellman et al. 2002). The optimal TI to null signal from viable myocardium (i.e., to render it dark) for an individual can be determined by obtaining a TI scout series with progressively larger TIs (TI surfing). Typical TIs are between 200 and 300 ms, depending on the amount of contrast medium administered (usually 0.1–0.2 mmol/kg body weight), time of image acquisition after contrast administration, and individual contrast medium clearance (Stork et al. 2007). An optimal TI enhances the contrast between dark viable myocardium and bright infarcted tissue. When delayed enhancement studies are performed with a phase-sensitive inversion recovery (PSIR) sequence, it is possible to use a nominal value of TI, which eliminates the need for TI surfing and achieves a consistent contrast over a wide range of TIs without artifacts due to incorrect polarity (Kellman et al. 2002).
Phase-sensitive reconstruction is used to remove the background phase while preserving the sign of the desired magnetization during IR. The phase-sensitive reconstruction method reduces the variation in apparent infarct size observed in magnitude images as TI is changed. Phase-sensitive detection also has the advantage of decreasing the sensitivity to changes in tissue T1 with increasing delay from contrast medium injection (Kellman et al. 2002) (Fig. 7.4).
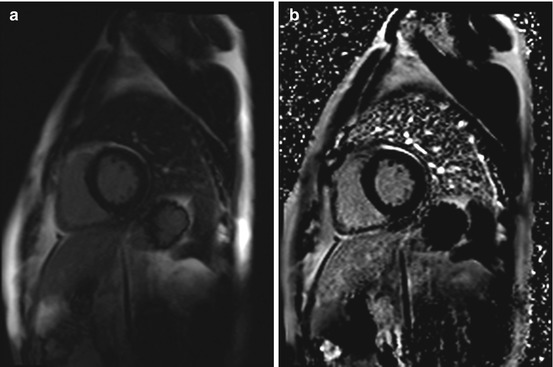
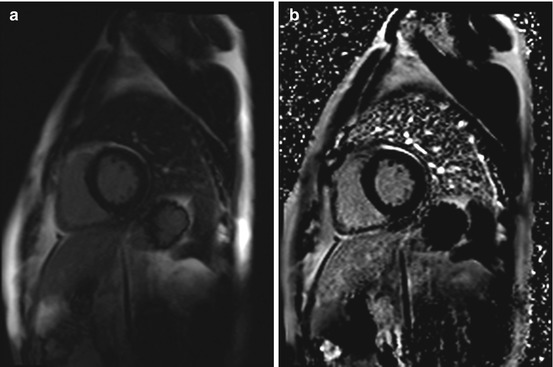
Fig. 7.4
Single-shot inversion recovery sequences (short axis) for delayed enhancement imaging in a healty subject. (a) magnitude reconstruction (b) phase-sensitive reconstruction
There are certain pitfalls one must be aware of when interpreting delayed enhancement images. Mural thrombi do not take up contrast medium and will appear dark, just like normal myocardium. Regions of microvascular obstruction after acute myocardial infarction also have low signal intensity (Jiji and Kramer 2011). Hence, it is not possible to reliably differentiate thrombus, microvascular obstruction, and viable myocardium, precluding accurate estimation of infarct size (Karamitsos et al. 2009).
7.1.4 Viability Imaging
The use of specialized pulse sequences allows myocardial perfusion imaging by assessing contrast medium inflow and clearance from the myocardium. Myocardial perfusion can be assessed at rest and stress. Pharmacologic stress is induced by administration of adenosine or dobutamine. Areas of reduced myocardial perfusion show delayed contrast medium uptake, i.e., after administration of contrast medium, they remain dark longer than well-perfused myocardium (Karamitsos et al. 2011).
Myocardial perfusion MRI allows noninvasive assessment of myocardial viability and differentiation of infarcted areas from dysfunctional but viable myocardium (Saraste et al. 2008; Wellnhofer et al. 2004). In conjunction with cine MRI and delayed enhancement imaging, cardiac perfusion MRI enables reliable detection of coronary stenosis >70 % (De Mello et al. 2012).
7.1.5 Imaging in Rare Diseases of the Heart
Cardiac MRI also has an important role in diagnosing less common diseases of the heart such as arrhythmogenic right ventricular cardiomyopathy, cardiac sarcoidosis, myocardial involvement in amyloidosis, and tumors of the heart. Moreover, it can contribute to the detection of pericardial or endocardial disease (ACCF/ACR/AHA/NASCI/SCMR 2010).
