FIGURE 17.1-1: Embryologic development of the respiratory diverticulum, lung buds, trachea, and bronchi. A: Formation of the respiratory diverticulum from the ventral surface of the embryologic foregut, from which it is eventually separated by two tracheoesophageal ridges. B: Elongation of the respiratory diverticulum with subsequent formation of the lung buds. C–E: Development of the trachea and lungs at 5 weeks (C), 6 weeks (D), and 8 weeks (E). (Adapted from Sadler TW. Langman’s Medical Embryology. 12th ed. Philadelphia, PA: Lippincott Williams & Wilkins; 2012.)
The respiratory diverticulum elongates to form the trachea and two lung buds, each of which enlarges to form the main bronchi at the 5th week of gestation. Branching morphogenesis is driven by signals received in the epithelium of the lung bud from the surrounding mesenchyme. The lung buds divide successively in dichotomous fashion, resulting in 10 right segmental bronchi and 8 left by the end of 6 weeks’ gestation, the precursors of the bronchopulmonary segments in the adult lung (Fig. 17.1-1C–E). By 16 weeks after additional rounds of branching, the basic conducting architecture of the lung has been established.
The development of the pulmonary vasculature closely mirrors that of the bronchial tree. After their appearance at approximately 5 weeks’ gestation, the sixth branchial arches develop into the main pulmonary artery and its right and left branches.1 The preacinar pulmonary arteries and veins form by vasculogenesis using the budding airways as a scaffold through the 17th week of gestation. Postacinar pulmonary vessels subsequently form by angiogenesis in a network closely associated with the growing alveoli.6 The bronchial arteries develop later than the pulmonary arteries, arising during the 8th week of gestation from the dorsal aorta and entering the lungs alongside the main bronchi.
The peripheral airways enlarge during the canalicular period, and the capillaries in the surrounding mesenchyme undergo massive proliferation and become closely apposed to the airway epithelium.6 Type II epithelial cells are derived from the cuboidal epithelium of the bronchial tree and begin to produce surfactant at approximately 22 to 24 weeks. The acinar epithelium also differentiates into type I epithelial cells, which contribute to formation of the first functional gas-exchange surfaces.5 During the subsequent saccular phase, an increase in the number of terminal sacs (primitive alveoli) results in a rapid expansion of the gas-exchanging surface area. By 26 to 28 weeks’ gestation, there is sufficient vascularization of the lungs, surfactant, and terminal sacs to potentially allow survival of a prematurely born infant who receives intensive neonatal care.
Alveolarization commences at 36 weeks’ gestation; however, the vast majority of alveoli develop after birth.2 This process continues until late childhood, approximately 8 years of age.1 Postnatal growth in the lung is due primarily to an increase in the number of respiratory bronchioles and alveoli as opposed to an increase in alveolar size.
Factors Affecting Lung Growth
The most important factors needed for normal lung development and growth include amniotic and lung fluid and adequate thoracic space. The formation and clearance of fetal lung fluid is a dynamic process. Lung liquid is formed by active transport across the pulmonary epithelium into the bronchial system, establishing positive transpulmonary pressure. Clearance of this fluid is achieved by fetal breathing movements7 and by peristaltic airway contractions.8 Approximately 50% of lung fluid is swallowed by the fetus, the principal mechanism of amniotic fluid resorption, with the other 50% effluxed through the trachea into the amniotic space, comprising 30% of the amniotic fluid.9 The efflux of lung liquid and intratracheal pressures are carefully regulated by the larynx.
Inadequate amniotic fluid is well documented to be associated with pulmonary hypoplasia, although the exact mechanism remains unclear. Proposed mechanisms include physical compression of the thorax by the uterine wall, inhibition of fetal breathing movements, and increased efflux of lung liquid into the amniotic space.1 Limited intrathoracic space also has a detrimental effect on lung growth and can result in pulmonary hypoplasia, as seen in the case of congenital diaphragmatic hernia (CDH), pulmonary masses, and skeletal malformations. Fetal breathing movements, which appear as early as 11 weeks’ gestation, produce changes in intrathoracic pressure, and there is experimental evidence that these movements also contribute to normal lung development.10,11
A balance between lung volume and pressure is required for appropriate growth as adequate transpulmonary pressure maintains lung expansion. Lung liquid not only distends the developing airways but also stimulates proliferation of alveolar cells. Disruption of this balance in either direction has significant consequences on lung development, as seen in the opposing examples of pulmonary hypoplasia and congenital high airway obstruction syndrome (CHAOS).
