Ahmed Aljizeeri1,2,3 and Mouaz H. Al‐Mallah4 1 King Abdulaziz Cardiac Center, Riyadh, Saudi Arabia 2 King Saud bin Abdulaziz University for Health Sciences, Riyadh, Saudi Arabia 3 King Abdullah International Medical Research Center, Riyadh, Saudi Arabia 4 Houston Methodist DeBakey Heart & Vascular Center, Houston Methodist Hospital, Houston, TX, USA The global health and economic burdens of cardiovascular diseases are immense. Cardiovascular diseases are one of the top healthcare challenges of the new millennium, with similar morbidity and mortality in industrial and developing countries. There is a global call for a comprehensive plan to control the pandemic of cardiovascular diseases through prevention and early detection. In addition, precise diagnosis and accurate prognosis to reduce complications are of paramount importance in the management of cardiovascular diseases. Non‐invasive cardiac imaging appears to hold the promise of all the properties needed to control the pandemic of cardiovascular diseases. This chapter summarizes the role of nuclear cardiology in the initial evaluation, diagnosis, and prognosis of cardiovascular diseases. Ischemic heart disease (IHD) remains the leading cause of mortality and morbidity all over the world despite the significant reduction of mortality rates in high‐income countries [1, 2]. IHD, albeit chronic, represents a dynamic process, with presentation ranging from acute life‐threatening conditions known as acute coronary syndromes to less life‐threatening chronic conditions known as stable IHD. In recent years, stable IHD became the most frequently encountered clinical presentation of IHD [3]. Management of patients with IHD starts with the evaluation of patients with new‐onset chest pain suspected to have IHD and extends to establishing the prognosis and management plan for those with known IHD. Nuclear cardiology remains the cornerstone of the management of patients with IHD. Exercise stress electrocardiogram is the most commonly used test for the initial evaluation of patients with suspected IHD. It is considered positive when it demonstrates at least 1 mm horizontal or down‐sloping ST‐segment depression 80 milliseconds after the J point on the electrocardiogram (ECG) [4]. The diagnostic accuracy of the exercise stress test is limited by many confounders, such as left ventricular hypertrophy, right bundle branch block, resting ST‐segment abnormalities, and medications that affect the baseline ECG [5, 6]. In general, the sensitivity and specificity of the exercise stress test are 68% and 77%, respectively [7] and these values are lower in women compared to men [8–11]. To improve diagnostic accuracy, the exercise stress test can be combined with myocardial perfusion imaging (MPI). There is an enormous body of evidence supporting the role of MPI in the diagnosis and management of the whole spectrum of IHD presentation. The most widely used and validated exercise stress test protocol is the Bruce protocol [12, 13]. It consists of multiple 3‐minute stages based on achieved estimated metabolic equivalent (MET). The patient blood pressure and ECG are monitored during the test. The radiotracer is injected when the patient achieves the target heart rate. The target heart rate is calculated as 85% of the maximum age‐predicted heart rate. The maximum age‐predicted heart rate is conventionally calculated as 220 – age (in years) [14]. The stress images are then obtained 10–15 minutes after the termination of the stress test and compared to rest images [15]. A delay of 45–60 minutes after injection of the radiotracers is allowed before obtaining the rest images [15]. Although exercise is the preferred form of stress testing because since it is more physiologic, most of the patients with IHD cannot exercise due to age and comorbidities, therefore the pharmacological stress test is used in IHD patients who cannot exercise or in whom the exercise stress test is contraindicated. There are two types of the pharmacological stress test: dobutamine and vasodilator. Dobutamine is a synthetic catecholamine that increases oxygen demand through the heart rate and blood pressure. Dobutamine is given as a continuous intravenous infusion with graded doses to achieve the target heart rate. Atropine is usually given if the maximum dobutamine dose of 40 μg/kg/min is reached without achieving the target heart rate. The radiotracer is administered when the target heart rate is achieved [15]. The vasodilator stress test is the most used pharmacological stress tests in contemporary cardiology practice. Three vasodilators are currently in clinical use, adenosine, regadenoson, and dipyridamole (Table 8.1). These agents act by increasing the myocardial blood flow through the vasodilatation of the coronary arteries [16]. Ischemia is demonstrated when there is a relative difference in the blood distribution between areas supplied by arteries with and without stenosis [17]. The