7.1.6 Cardiac Imaging in Whole-Body MRI
Due to time constraints, a cardiac MRI examination performed as part of a whole-body MRI protocol cannot include all components of a comprehensive cardiac MRI study. The cardiac MRI protocol used in the SHIP included cine sequences for functional assessment in four-chamber, three-chamber, and two-chamber views as well as short-axis views for left ventricular function assessment. Strict axial cine sequences were acquired for evaluation of right ventricular function. A fat-saturated T2-weighted pulse sequence was deliberately not included although edema may persist for some time after a myocardial event. Delayed enhancement imaging was performed using an inversion recovery single shot sequence with magnitude reconstruction, highly sensitive to the inversion time (TI) selected and a phase-sensitive inversion recovery (PSIR) single shot sequence acquired 15 min after administration of 0.15 mmol/kg body weight of gadobutrol. The PSIR sequence used in the SHIP MRI protocol creates two image data records. First dataset contains images with magnitude reconstruction, in the second dataset phase-sensitive reconstruction images are generated.
The following sections give an overview of the most common incidental findings detected by cardiac MRI performed as part of a whole-body screening examination.
7.2 Diseases of Myocardium
Areas of delayed myocardial enhancement are the most common incidental findings in the heart at screening MRI. Note, however, that delayed enhancement is a mere descriptive term and provides no clues to possible underlying causes, which are multifarious (Kim et al. 2006). Classic causes include acute and chronic myocardial infarction, cardiomyopathy, and acute or chronic myocarditis (Stork et al. 2007). In a cardiac MRI protocol, late enhancement sequences enable accurate and noninvasive quantification of the extent of myocardial infarction (gold standard). The transmural extent of delayed enhancement may be used to predict functional outcome in ischemic heart disease (Saraste et al. 2008).
7.2.1 Ischemic Heart Disease
Ischemic heart disease (IHD) or myocardial ischemia is characterized by reduced blood supply to the heart muscle and is usually due to atherosclerosis of the coronary arteries (ACCF/ACR/AHA/NASCI/SCMR 2010) (Table 7.1).
Table 7.1
Ischemic heart disease
Frequency | Common, prevalence of up to 20 %, increases with age M:F ratio of 4:1 |
Causes | Endothelial damage by atherogenic risk factors, e.g., hypercholesterinemia, hyperlipoproteinemia, smoking, diabetes mellitus, arterial hypertension, obesity, and familial predisposition |
Subsequent development of atheromatous plaque with narrowing of arterial lumen (critical when lumen loss is 70 % or greater) | |
Resulting mismatch between myocardial oxygen supply and demand | |
Clinical presentation | Angina pectoris, exercise-induced dyspnea, cardiac insufficiency, cardiac dysrhythmia, myocardial infarction in coronary artery occlusion |
Flow-limiting coronary stenosis causes coronary insufficiency, or a mismatch between oxygen supply and demand. The resulting myocardial ischemia has different clinical presentations:
Angina pectoris (stable/unstable; Canadian Cardiovascular Society (CCS) classification system)
Acute coronary syndrome (ACS)/myocardial infarction
Ischemic cardiomyopathy with cardiac insufficiency
Cardiac arrhythmia/sudden cardiac death
In patients first presenting with IHD, angina pectoris (55 %) is most common, followed by myocardial infarction (25 %) and sudden cardiac death (20 %).
IHD remains a leading cause of mortality and morbidity.
Cardiac MRI is highly accurate and has robust prognostic value in the evaluation of patients with both acute and chronic IHD (Heydari and Kwong 2014).
MRI Features
Lines of myocardial calcification or subendocardial fatty metaplasia.
Myocardial thinning with akinesia, hypokinesia, and dyskinesia.
Akinesia is common in areas of transmural infarction and manifests as absence of systolic thickening and contraction in affected wall segments.
Hypokinesia is reduced systolic thickening and contraction of myocardium.
Dyskinesia is paradoxical motion, often involving predominantly the septal segments of the left ventricle (septal dyskinesia).
Myocardial aneurysm or pseudoaneurysm can develop.
Subendocardial to transmural delayed enhancement, usually without an increase in T2 signal intensity, which can be localized to the area supplied by a particular coronary artery (Stork et al. 2007). In acute infarction, concomitant edema of affected wall segments may be detectable on fat-saturated T2-weighted images (Karamitsos et al. 2011).
The severity of wall motion abnormality varies with the transmural extent of infarction. Wall motion disturbance and myocardial thinning are usually more marked when MRI shows full-thickness late enhancement compared with subendocardial enhancement (Fig. 7.5).
