CHAPTER 4 This chapter describes the radiology of many common injuries to the lower extremities in adults. Fractures of the proximal femur are common only in the elderly because of osteoporosis (see Chapter 15); more than 95% of these fractures occur in patients older than 50 years, and the incidence is increasing as the population ages. The eventual mortality rate associated with hip fractures approaches 20%, and many survivors lose their independence of movement. Ninety-nine percent of fractures of the proximal femur are caused by simple falls. Even osteoporotic femurs are resistant to the compressive and tensile forces that occur during normal weight bearing, but there is great vulnerability to torsional or shearing stress. The roles of preexisting insufficiency microfractures in osteoporotic bone and decreased muscle tone as they relate to proximal femur fractures are unclear. Patients present with a painful, shortened, externally rotated limb that is unable to bear weight. Femoral fractures in persons younger than 40 years result from high-energy trauma and usually have associated injuries. Fractures of the proximal femur can be classified as intracapsular in 37% of cases, intertrochanteric in 49%, and subtrochanteric in 14%. Women sustain three to six times as many intracapsular fractures as men, but the incidence of intertrochanteric fractures is equal between the sexes. The initial evaluation in a suspected fracture should begin with radiographs. If a fracture is not identified and there is high clinical suspicion of a fracture, MRI should be obtained. If MRI is not available, CT or radionuclide bone scan should be used, although they are slightly less accurate than MRI. In the elderly population, fractures may not show radionuclide uptake until several days after the injury. A patient who was ambulatory before the fall but is unable to bear weight afterward should be presumed to have a fracture until proved otherwise. The capsule of the hip joint encloses the head and most of the neck of the femur, extending from the acetabulum to the intertrochanteric line anteriorly and to the midneck posteriorly. Most femoral neck fractures are intracapsular and therefore heal less rapidly (synovial fluid lyses clot) than intertrochanteric and subtrochanteric fractures. In addition, the vascular supply to the head is largely disrupted. Subcapital femoral neck fractures extend transversely across the neck, just below the femoral head. Intracapsular fractures can be classified as either nondisplaced (Fig. 4.1) or displaced (Fig. 4.2). Fractures through the base of the neck are similar to two-part intertrochanteric fractures in mechanism and treatment (discussed in the next section). The greater the displacement, the greater the likelihood of subsequent femoral head osteonecrosis. With displaced fractures, the incidence of osteonecrosis and nonunion is approximately 25%. Patients with impacted fractures are usually ambulatory with an antalgic limp and pain that is referred to the groin or the medial knee. Nondisplaced fractures have no inherent stability and may displace with continued ambulation. Femoral neck fractures heal by endosteal callus formation. Nondisplaced fractures are usually fixed with multiple pins placed in parallel; displaced fractures may be reduced and fixed with a variety of methods, including multiple pins, a telescoping hip screw, or some combination thereof. When the risk of femoral head osteonecrosis is high or the quality of trabecular bone is too poor for internal fixation, femoral endoprosthesis or total hip replacement may be used as primary treatment. FIGURE 4.1. Impacted subcapital femoral neck fracture in an elderly woman. A: AP radiograph shows subtle contour abnormality of the lateral cortex at the head-neck junction (arrow) with linear density traversing the neck (arrowhead). B: Frog lateral radiograph shows buckled posterior cortex (arrow). FIGURE 4.2. Displaced subcapital femoral neck fracture (arrows). A: AP radiograph. B: True lateral radiograph. The major fracture line of intertrochanteric fractures extends diagonally from superolateral (greater trochanter) to inferomedial (lesser trochanter). Most intertrochanteric fractures are comminuted, with the greater and lesser trochanters sometimes present as separate fragments (Fig. 4.3). In describing these fractures, the major fragments and the fracture lines should be enumerated in relation to the trochanters, the neck, and the shaft. The degree of displacement and comminution and the presence or absence of subtrochanteric extension along the femoral shaft affect the orthopedic treatment plan and prognosis. Open reduction and internal fixation with a dynamic hip screw are usual. Unlike intracapsular femoral neck fractures, these injuries tend to heal promptly and without complication. The incidence of avascular necrosis of the femoral head is approximately 1%. Incomplete intertrochanteric fractures may occur in elderly adults as a result of falls. Radiographs in this circumstance may be normal, but MRI will demonstrate the fracture (Fig. 4.4). Isolated fractures of the greater trochanter occur in elderly adults as a result of direct trauma sustained in falls. Isolated fractures of the lesser trochanter