Increased knowledge of fetal lung fluid dynamics has resulted in the development of fetoscopic tracheal occlusion for treatment of CDH and the use of amnioinfusion for pulmonary hypoplasia related to oligohydramnios.
PULMONARY HYPOPLASIA
Pulmonary hypoplasia can be clinically defined as incomplete development of the pulmonary parenchyma in the presence of a normal tracheobronchial tree. Pulmonary agenesis is a complete failure of airway and parenchymal development, and pulmonary aplasia is defined by incomplete development of lung parenchyma with a rudimentary bronchus. The formal histologic diagnosis of pulmonary hypoplasia is determined by postmortem measurements, including lung weight-to-body weight ratio, radial alveolar count, and DNA estimation,12 and therefore limited for clinical use. Ultrasound (US) and MRI, however, are noninvasive tools that can aid in the antenatal prediction of and differentiation between lethal and nonlethal forms of pulmonary hypoplasia.
Incidence: Pulmonary hypoplasia is relatively common with a reported incidence of 0.9 to 1.1 per 1,000 live births and 1.4 per 1,000 of all births.13,14 The prevalence of this condition in autopsies ranges between 4.9% and 22%.13,15,16
Pathogenesis/Etiology: Bilateral pulmonary hypoplasia may be the result of various congenital abnormalities or complications of pregnancy that inhibit lung development (Table 17.1-1). Pulmonary hypoplasia most commonly occurs in the setting of oligohydramnios due to preterm premature rupture of membranes (PPROM) or fetal renal abnormalities, reportedly occurring with oligohydramnios for as short as 6 days.12 Intrathoracic masses, including CDH and congenital pulmonary airway malformations (CPAM), also cause pulmonary hypoplasia. Other potential etiologies include skeletal malformations deforming the thoracic cavity, pleural effusions, neuromuscular anomalies, abdominal wall defects, and cardiac lesions. Complete pulmonary agenesis may be due to the failure of the development of bronchial buds or an in utero vascular accident.
Numerous syndromes are associated with primary abnormalities in lung development, including trisomies 13, 18, and 21. Other syndromes associated with pulmonary hypoplasia include fetal akinesia deformation sequence (FADS), cerebrooculofacioskeletal (COFS) syndrome due to hypotonia and decreased fetal breathing, and various skeletal dysplasias that cause restriction of thoracic volume. Primary isolated pulmonary hypoplasia in the absence of an underlying disorder is extremely uncommon, typically sporadic, but may have rare familial occurrence.17
Unilateral pulmonary hypoplasia is typically secondary to conditions that limit the thoracic space available for lung growth and is rarely due to a primary embryologic defect.18 Less common etiologies for congenital unilateral pulmonary hypoplasia include main pulmonary artery atresia or thrombus, lobar agenesis–aplasia complex (including pulmonary venolobar or scimitar syndrome), and accessory diaphragm.18–20
Diagnosis: In the setting of pulmonary hypoplasia, a reduction in pulmonary lung volumes will be observed on both US and MRI. A bell-shaped chest is common. Decreased T2 parenchymal signal intensity for gestational age is typically seen on MRI.21,22 Both US and MR may show ancillary findings that may explain the presence of pulmonary hypoplasia (Fig. 17.1-2). In unilateral pulmonary agenesis or hypoplasia, the affected lung and pulmonary artery are absent or small, and there is shift of mediastinal structures toward that side (Fig. 17.1-3A,B). The contralateral lung may be normal in size but is often hyperdistended. MRI may be helpful to exclude underlying lung mass (Fig. 17.1-3C).