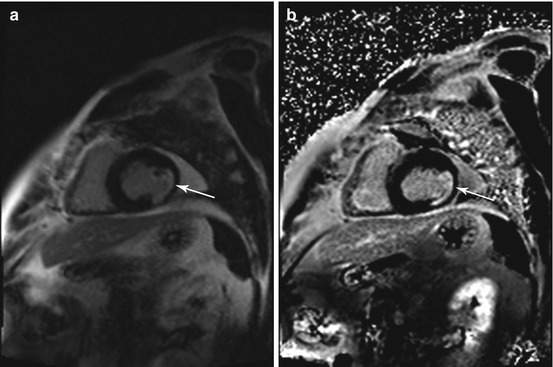
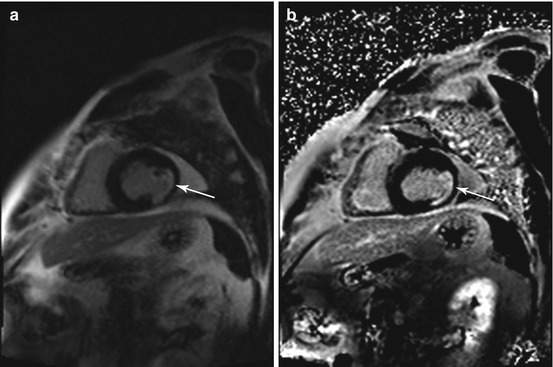
Fig. 7.5
Incidental findings in a 57-year-old subject. Typical subendocardial delayed enhancement in the lateral walls of the left ventricle (arrow). Late enhancement imaging with single shot inversion recovery sequences (short axis) (a) phase sensitive reconstruction (b) magnitude reconstruction
Myocardial infarction involving the papillary muscles can impair mitral valve closure. Short-axis views are well suited to localize infarcted myocardial segments to specific coronary artery territories.
Among coronary dominance patterns, so-called balanced circulation is the most common type (80 %). In balanced circulation, the left coronary artery supplies the anterior wall of the left ventricle and most of the interventricular septum. The right coronary artery supplies the right ventricle and the posterior wall segments. The left coronary artery divides into the left anterior descending artery (LAD), or anterior interventricular branch, and the circumflex branch (LCX) (Cerqueira et al. 2002) (Fig. 7.6).
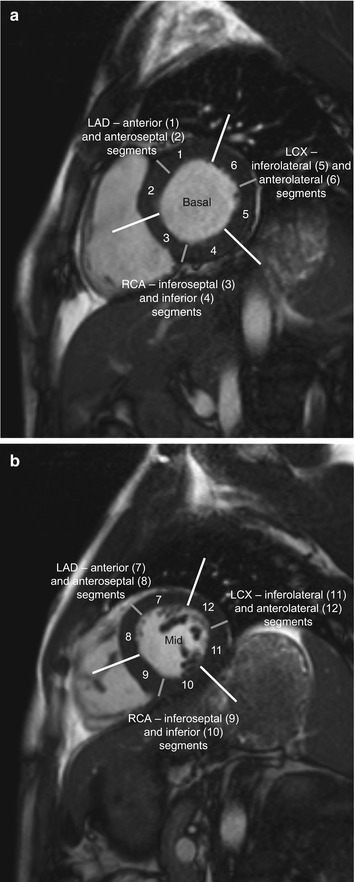
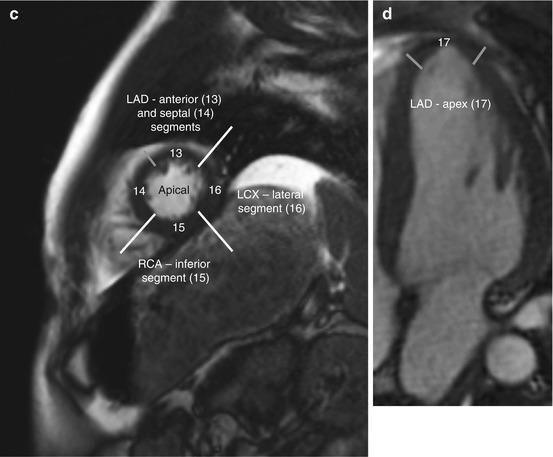
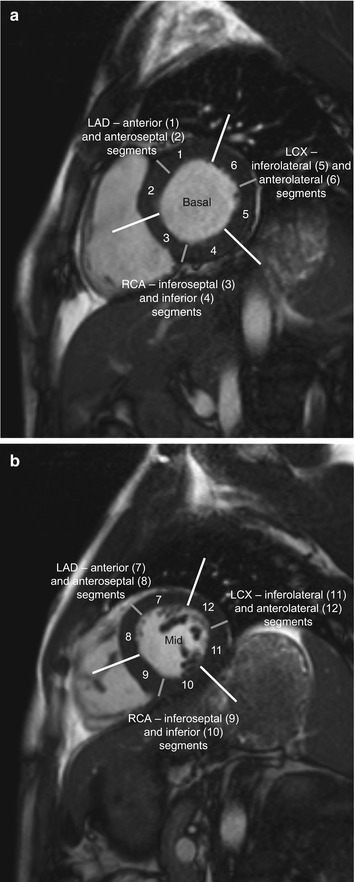
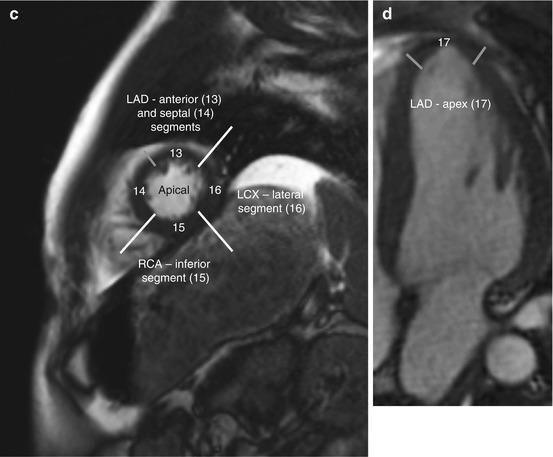
Fig. 7.6
Myocardial segmentation and assignment of the 17 myocardial segments to coronary artery territories. Short-axis views at basal level (a), at midventricular level (b), and at apical level (c) and apical long-axis view (d) (LAD left anterior descending artery, LCX left circumflex branch, RCA right coronary artery) (According to Cerqueira et al. (2002))
Differential Diagnosis
Delayed enhancement of other cause (Stork et al. 2007)
Acute myocardial infarction (edema on fat-saturated T2-weighted sequences, myocardial thickening, fatty metaplasia, calcification, or aneurysm detectable) (Perazzolo et al. 2011)
Acute myocarditis (see below; edema on fat-saturated T2-weighted sequences)
Nonischemic cardiomyopathy (see below; nonischemic myocardial fibrosis or scar has a variety of delayed enhancement patterns but is never subendocardial, making it easy to differentiate from ischemic infarction) (Stork et al. 2007)