contraindications and when to stop the exercise and pharmacological stress tests are summarized in Table 8.2. There are multiple imaging protocols for MPI with single‐photon emission tomography (SPECT) (Figure 8.1). In the one‐day protocol both the rest and stress images are obtained on the same day with enough time allowed for adequate decay of the first injection of the radiotracer. When using this protocol, rest images are obtained first and then stress images are obtained with a higher dose of radiation [15]. The other protocol is a 2‐day protocol in which the stress and rest images are obtained in 2 days [18, 19]. This protocol is used in patients with a large body habitus to decrease the radiation dose since the same dose of radiotracer is administered for rest and stress [15]. A stress‐only protocol is also used. In this protocol, only stress images are obtained without rest images if the stress images are normal. MPI with positron emission tomography (PET) is usually done in a 1‐day protocol due to the short half‐life of PET radiotracers [20]. SPECT MPI uses three radiotracers: thallium‐201 (201Tl) and two technetium‐99m (99mTc) tracers, sestamibi and tetrofosmin. In addition, iodine‐123 meta‐iodobenzylguanidine (123I‐MIBG) is used for risk stratification of patients with heart failure. The higher energy and shorter half‐life of 99mTc‐based radiotracers allow for imaging with a lower radiation dose [21–23]. In addition, 99mTc‐based radiotracers are produced from a generator while thallium is cyclotron produced, therefore technetium‐based radiotracers are preferred over 201Tl [24]. The most widely used radiotracers for PET MPI in a clinical setting are rubidium‐82 and ammonia‐13. While rubidium‐82 is generator produced, ammonia‐13 is produced from a cyclotron. Both these radiotracers are used for MPI and quantification of myocardial blood flow [25]. Furthermore, 18‐fluorodeoxyglucose (18FDG) is used for metabolic imaging in the evaluation of myocardial viability and cases of inflammation and infections [26, 27]. The characteristics of SPECT and PET radiotracers are summarized in Table 8.3. Table 8.1 Characteristics of the vasodilator in the pharmacological stress test. Table 8.2 Contraindications and indications for early termination of exercise and pharmacological stress tests. AV, atrioventricular; BP, blood pressure; LBBB, left bundle branch block; MI, myocardial infarction. Figure 8.1 Common protocols in SPCET imaging. Table 8.3 Characteristics of the commonly used cardiac SPECT and PET tracers. FDA, US Food and Drug Administration; 18‐FDG, 18‐fluorodeoxyglucose; MPI, myocardial perfusion imaging. The initial evaluation of patients with suspected IHD includes confirming that the presenting symptoms are due to IHD, considering the need for further evaluation with invasive coronary angiography and, subsequently the need for revascularization. The fundamental goal of the management of those with known IHD is to guide medical and revascularization therapy, predict future cardiac events such as cardiac death and nonfatal myocardial infarction (MI), and find measures to prevent them. In addition, MPI is one of the most trusted methods of evaluating the physiological significance of known anatomical stenosis. With more than 30 years of experience, nuclear cardiology MPI is arguably the most evidence‐based noninvasive modality in the management of patients with stable IHD. MPI has been evaluated across the full spectrum of IHD presentations with rigorous prospective and retrospective studies. Multiple studies have demonstrated the high diagnostic accuracy of SPECT and PET MPI in the detection of significant coronary artery disease (CAD). In a large meta‐analysis of 108 SPECT studies and four PET studies involving 11 862 patients, the pooled sensitivities were 92.6% and 88.3% for PET and SPECT, respectively [28], while the pooled specificities were 81.3% and 75.8%, respectively [29]. Another meta‐analysis of eight SPECT and 15 PET studies demonstrated sensitivities of 85% and 90% for SPECT and PET, respectively, while the specificities were 85% and 88%, respectively, when compared to invasive coronary angiography [30]. When compared to invasive fraction flow reserve (FFR), a head‐to‐head comparison in 208 patients demonstrated sensitivities of 57% and 87% for SPECT and PET, respectively, while the specificities were 94% and 84%, respectively [31]. A meta‐analysis of 37 studies examining the diagnostic accuracy of multiple noninvasive stress tests against invasive FFR showed specificities of 61% and 83%, respectively, and specificities of 84% and 89%, respectively [32] (Figures 8.2 and 8.3). In general, PET has better diagnostic accuracy than SPECT owing to its better spatial resolution and the use of attenuation correction, which results in better image quality and a lower rate of artifact [33] (Figure 8.4). Another advantage of PET over SPECT is the ability of PET to