in adults are pathologic and usually indicate an underlying osseous metastasis (see Chapter 10). FIGURE 4.3. Four-part intertrochanteric fracture with greater and lesser trochanters as separate fragments. FIGURE 4.4. Incomplete intertrochanteric fracture (arrows) on coronal T1-weighted MRI. Subtrochanteric fractures in the elderly may represent extensions of intertrochanteric fractures into the shaft. In other age groups, subtrochanteric fractures are sustained in high-energy trauma such as motor vehicle collisions. The opposing pull of the gluteal and iliopsoas muscles and the thigh adductor muscles distracts and angulates the major fracture fragments. The cantilevered configuration of the femoral shaft and neck creates large stresses on the medial femoral cortex that complicate orthopedic management. A possibility of a pathologic or insufficiency fracture must be considered in subtrochanteric fractures that occur in the absence of significant trauma. Fractures of the femoral shaft occur typically in young adults as a result of major blunt force trauma, such as motor vehicle crashes. Because the femur is the largest and strongest bone in the human body and is protected by the largest and strongest muscle groups, considerable force is necessary to fracture it. Blood loss of two or more units typically accompanies an acute femoral shaft fracture. When the femoral shaft fractures with minimal trauma in an adult, an underlying pathologic lesion should be sought. Femoral fractures can also occur when there is advanced bony demineralization (Fig. 4.5). Insufficiency fractures in the elderly with osteoporosis sometimes may only be identified with MRI. FIGURE 4.5. Osteoporotic supracondylar femur fracture. Lateral radiograph shows fat-fluid level (arrow). Radiographs are frequently obtained in the initial evaluation of knee injuries. Radiographs may show fractures, joint effusion, and dislocation or subluxation. Small avulsion fractures, particularly of the fibular head, medial femoral condyle, lateral tibial margin, or tibial spines, may indicate major ligamentous disruptions. When the mechanism of injury or the clinical examination suggests a ligament, cartilage, or other soft-tissue injury, MRI is the imaging method of choice. The extensor mechanism of the knee is composed of the quadriceps muscles and tendons, the patella, and the infrapatellar tendon. The patella is a large sesamoid bone. The quadriceps and infrapatellar tendons are continuous with each other across the patella; the medial and lateral retinacula are tendinous extensions that pass beside the patella. Injuries to the extensor mechanism may result from forced flexion during strong quadriceps contraction. With complete disruption of the extensor mechanism, voluntary knee extension is lost; a partial disruption results in weakness of extension. In the adult, the extensor tendons are thinnest where they pass over the patella. Tear of the tendon coupled with a fracture of the patella from tensile loading results in a transverse patellar fracture with the fragments distracted by the quadriceps muscle (Fig. 4.6). The quadriceps and infrapatellar tendons are also subject to injury. Quadriceps tears usually occur at the musculotendinous junction in patients who have degenerative changes in their tendons, resulting in a low-lying patella (patella baja). Quadriceps tendon injuries are best demonstrated by MRI (Fig. 4.7). Infrapatellar tendon tears are seen most often in young adult athletes. In complete tears, superior retraction of the patella by unopposed pull of the quadriceps results in a high-riding patella (patella alta) (Fig. 4.8). Partial tears of the infrapatellar tendon are seen as increased T2-weighted signal within the tendon on MRI (Fig. 4.9). Stellate fractures of the patella may be caused by direct trauma. The extensor mechanism remains intact, and the fragments are not distracted. Hemarthrosis in the knee capsule is invariably present (Fig. 4.10). FIGURE 4.6. Avulsion fracture of the inferior pole of the patella (arrow). FIGURE 4.7. Quadriceps tendon tear. Sagittal proton density MRI shows discontinuity of the tendon (arrow) at the superior pole of the patella. FIGURE 4.8. Infrapatellar tendon sprain (grade 3). Proximal retraction of the patella by the quadriceps muscles. Note the high position of the patella (patella alta). FIGURE 4.9. Infrapatellar tendon sprain (grade 2). Sagittal PD MRI shows partial tear of the infrapatellar tendon (arrow) at its insertion on the anterior tibial tubercle. The patella has not retracted proximally because the tear is incomplete. FIGURE 4.10. Minimally displaced comminuted patellar fracture (long arrow). Lateral radiograph with horizontal X-ray beam demonstrates a fat-fluid level, (short arrow) indicative of intra-articular fracture. Patellar dislocations are typically lateral and may occur with flexion and internal rotation of the femur on a fixed tibia. Impaction injuries of the medial patella and lateral femoral condyle are characteristic of patellar dislocation (Fig 4.11), and associated tears of the medial patellar retinaculum (Fig. 4.12), MCL, and ACL may be found. Chondral or osteochondral fractures may involve either the patella or the femoral trochlea, sometimes