In most patients, a variety of further cardiac and myocardial changes can be observed secondary to a myocardial infarction. Ventricular function may be impaired by left ventricular remodeling. Left ventricular dilatation is common and can evolve into full-blown dilated cardiomyopathy (De Smet et al. 2012). Initially the Frank-Starling mechanism maintains a constant left ventricular ejection fraction by responding to an increase in volume with an increase in wall tension. At some point, however, the bulge becomes so large that a marked drop in LVEF results. Mitral regurgitation due to remodeling of the chordal fibers of the papillary muscles is another common sequela.
Finally, pseudoaneurysm may develop, typically involving the posterior and lateral walls, less commonly the anterior wall.
Clinical Management
A previous small subendocardial or transmural myocardial infarction with well-preserved pump function requires no further clinical diagnostic evaluation. A larger subendocardial scar is critical even when pump function is preserved. It should be disclosed if a subject has another significant pathologic finding elsewhere in the body that may require surgery. The same holds true for transmural late enhancement with wall motion abnormalities. Markedly impaired pump function due to myocardial scar formation, which may be associated with concomitant pericardial or pleural effusion, should always be revealed to the subject. In this case, electrocardiography, ergometry, and echocardiography are recommended as supplementary diagnostic tests. Depending on the findings, cardiac catheterization may be the next step.
7.2.2 Cardiomyopathies
According to a consensus of the WHO and the International Society and Federation of Cardiology Task Force (1995), cardiomyopathies are diseases of the myocardium associated with cardiac dysfunction. Five main types are distinguished: dilated cardiomyopathy (DCM), hypertrophic cardiomyopathy (HCM and HOCM), restrictive cardiomyopathy (RCM), arrhythmogenic right ventricular cardiomyopathy (ARVC), and unclassified cardiomyopathies, such as noncompaction cardiomyopathy and Takotsubo cardiomyopathy. They are primarily not based on valvular, pericardial, or cardiovascular disorders (ACCF/ACR/AHA/NASCI/SCMR 2010) (Tables 7.2 and 7.8).