diagnose balanced ischemia that can be easily overlooked in relative perfusion imaging. The ability of PET to measure myocardial blood flow allows for the diagnosis of balanced ischemia due to multivessel disease as well as coronary microcirculatory dysfunction [34]. Coronary flow reserve (CFR), which is calculated as the ratio between stress and rest myocardial blood flow, can also be used to exclude significant left main disease [20, 28]. A CFR >2 reliably excludes high‐risk angiographic stenoses such as left main disease or two‐vessel disease, including proximal left anterior descending artery with a negative predictive value (NPV) of 97% [35] (Figure 8.4). Coronary microvascular dysfunction (CMD) poses a diagnostic challenge and appears to be more common than initially thought. Almost 60% of patients with angina do not have obstructive CAD on invasive coronary angiography [36]. The diagnosis of CMD on PET MPI is made when there is a reduced CFR (<2) with normal relative perfusion images [37]. PET also allows for evaluation of left ventricular ejection fraction (LVEF) reserve, which is calculated as LVEF at peak hyperemia – LVEF at rest. A reserve of more than +5% excludes left main/three‐vessel disease with an NPV of 97%, while a reserve of −5% has a positive predictive value (PPV) of 77% for the diagnosis of left main/three‐vessel disease [38]. Figure 8.2 63‐year old male with diabetes, hypertension, and hypercholesterolemia, referred for PET MPI for evaluation of chest pain. The perfusion images show severe reversible perfusion defect on the mid and apical segments of the anterior wall and apex (arrows). The coronary angiography revealed severe disease in the mid segment of the anterior descending coronary artery. Figure 8.3 58‐year‐old male with diabetes and hypertension referred for evaluation of exertional chest pain. PET MPI demonstrates normal perfusion with high transient ischemic dilatation (1.26) and normal coronary flow reserve (2.3). In view of normal perfusion and normal CFR, she was referred to coronary angiography, which revealed nonobstructive coronary artery disease. The real strength of MPI is its ability to evaluate risk among patients with IHD even after revascularization. With more than 30 years of follow‐up, MPI is arguably the most rigorous tool for risk stratification in patients with known and suspected IHD (Table 8.4). It is worth mentioning that exercise and pharmacological stress tests have similar prognostic capabilities, regardless of the stressor. A large meta‐analysis of 14 918 patients who underwent stress MPI demonstrated similar prognostic value between exercise and pharmacological stress tests [39]. However, it is well known that the inability to exercise portends a poor prognosis, which was demonstrated in the same meta‐analysis. The risk of cardiac death and nonfatal MI was <1% in patients with normal exercise MPI compared to 1.78% in normal pharmacological MPI [39]. Similarly, the rate of these events is much higher in abnormal pharmacological MPI compared to abnormal exercise MPI (4.3% vs. 9.9%). In general, an abnormal study is associated with a five‐ to sixfold increase in the risk of cardiac death and nonfatal MI [39], while normal MPI is associated with a less than 1% risk of cardiac death and nonfatal MI [40]. Furthermore, normal MPI is associated with favorable outcomes regardless of the Duke treadmill score [41]. The burden of ischemia can also risk‐stratify patients with suspected and known IHD. The higher the ischemic burden, the higher the risk of cardiac death [42, 43]. Mildly abnormal MPI is associated with a more than twofold increase in the risk of cardiac death while severely abnormal MPI is associated with an almost fivefold increase in cardiac death [42, 44]. Figure 8.4 54‐year‐old male with hypertension referred for evaluation of chest pain. SPECT MI done with and without attenuation correction. The noncorrected images show a stress perfusion defect, which was resolved with attenuation correction. Table 8.4 Prognostic variables in cardiac MPI. CFR, coronary flow reserve; HLR, heart/lung ratio; LV, left ventricular; LVEF, left ventricular ejection fraction; MPI, myocardial perfusion imaging; SPECT, single‐photon emission tomography; TID, transient ischemic dilatation. Multiple nonperfusion variables are found to have prognostic value. Left ventricular size and have been shown to have prognostic value. Patients with LVEF <45% and left ventricular (LV) end‐systolic volume of >70 mL have lower survival regardless of ischemic burden [45]. Stress‐induced wall motion abnormalities are associated with increased risk of cardiac death and nonfatal MI regardless of perfusion findings [46]. In addition, transient ischemic dilatation (TID) (increase in post‐stress LV size compared to rest) of >1.2 is associated with increased risk of significant CAD and poor outcome [46, 47]. However, recent analysis has questioned the diagnostic and