with displaced fragments (Fig. 4.13; see also Fig. 1.34). FIGURE 4.11. Lateral patellar dislocation. Axial inversion recovery MRI shows reduced lateral patellar dislocation with bone bruises of the medial patellar facet (short arrow) and lateral femoral condyle (long arrow). There is a sprain of the medial patellar retinaculum (arrowhead) and a large joint effusion. FIGURE 4.12. Medial retinaculum tear postpatellar dislocation. Axial T2-weighted fast spin echo MRI of the knee shows tear of the medial retinaculum (arrow). FIGURE 4.13. Axial T2-weighted fat-suppressed MRI shows reduced lateral patellar dislocation with medial retinacular separation (arrowhead) and osteochondral injuries of the patella (short arrow) and lateral femoral trochlea (long arrow). Similar to the spine, the knee may be thought of as an articulated column stabilized by surrounding soft-tissue structures. The joint capsule invests the entire knee and supports it on all sides. The medial side is also supported by the MCL and medial retinaculum; the lateral side by the LCL complex (iliotibial band, LCL itself, biceps femoris tendon) and lateral retinaculum; the anterior aspect by the structures of the quadriceps mechanism; and the posterior aspect by the gastrocnemius muscles. The posterolateral corner has the popliteus muscle and tendon and arcuate ligament; the posteromedial corner has the semimembranosus tendon and posterior oblique ligament. The ACL and PCL occupy the central portion of the knee, with the ACL lateral to the PCL. The medial and lateral menisci deepen the articular surface of the tibial plateaus. When the extended knee is loaded by bending forces, the structures on the concave side of the bend are compressed, and the structures on the convex side are distracted. Compressive injuries include bone bruises and impaction fractures; distractive injuries include tears of ligaments, tendons, muscles, and capsule, or avulsions of their bony attachments. Under valgus loading, an MCL injury is combined with lateral compartment bone bruises or impaction fractures. If the loading is severe enough, the ACL and PCL also tear under tension (Fig. 4.14). Under varus loading, the LCL complex tears under tension, and the medial compartment sustains the compressive injury; with severe loading, the ACL and PCL also tear (Fig. 4.15). With hyperextension, the compressive injury involves the contiguous anterior aspects of the femur and tibia (see Fig. 1.11), and the tensile injury involves the posterior capsule and gastrocnemius muscles; if there is accompanying anterior or posterior translation of the tibia, the ACL or PCL may tear, respectively. With hyperextension-valgus loading, the compressive injury is anterolateral, and the distractive injury involves the posteromedial corner, the MCL, and the PCL. With hyperextension-varus loading, the compressive injury is anteromedial, and the distractive injury involves the posterolateral corner and ACL (Fig. 4.16). FIGURE 4.14. Valgus knee injury in a pedestrian struck by a car. A: Coronal T2-weighted fat-suppressed MRI shows torn MCL (black arrow) and lateral condyle bone bruise (white arrow). B: Sagittal proton density MRI shows torn ACL (arrow). C: Sagittal proton density MRI shows torn PCL (arrow). FIGURE 4.15. Varus knee injury in pedestrian struck by car. A: Coronal inversion recovery MRI shows tears of the LCL complex, including an avulsion of the iliotibial tract (black arrow). There is a compression injury on the medial side with tibial bone bruise (white arrow) and medial meniscal tear. B: Sagittal PD fat-suppressed MRI shows ACL tear (arrow). C: Sagittal PD fat-suppressed MRI shows PCL tear (arrow). FIGURE 4.16. Hyperextension-varus injury. Axial inversion recovery MRI shows anteromedial bone bruise (short arrow) in the distal femur and posterolateral corner injury (long arrow). When the flexed and externally rotated knee sustains valgus stress, the ACL and the MCL are loaded under tension. If the ACL tears, the tibia is able to translate anteriorly beneath the femur and impact the lateral condyle, resulting in the pivot-shift bone bruise pattern that is highly correlated with ACL tears (Fig. 4.17). The medial and lateral menisci, which act as buttresses against translation of the knee, may also be torn. With weight-bearing or axial compression, meniscal tears are more likely. The combination of tears of the ACL, the MCL, and a meniscus (usually lateral) is called the unhappy triad; if both menisci are torn, it is an unhappy tetrad. Much less commonly, varus stress on the internally rotated and flexed knee may result in tears of the ACL, lateral, and posterolateral structures; the Segond fracture may be part of this injury complex (Fig. 4.18). FIGURE 4.17. Flexion-external rotation-valgus injury (unhappy triad). A: Sagittal T2 fat-suppressed MRI through the lateral compartment shows impaction fracture of the anterior aspect of the lateral femoral condyle (long arrow) and bone bruise in posterolateral tibia (short arrow) (pivot shift pattern). B: Sagittal T2 fat-suppressed MRI through the intercondular notch shows ACL tear (arrow). C: Coronal inversion recovery MRI shows MCL sprain, grade 2 (arrow). A lateral meniscal tear was also present (not shown).