Table 7.2
WHO classification of cardiomyopathies
Type | Abbreviation | Characteristics |
---|---|---|
Dilated cardiomyopathy | DCM | Systolic dysfunction with reduced ejection fraction |
Hypertrophic cardiomyopathy, subtype: hypertrophic obstructive cardiomyopathy | HCM, subtype: HOCM | Thickening of ventricular walls prevents adequate left ventricular expansion during diastole, impairing diastolic compliance |
Restrictive cardiomyopathy | RCM | Increased wall stiffness due to endocardial fibrosis, impairing diastolic compliance |
Arrhythmogenic right ventricular cardiomyopathy | ARVC | Right ventricular diastolic and systolic dysfunction and concomitant ventricular tachycardia as well as localized aneurysms of the right ventricle |
Unclassified cardiomyopathies | UCCM | For example, noncompaction cardiomyopathy, Takotsubo cardiomyopathy |
Table 7.8
MRI features of the different cardiomyopathies
Parameter | DCM | HCM/HOCM | RCM | ARVC | NCCM |
---|---|---|---|---|---|
Atrial size | Typically both LA and RA are enlarged | Typically both LA and RA are enlarged | Typically both LA and RA are enlarged | LA of normal size, RA occasionally enlarged | LA and RA of normal size |
Ventricular size | Enlargement of both LV and RV or of LV alone | LV and RV of normal size, rarely reduced in size | LV and RV of normal size | RV enlarged, LV usually of normal size | LV and RV typically of normal size, LV enlarged in rare cases |
LV mass | LV normal or slightly hypertrophied; LV and RV mass normal or increased | Generalized hypertrophy of LV; septal hypertrophy, primarily apical, in 25 % of cases | LV and RV of normal size | RV wall involved at apex and inflow/outflow tract Wall thinning giving rise to microaneurysms Prominent trabeculation (stack of dishes) and fat deposition in RV wall | LV wall normal or thickened at apex and/or midventricle |
LVEF | LVEF reduced | LVEF usually normal; may be increased in HOCM due to obstruction | LVEF normal | LVEF normal RVEF reduced | LVEF normal or reduced |
Delayed enhancement | Atypical delayed enhancement (in 10–40 % of cases): intramural, subepicardial | Diffuse or confluent delayed enhancement; often patchy enhancement of septum in HOCM | Absent | Diffuse patchy delayed enhancement of RV (often difficult to evaluate) | Absent |
Diseases of the heart muscle associated with cardiac or systemic disorders are referred to as specific cardiomyopathies in the WHO classification. They comprise ischemic, valvular, hypertensive, inflammatory, and metabolic cardiomyopathies, general system diseases, muscular dystrophies, neuromuscular disorders, sensitivity and toxic reactions, and peripartum cardiomyopathy. Dilated cardiomyopathy is the most common (Richardson et al. 1996) (Tables 7.2 and 7.3).
Table 7.3
Dilated cardiomyopathy
Frequency | 6/100,000 population/year |
Causes | Idiopathic |
Genetic (familial) | |
Primary and secondary causes | |
Postinfectious (viral, bacterial, fungal, parasitic), toxic (alcohol, medications), autoimmune (vasculitis, systemic lupus erythematosus), endocrine (thyroid dysfunction, pheochromocytoma), neuromuscular and metabolic, infiltrative (amyloidosis), inflammatory (sarcoidosis) | |
End stage of long-standing arterial hypertension, ischemia based on coronary artery disease, and progressive valvular dysfunction | |
Clinical presentation | Progressive left ventricular failure with exercise-induced dyspnea; global insufficiency and ventricular arrhythmia in advanced disease |
New scientific insights led to a revision of the definition and classification of cardiomyopathies in 2006. According to this revised definition, the cardiomyopathies are a heterogeneous group of diseases of the myocardium associated with mechanical and/or electrical dysfunction that usually exhibit inappropriate ventricular hypertrophy or dilatation and are due to a variety of causes that frequently are genetic. Cardiomyopathies either are confined to the heart or are part of generalized systemic disorders, often leading to cardiovascular death or progressive heart failure-related disability (Maron et al. 2006).
The revised classification distinguishes two major groups based on predominant organ involvement: primary cardiomyopathies, in which the clinically relevant disease processes solely or predominantly involve the myocardium, and secondary cardiomyopathies, which show pathologic myocardial involvement as part of a large number and variety of generalized systemic (multiorgan) disorders.
Primary cardiomyopathies are further subdivided by etiology: genetic (hypertrophic cardiomyopathy, hypertrophic obstructive cardiomyopathy, arrhythmogenic right ventricular cardiomyopathy, and noncompaction cardiomyopathy), mixed (dilated cardiomyopathy and restrictive cardiomyopathy), and acquired (inflammatory cardiomyopathy based on myocarditis, Takotsubo cardiomyopathy). Secondary cardiomyopathies appear in the context of various diseases: infiltrative (amyloidosis), inflammatory (sarcoidosis), storage (hemochromatosis), endomyocardial (endomyocardial fibrosis, hypereosinophilic syndrome (Löffler’s endocarditis)), endocrine (diabetes mellitus, hyperthyroidism, pheochromocytoma), and neuromuscular diseases; nutritional deficiencies; autoimmune disorders (vasculitis, systemic lupus erythematosus); and exposure to toxic substances (cancer therapy) or drugs (Maron et al. 2006).