prognostic value of TID in patients with normal perfusion [48]. Increased lung/heart ratio (>0.5 for thallium and >0.32 for technetium) is associated with higher risk of cardiac death [49, 50]. This is thought to be due to severe CAD leading to diastolic dysfunction. LV dyssynchrony by phase analysis was also found to predict major adverse clinical events including cardiac death and nonfatal MI independent of LVEF [51]. Finally, vasodilator‐induced ST‐segment change is associated with a worse outcome even in the presence of normal MPI [52, 53]. The unique ability of PET to measure myocardial blood flow and the subsequent calculation of CFR provides an independent and incremental prognostic value in patients referred to PET MPI. Multiple studies have demonstrated the prognostic value of CFR irrespective of the presence and extent of ischemia. PET‐derived CFR of <2 is associated with increased risk of adverse outcome, including cardiac death, in multiple studies with various event rates [54–56]. A CFR of <2 is associated with a more than threefold increase in cardiac death while CFR <1.5 is associated with a more than fivefold increase in cardiac death [56]. The CFR was also able to further risk stratify patients within mild, moderate, and severe ischemia [54]. Furthermore, CFR was able to reclassify more than 50% with intermediate risk to either low‐ or high‐risk categories [56]. In a large study of more than 12 000 patients, CFR <1.8 was associated with a significant risk of cardiac and all‐cause mortality regardless of ischemia [57]. Moreover, MPI can be used to guide decisions about revascularization in patients with IHD. The initial data suggest that a benefit can be derived from revascularization if the ischemic burden is more than 10% of the total LV myocardium in patients without known IHD [58]. However, in patients with IHD and no prior MI, revascularization was associated with a better outcome when the ischemic burden was 10–15% [59]. Early revascularization was also found to be beneficial in patients with CFR < 1.8 irrespective of the burden of ischemia [57]. However, these observations are derived from retrospective observational studies, which may limit rigor. The International Study of Comparative Health Effectiveness with Medical and Invasive Approaches (ISCHEMIA) trial is a prospective study with more than 5000 patients comparing early revascularization and optimal medical therapy in patients with stable IHD and moderate to severe ischemia. The study showed that 3.3 years of follow‐up failed to demonstrate a benefit of routine invasive therapy for the primary outcome of cardiovascular death, MI, resuscitated cardiac arrest, or hospitalization for unstable angina or heart failure [60, 61]. Nuclear cardiology guide therapy and can also be used to predict the outcome of evaluation of myocardial viability. Chronic coronary insufficiency leads to reversible myocardial dysfunction, causing a presentation of heart failure with reduced ejection fraction. The condition of reversible dysfunction is called myocardial hibernation to differentiate it from irreversible dysfunction due to myocardial necrosis [62, 63]. Assessment of viability and hibernating myocardium is an essential step for the decision about revascularization for improvement of LV systolic function. SPECT MPI with thallium‐201 was one of the earliest assessment methods of myocardial viability. Assessment of viability with 201Tl depends on the redistribution property of the radiotracer [64]. Images are usually obtained 4 hours and then 24 hours after injection of thallium since about 20% of segments with perfusion defect at 4 hours show evidence of redistribution at 24 hours [65]. Segments that show >50% of the peak thallium uptake are considered viable. 99mTc was shown to have good a relationship with the histological viability of explanted hearts [66]. 201Tl MPI sensitivity and specificity to predict recovery after revascularization are 87% and 54%, respectively [26, 27, 67]. Metabolic imaging with PET is considered one of the established standards of assessment of myocardial viability [68, 69]. Hibernating myocytes shift metabolism to depend on glucose rather than fatty acid for energy. 18FDG is a glucose analogue used in PET imaging to evaluate myocardial metabolism. 