Trauma in Adults: Lower Extremity
PROXIMAL FEMUR
Intracapsular Fractures
Intertrochanteric Fractures
Subtrochanteric Fractures
SHAFT AND DISTAL FEMUR
KNEE
Extensor Mechanism
Complex Knee Injuries
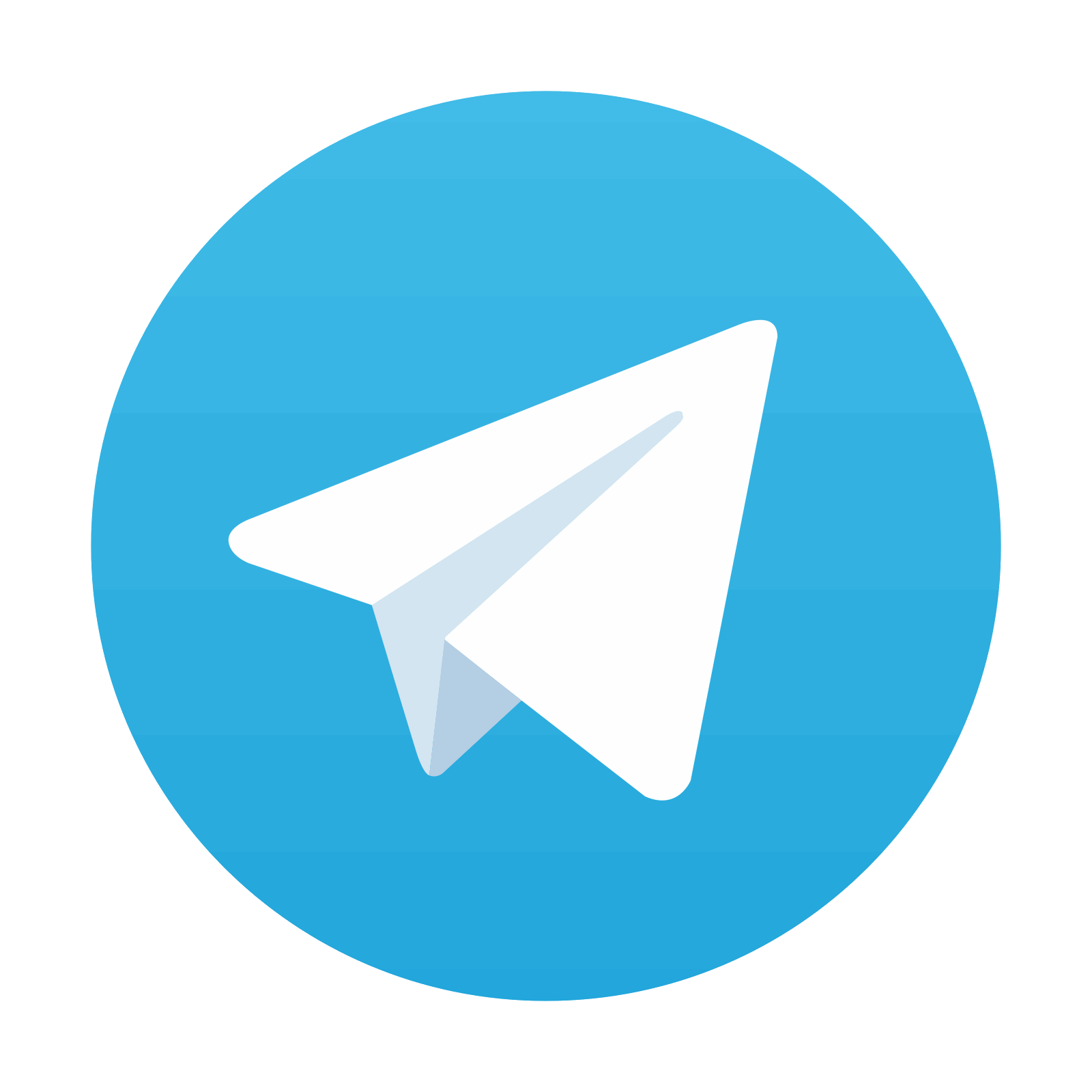
Stay updated, free articles. Join our Telegram channel

Full access? Get Clinical Tree
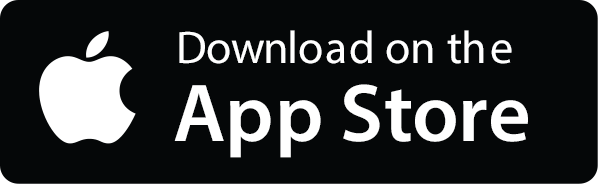
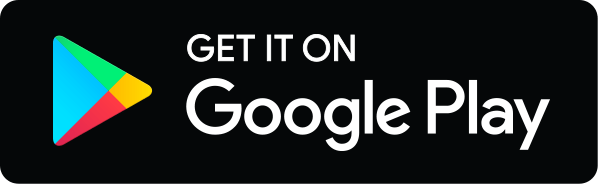