Dilated cardiomyopathies, which may be genetic or acquired, are the most common type. Causes of acquired dilated cardiomyopathy include a history of myocarditis or alcohol abuse. In addition, dilated cardiomyopathy may develop as the terminal stage of a variety of preexisting cardiac diseases, such as coronary heart disease or arterial hypertension (Richardson et al. 1996) (Tables 7.2 and 7.3).
Cardiac MRI has an important role in the diagnostic and clinical management of patients with cardiomyopathies, allowing evaluation of myocardial morphology and function in a single examination and hence also enabling identification of less common underlying causes (De Smet et al. 2012). The five main types of cardiomyopathy distinguished in the WHO classification are presented in more detail in the following sections.
7.2.2.1 Dilated Cardiomyopathy
Dilated cardiomyopathy (DCM) is characterized by left ventricular dilation and systolic dysfunction with a left ventricular ejection fraction of less than 40 % with or without concomitant right ventricular involvement (ACCF/ACR/AHA/NASCI/SCMR 2010) (Fig. 7.7).
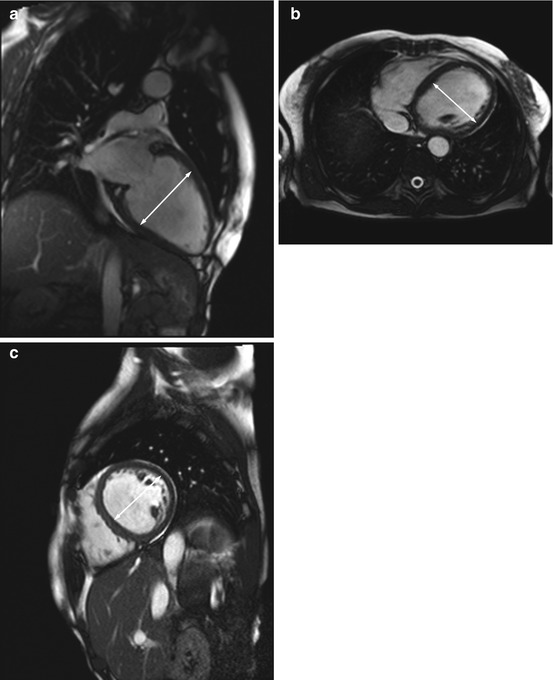
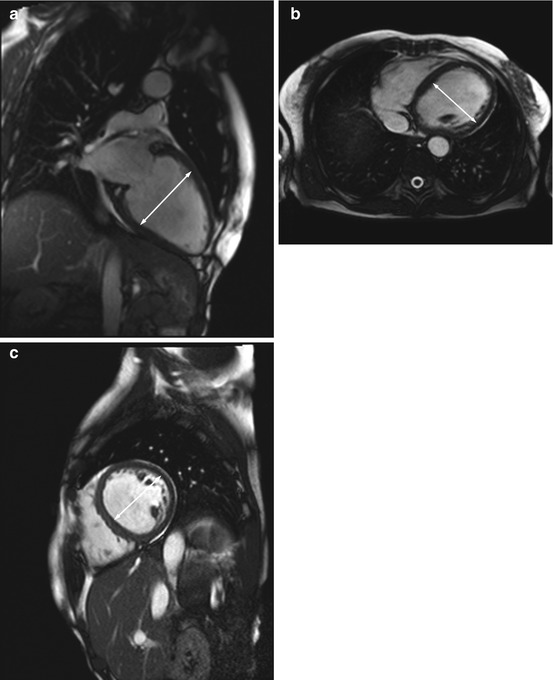
Fig. 7.7
DCM in a 66-year-old subject with reduced pump function (approx. 45 % EF) and global hypokinesia in all wall segments. Left ventricular enlargement (arrow indicates left ventricular diameter). (a) Two-chamber cine view, (b) true axial cine view, and (c) short-axis cine view
The severity of left ventricular dysfunction determines the patient’s prognosis.
DCM is probably the result of myocardial damage due to a variety of primary and secondary underlying causes: infectious agents; viral or chronic myocarditis; other bacterial, fungal, and parasitic infections; toxic myocardial damage (alcohol, cocaine); chemotherapy (primarily doxorubicin); metabolic defects (e.g., hyperphosphatemia, hypocalcemia, uremia); myocardial involvement in other tissue disorders (vasculitis, e.g., Churg-Strauss syndrome, systemic lupus erythematosus); endocrine disorders (thyroid dysfunction, pheochromocytoma, Cushing disease); infiltrative or inflammatory diseases such as amyloidosis or sarcoidosis; and neuromuscular diseases. In rare cases, DCM is associated with pregnancy (peripartum cardiomyopathy). DCM is also the end stage of other myocardial conditions, such as long-standing arterial hypertension, ischemia based on coronary artery disease, or progressive valvular dysfunction. Approx. 20–35 % of DCM cases are assumed to be familial. In many cases, no etiology is apparent (idiopathic DCM) (Table 7.3) (De Smet et al. 2012; Maron et al. 2006).