18FDG is given with glucose load and intravenous insulin to enhance uptake [70]. The 18FDG images are then compared to rest perfusion images to assess viability and scar (Table 8.5). A territory is considered viable if it has normal perfusion and metabolism (match) and considered hibernating if it has abnormal perfusion but normal metabolism (mismatch). A transmural infarct is diagnosed where there is a severe (<50% of the peak uptake) matched defect, while nontransmural infarct is diagnosed where there is a matched defect with >50% of the peak uptake [68]. Multiple studies have demonstrated the high diagnostic accuracy of PET in the evaluation of myocardial viability. In a meta‐analysis of 24 studies and 756 patients, the sensitivity and specificity of PET in predicting functional recovery were 92% and 63%, respectively [67]. An uptake of <50% of the peak uptake has NPVs of 87% and 92% for functional recovery in rest ammonia‐13 and 18FDG, respectively [71] (Figure 8.5). The prognostic value of PET viability is well documented. PET perfusion/metabolism mismatch ≥18% was associated with the best improvement in functional capacity after revascularization [72]. Moreover, patients with reduced LV ejection fraction and viability had significant mortality survival (80% reduction) with revascularization [69]. The long‐term follow‐up of the PARR‐2 trial showed better event‐free survival in patients who adhere to PET recommendation of revascularization compared to the standard care [73]. Furthermore, it was shown that delaying revascularization in patients with viability detected on PET is associated with an increased risk of perioperative death and failure to improve LV ejection fraction [74]. PET MPI has an increasing role in the management of patients post cardiac transplantation. PET‐derived CFR can predict all‐cause mortality, acute coronary syndrome, and hospitalization for heart failure [75]. It was also found that impaired CFR is associated with allograft vasculopathy and 2.8‐fold higher risk of death [76, 77]. Table 8.5 Interpretation of viability based on 18FDG PET imaging. Figure 8.5 60‐year old female with diabetes, hypercholesterolemia, and moderate chronic kidney disease referred for viability evaluation following a new diagnosis of heart failure with an ejection fraction of 30% and anterior wall akinesia. Viability images demonstrate a mismatch pattern with reduced rubidium‐82 perfusion (yellow arrows) and normal 18FDG uptake (white arrows) in the anterior wall, indicating hibernation of the anterior wall. Elderly patients, women, and diabetic patients with IHD represent special populations with added management challenges. Elderly patients are more likely to have comorbidities and significant coronary stenosis [78]. In addition, age is a strong predictor of ischemia in patients undergoing MPI for the evaluation of chest pain. However, the prognostic value of normal MPI and the ischemic burden remains valid in this population [79, 80]. It is documented that normal MPI has a similar risk of low annual event rates in the elderly [80]. Although women are known to have a lower burden of anatomical stenosis than men, they have a higher rate of cardiovascular events [81]. The exercise stress test has a higher rate of false results in women compared to men [8, 82]. However, MPI remains a robust diagnostic tool in women with and without known IHD [83]. MPI also has a robust prognostic role in women. Women with normal MPI have 99% event‐free survival [84]. Furthermore, the ischemic burden has been shown to better risk‐stratify women than men in addition to predicting outcomes [85]. PET‐derived CFR provides incremental diagnostic value in women with suspected IHD due to its ability to diagnose CMD, a disease common in women [86, 87]. It is interesting to know that women with impaired CFR are associated with a lower risk of multivessel disease compared to men [87]. It is also known that impaired CFR with normal MPI, a common finding in women, is associated with worse outcomes [88]. Finally, diabetic patients represent a high‐risk population with a high prevalence of silent ischemia [89]. Diabetic women with high‐risk abnormal scans have twice the risk of adverse events compared to men [90]. In a study of 2783 patients, diabetic patients with low CFR and no known CAD had a similar rate of cardiac death [91]. Therefore, PET CFR is a promising tool in the risk stratification of diabetic patients with and without IHD and with and without renal failure [44]. It is worth mentioning that ethnicity may play a role in the prognosis of IHD. In a large study of more than 7000 patients, abnormal SPECT MPI was associated with a higher risk of mortality in African‐American and Hispanics compared to Caucasians (14% vs. 8% vs. 2.7%, respectively) [92]. The utility of coronary artery calcium (CAC) scoring has been the subject of multiple cohort studies in the past two decades [93, 94]. Multiple large single‐ and multicenter studies have confirmed its superiority to traditional risk factors for the prediction of incident cardiovascular events [95, 96]. It can be performed with low radiation dose exposure and high reproducibility (Figure 8.6). In addition, it adds incremental prognostic value [97–99], especially when combined with perfusion imaging [100]. In recent years, cardiac computed tomography angiography (CCTA) has come to the forefront of the anatomical evaluation of coronary arteries. Over the past decade, CCTA has accumulated a large body of evidence to support its value in the initial evaluation of patients suspected to have CAD [101] (Figure 8.7). Multiple multicenter studies have demonstrated the high accuracy of CCTA in the diagnosis of significant CAD (>50% stenosis) [102, 103]. These studies have persistently demonstrated high sensitivity of 95–98% and a very high NPV of 97–99%. Unfortunately, this diagnostic utility of CCTA is limited by lower specificity of 64–90% and PPV of 64–91%. The high diagnostic accuracy of CCTA extends to patients with coronary revascularization with percutaneous intervention and coronary artery bypass surgery (CABG). A pooled data of 843 CABG patients showed sensitivity, specificity, PPV, and NPV of 98%, 97%, 92%, and 99%, respectively [104]. Likewise, a meta‐analysis of 1300 patients with coronary stents showed sensitivity, specificity, PPV, and NPV of 90%, 92%, 72%, and 97%, respectively [105]. The bigger the size of the stent the more likely it can be evaluated by CCTA [106–108]. However, the real challenge facing CCTA is its modest PPV in the detection of significant stenosis. CCTA‐derived fractional flow reserve (CT‐FFR) has emerged in recent years as a noninvasive tool in the evaluation of the functional significance of coronary stenoses. CT‐FFR employs fluid dynamics to estimate the ratio of the flow through a stenosed artery compared to the flow when there is no stenosis. Multiple studies have demonstrated the diagnostic accuracy of CT‐FFR against invasive FFR with significant improvement of the AUC [109–111], but is limited in accuracy in the intermediate range [112] (Figure 8.8). In one study, there was at least 40% improvement of the diagnostic accuracy compared to CCTA [110]. CT MPI is a promising tool in the identification of significant anatomic stenosis [32], but its use is limited by the inherent high radiation [113]. Figure 8.6 High three‐vessel calcium score in a patient with normal perfusion PET (with diaphragmatic attenuation artifact). Figure 8.7 CCTA of the left anterior descending coronary artery (LAD) of three different patients: (a) normal LAD, (b) mild noncalcified plaque (yellow arrow), and (c) calcified nonobstructive plaque (yellow arrow) and mixed plaque resulting in occlusion of the mid LAD (white arrow). The CONFIRM registry, which is a large multicenter registry of CCTA, has demonstrated the ability of CCTA to predict adverse outcomes in various patient populations [114–117]. The registry showed an increased risk of MACE (all‐cause mortality, nonfatal MI, unstable angina or late revascularization) significantly increased in patients with nonobstructive CAD compared to those without CAD (13.24% vs. 5.6%) [118]. In real‐world practice, a cohort of nearly 17 000 patients demonstrated the prognostic value of CCTA in predicting adverse outcome, including all‐cause death, MI, and late revascularization [119, 120]. The high special resolution of CCTA allows for the identification of vulnerable plaques [121], which are plaques with a high likelihood to rupture causing acute coronary syndrome [122–124]. High‐risk features indicative of vulnerable plaque include soft low attenuation, spotty calcification, and positive remodeling [125, 126]. The Coronary Artery Disease Reporting and Data System (CAD‐RADS) score is recommended for the standardization of CCTA reporting. The CAD‐RADS score assigns higher scores to coronary stenoses based on their severity [127]. Higher CAD‐RADS scores are associated with an increased risk of MACE [128]. In the large SCOT‐HEART study, the addition of CCTA to the standard care of patients presenting with chest pain resulted in a lower rate of cardiac death and nonfatal MI at 5 years [129]. The prognostic value of CT‐FFR was also proven in the prediction of future MACE [130, 131]. The data from the ADVANCE registry, 5083 patients, showed that normal CT‐FFR was associated with a lower risk of cardiac death and nonfatal MI [132]. Figure 8.8 FFR CT of the left anterior descending coronary artery of a patient with severe lesion showing hemodynamically significant stenosis. The role of cardiac magnetic resonance (CMR) imaging is ever‐increasing, particularly with the emerging data from large registries and multicenter trials. CMR can provide a wide range of information in patients with known and suspected IHD from diagnosis through guiding therapy to prognosis. It allows for the accurate evaluation of many diagnostic and prognostic parameters without the risk of ionizing radiation [133, 134]. Three earlier prospective randomized trials (IMPACT‐I, IMPACT‐II, and CE‐ MARC) demonstrated the high diagnostic accuracy of stress CMR in the evaluation of patients with suspected IHD [135–137]. Stress CMR has sensitivity, specificity, PPV, and NPV of 86.5%, 83.4%, 77.2%, and 90.5%, respectively [136]. CE‐MARC further consolidated the role of stress CMR in the management of stable IHD. Stress CMR was superior to guideline‐directed care in reducing the rate of unnecessary invasive angiography with no difference in the rate of MACE [138].