FIGURE 17.1-2: Pulmonary hypoplasia and hydrops in a fetus at 27 weeks’ GA. A: Axial ultrasound image of small lungs and bilateral pleural effusions (arrows). H, Heart. B: Coronal T2 MR image of the chest demonstrates marked pulmonary hypoplasia. The lungs are small and dark T2 signal (solid straight arrow) and are surrounded by hyperintense pleural fluid (dashed arrows). Ascites (curved arrow) and skin thickening (arrowhead) are also present.
FIGURE 17.1-3: Unilateral pulmonary hypoplasia in a fetus at 25 weeks’ GA. Gray scale ultrasound (A) and color Dopper (B) axial images through the chest demonstrate markedly small right lung with rightward mediastinal shift and overexpansion of the left lung. Arrow in B shows normal-sized left pulmonary vessels but lack of visualization of right. C: Axial T2-weighted MR demonstrates diminished signal intensity in the affected right lung. H, heart; LL, left lung; RL, right lung.
Prognosticators: Gray scale US can assess fetal lung size by two-dimensional (2D) sonographic parameters, including thoracic circumference (TC) (Table 33 in Appendix A1), thoracic area, and lung length. The TC is measured by the axial plane, along the outer margin of the thoracic cage at the level of the four chambers of the heart and the atrioventricular valves during fetal diastole (Fig. 17.1-4). Ratios utilizing these parameters may be calculated to adjust for gestational age (GA) or fetal size, including a ratio of TC to abdominal circumference (AC),23,24 gestational age, or femur length,25 thoracic area/heart area26 (Fig. 17.1-5), and (thoracic area-heart area) × 100/thoracic area.26 Despite their high specificity, 2D sonographic parameters, with the exception being the lung area-to-head circumference ratio (LHR) discussed in the CDH section of this chapter, have been shown to be less sensitive than preferred for clinical practice.27
FIGURE 17.1-4: Normal thoracic circumference. Axial four chamber view at the level of the atrioventricular valves shows normal thoracic circumference measurement. H, heart.
FIGURE 17.1-5: Graph illustrating distribution of chest area-to-heart area ratios of 13 fetuses at risk for pulmonary hypoplasia. Solid triangles, lethal pulmonary hypoplasia; open triangles, no lethal pulmonary hypoplasia. (Reproduced with permission from Vintzileos AM, Campbell WA, Rodis JF, et al. Comparison of six different ultrasonographic methods for predicting lethal fetal pulmonary hypoplasia. Am J Obstet Gynecol. 1989;161:606–612.)
Three-dimensional (3D) US, which can correct for contour irregularities, has been shown to produce reliable lung volume measurements (Table 34 Appendix A1) and may be useful in the assessment of pulmonary hypoplasia28–30 (Fig. 17.1-6). There are two main approaches to obtaining lung volume measurements by 3D US. The multiplanar approach involves scrolling through the lungs in one reference plane, while simultaneously displaying three orthogonal planes. Alternatively, virtual organ computer-aided analysis (VOCAL) allows volume calculation around a fixed axis in a sequential number of steps.31 Nomograms for right and left lung volumes versus estimated fetal weight have been shown to be reliable for prediction of hypoplasia despite differing methodologies and sonographic equipment across studies29,32 (Tables 35 and 36 in Appendix A1).