DCM is characterized by progressive heart failure and a decline in left ventricular contractile function, ventricular and supraventricular arrhythmias, conduction system abnormalities, thromboembolism, and sudden or heart failure-related death (Maron et al. 2006). Patients with symptomatic DCM have a mortality rate of 11–13 %.
Cardiac MRI provides very accurate estimates of left and right ventricular volumes, atrial dimensions, myocardial mass, and left ventricular ejection fraction, and it also offers a quick and reliable means of detecting areas of fibrotic transformation (Schalla et al. 2010). The prognosis of idiopathic DCM depends on the severity of left ventricular dysfunction, with delayed enhancement being an additional prognostic factor. Areas of delayed enhancement indicate more extensive fibrotic transformation of myocardium, which is usually associated with lower LVEF and an increase in end-diastolic volume (Lehrke et al. 2011).
However, the estimates should be verified by echocardiography to confirm an initial MRI-based diagnosis of DCM.
MRI Features
Enlargement of the left ventricle without an increase in mass; globally reduced systolic inward motion and wall thickening as well as markedly reduced LVEF (De Smet et al. 2012).
Markedly reduced pump function and enlarged volume lead to circular movement of blood with an increased risk of thrombus formation.
Ventricular dilation additionally causes regurgitation through the mitral valve and possibly through the tricuspid valve as well.
This can result in enlargement of both atria.
Depending on the severity of DCM, end-diastolic and end-systolic volumes (EDV and ESV) may be increased.
In idiopathic DCM, first-pass perfusion usually appears normal.
Concomitant enlargement of the right ventricle indicates advanced DCM.
Thirty to forty percent of patients with idiopathic cardiomyopathy show midwall enhancement from the base to the midventricle (De Smet et al. 2012) (Fig. 7.8); the typical pattern of late enhancement is in the form of longitudinal striae not corresponding to a coronary artery territory (Schalla et al. 2010; Stork et al. 2007).
As DCM can also develop secondary to ischemic heart disease, marked subendocardial or transmural delayed enhancement may be seen in conjunction with left ventricular enlargement (McCrohon et al. 2003).
An occasional patient may show no delayed enhancement at all (Jiji and Kramer 2011).
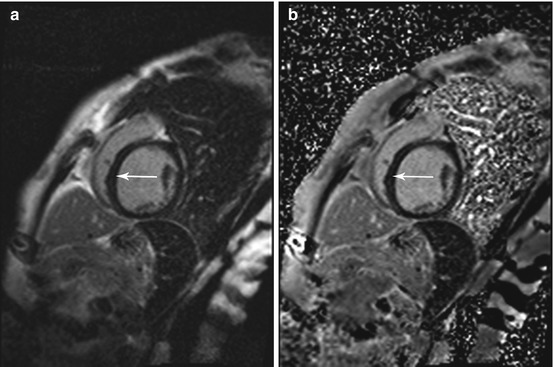
Fig. 7.8
Phase-sensitive inversion recovery (PSIR) single shot sequence for delayed enhancement imaging in the same subject as in Fig. 7.7. Typical midwall enhancement predominantly involving the septum (arrow). (a) Magnitude reconstruction and (b) phase-sensitive reconstruction
Differential Diagnosis
Restrictive cardiomyopathy (see below; typically, isolated enlargement of both atria with normal ventricular size and diastolic dysfunction) (Mookadam et al. 2011)
Hypertrophic or hypertrophic obstructive cardiomyopathy (see below; no dilatation but left ventricular wall thickening, predominantly of the basal interventricular septum) (De Smet et al. 2012)
Valvular diseases (predominantly chronic reflux through the aortic or mitral valve, resulting in increased volume load and reduced systolic function) (Bonow et al. 2008)
Clinical Management
When dilated cardiomyopathy (DCM) with markedly reduced pump function is suspected, the subject should be informed and undergo further diagnostic workup. The ventricular size measured by MRI should be confirmed by echocardiography as a second imaging modality and vice versa. In addition, most patients subsequently require myocardial biopsy.