8
The Role of Noninvasive Cardiac Imaging in the Management of Diseases of the Cardiovascular System
Introduction
Ischemic Heart Disease
The Role of Nuclear Cardiology in the Management of IHD
Myocardial Perfusion Imaging Radiotracers and Protocols
Exercise Stress Test
Pharmacological Stress Test
Vasodilator
Adenosine
Regadenoson
Dipyridamole
Dose
140 mg/kg/min
0.4 mg
0.56 mg/kg
Mechanism of action
Non‐selective activation of adenosine receptors
Activation of adenosine A2 receptors
Phosphodiesterase enzyme inhibitor, inhibition of the cellular reuptake of adenosine
Duration of infusion
4–6 minutes
10 seconds
4 minutes
Maximum hyperemia
3 minutes of infusion
10–20 seconds after the infusion
3 minutes after completion of infusion
Half‐life
2–10 seconds
2–3 minutes
40 minutes
Elimination
Cellular uptake
Renal
Biliary
antidote
Stop the medication
Aminophylline
Aminophylline
Common side effect
Flushing, chest pain, and bronchoconstriction
Shortness of breath, headache, and flushing
Chest pain, headache, and dizziness
Exercise stress test
Dobutamine
Vasodilator
Contraindications
When to stop
Cardiac SPECT tracers
201 Tl
99m Tc‐sestamibi
99m Tc‐tetrofosmin
Photon energy (keV)
67
140
140
Physical half‐life (hours)
73
6
6
Uptake mechanism
Active transport
Passive diffusion
Passive diffusion
Preparation
Cyclotron
Generator
Generator
Heart uptake (%)
3
1–1.4
1.2
Elimination
Renal
Hepatic
Hepatic
Redistribution
Significant
Minimal
Minimal
Stress modality
Exercise or pharmacologica
Exercise or pharmacological
Exercise or pharmacological
Cardiac PET tracers
Ammonia‐13
Rubidium‐82
Flurpiridaz‐18
18‐FDG
Positron energy (MeV)
0.49
1.48
0.25
0.25
Physical half‐life
10 minutes
76 seconds
110 minutes
110 minutes
Positron range (mm)
2.5
8.6
1
1
Uptake mechanism
Diffusion and metabolic trapping
Active transport
Mitochondrial binding
Glucose transport
Preparation