FIGURE 17.1-6: Three-dimensional multiplanar imaging of the fetal thorax and measurement of the contralateral (right) lung volume at 23 gestational weeks in a case of left congenital diaphragmatic hernia. A: Transverse plane. B: Sagittal plane. C: Coronal plane. D: 3D rendering of the right lung, the volume of which is 3.87 cm3
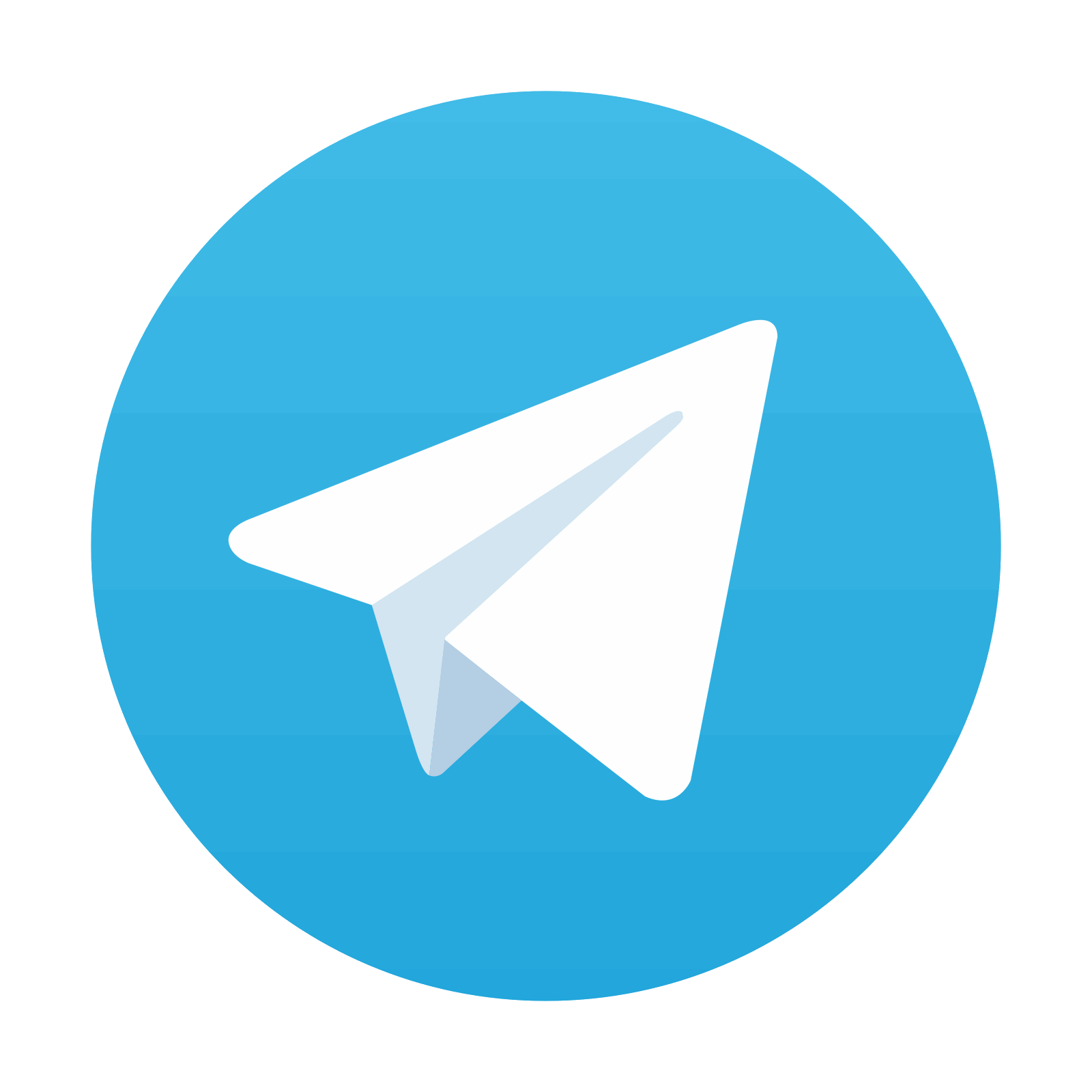
Stay updated, free articles. Join our Telegram channel

Full access? Get Clinical Tree
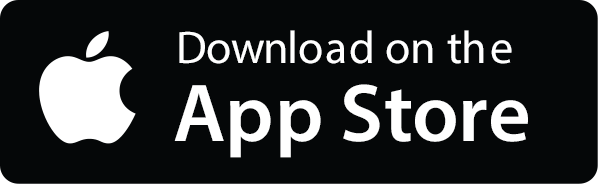
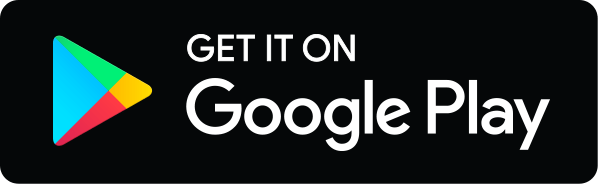