7.2.2.2 Hypertrophic and Hypertrophic Obstructive Cardiomyopathy
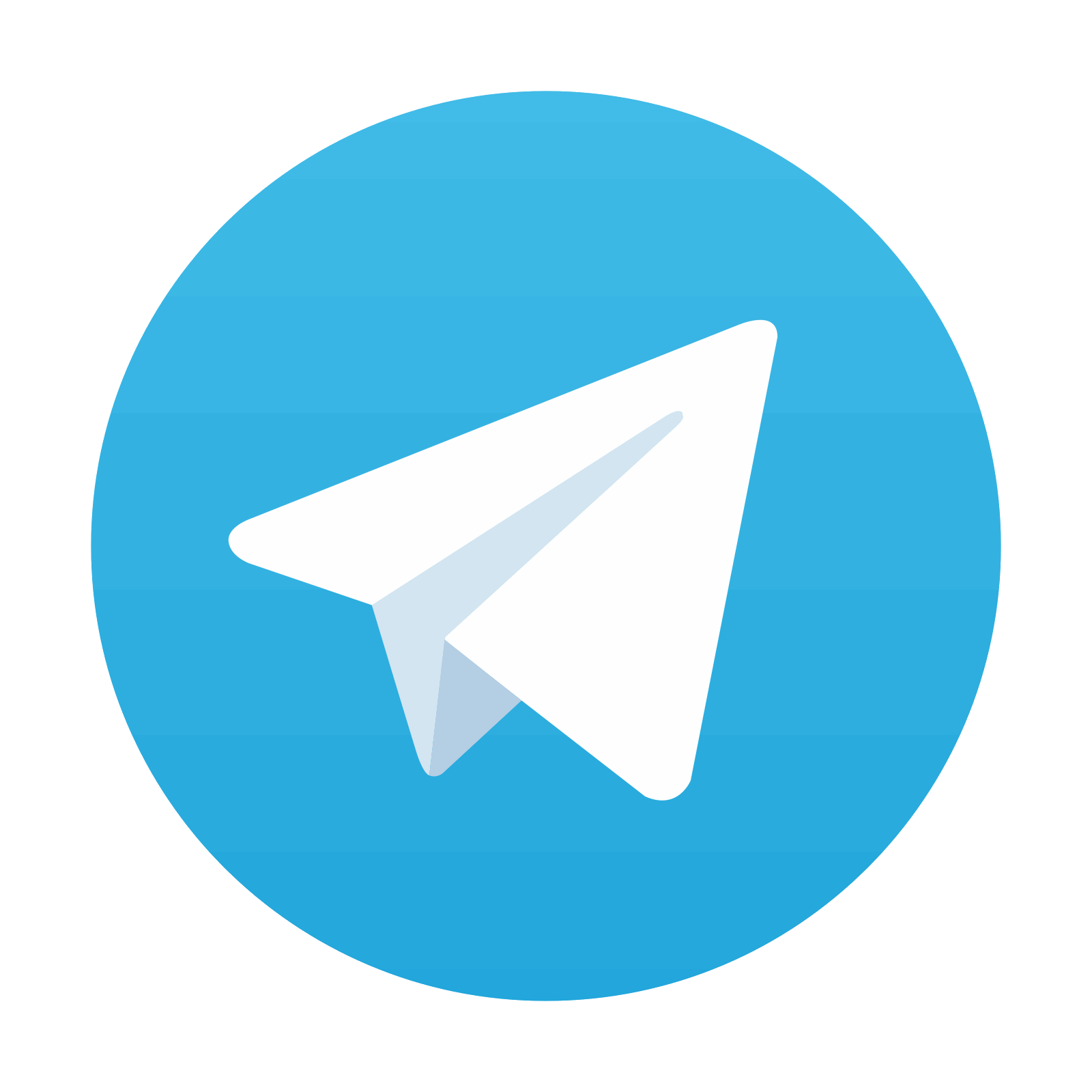
Stay updated, free articles. Join our Telegram channel

Full access? Get Clinical Tree
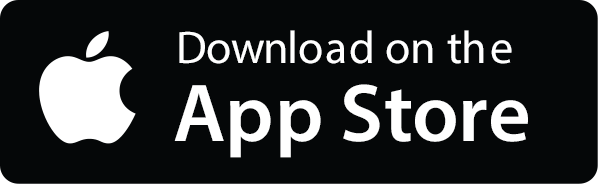
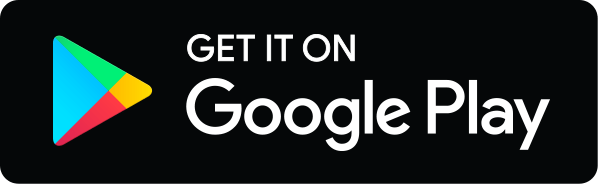