On‐site cyclotron
Generator
Regional cyclotron
Regional cyclotron
Myocardial extraction fraction (%)
80
65
94
3
Indication
Perfusion MPI
Perfusion MPI
Perfusion MPI
Viability
Stress modality
Pharmacological
Pharmacological
Exercise or pharmacological
Clinical status
FDA approved
FDA approved
FDA approved
Phase III clinical trails
Nuclear Cardiology and the Diagnosis of IHD
Nuclear Cardiology and the Prognosis of IHD
Clinical variables
Hemodynamic variables
Electrocardiographic variables
Perfusion imaging variables
Nonimaging variables
Exercise‐induced anginal
Exercise capacity
Maximum ST depression
Large area of ischemia
Rest LCEF < 40%
Exercise‐limiting anginal
Exercise duration
Number of leads with ST depression
Multiple areas of ischemia
Stress LVEF < 45%
Maximum exercise heart rate
Time of onset of ST depression
Ischemia burden >10%
End‐systolic LV volume > 70 mL
Maximum exercise systolic blood pressure
Exercise‐induced ventricular arrhythmia
TID > 1.2
LVEF reserve <−5%
Chronotropic incompetence
Vasodilator‐induced ST depression
Lung uptake on SPECT
(HLR > 0.5 for thallium and >0.32 for technetium)
CFR < 1.5
Calcium score >400
Rest perfusion
18FDG imaging
Wall motion
Interpretation
Recovery
Normal
Normal
Normal
Normal
High
Reduced
Normal
Reduced
Hibernation
Yes
Reduced
Partially reduced
Reduced
Nontransmural
infarct
Possible
Reduced
Severely reduced
Reduced
Transmural infarct
Unlikely
Prognostic Role of Nuclear Cardiology in Special Patient Populations
The Role of Coronary Calcium Scoring in the Diagnosis and Prognosis of IHD
The Role of CCTA in the Diagnosis and Prognosis of IHD
The Role of CMR Imaging in the Diagnosis and Prognosis of IHD
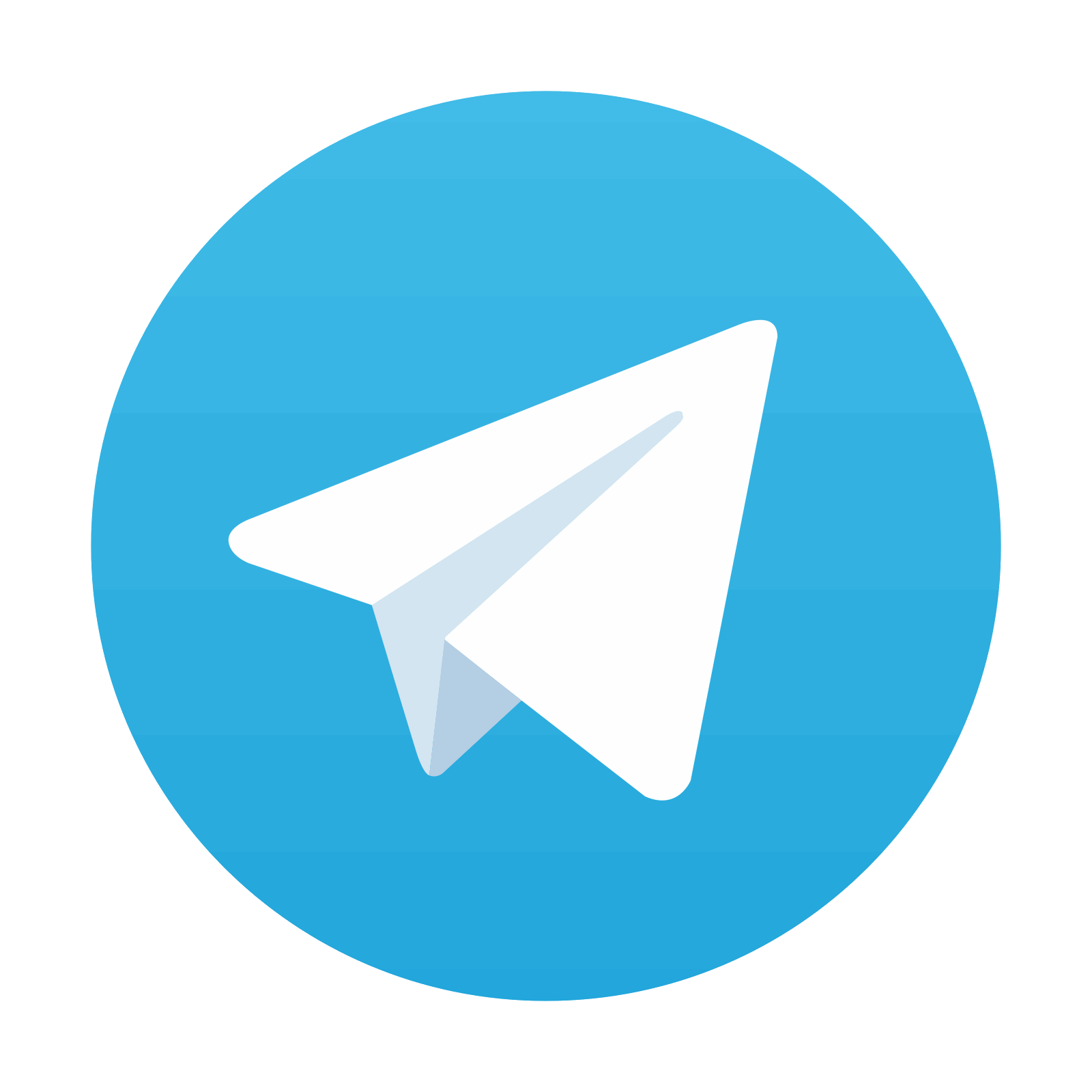
Stay updated, free articles. Join our Telegram channel

Full access? Get Clinical Tree
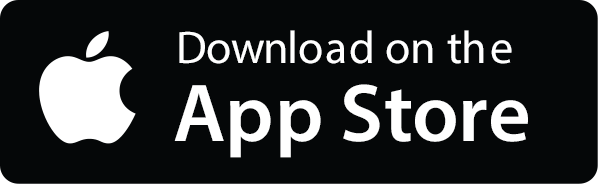
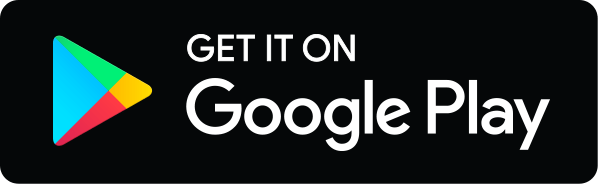