Study
Site
Technique
# of patients
Measurement
Repeatability (1SD, CC)
Hanley et al. (1999) [30]
Lung
DIBH
9
Diaphragm
0.9 mm
Remouchamps et al. (2003) [31]
Breast
mDIBH
14
Lung surface
1.1 mm
Dawson et al. (2001) [32]
Liver
ABC
8
Diaphragm
2.5 mm
Eccles et al. (2006) [33]
Liver
ABC
21
Diaphragm
1.5 mm
Koshani et al. (2006) [34]
Lung
ABC
10
GTV
1.4 mm
Hurst et al. (2010) [35]
Lung
ABC
9
GTV
Mean < 2.0 mm
Nakamura et al. (2010) [36]
Pancreas
Visual feedback
10
GTV
1.0 mm
Peng et al. (2011) [37]
Lung
Visual feedback
13
GTV
1.3 mm
9.3.2.3 Respiratory-Gating and Real-Time Tumor Tracking
Breath-hold CT scans can also potentially be used during respiratory-gated and real-time tumor tracking irradiation; however, it should be noted that a 4D CT scan is preferred over a breath-hold CT scan taken at the same respiratory position, because the respiratory muscles used during breath-holding and free breathing may differ, and any tumor lag occurring during free breathing will be absent during breath-hold. It is also known that the positions of implanted markers do not always represent the tumor position because the tumor and the markers move at different rates during respiration [38]. The ITV should consider such intrafraction variations and potential changes in internal-external correlation factors [39, 40], both within the gating window and the entire cycle of respiration.
9.4 Targeting
The literature shows clearly shows that the single largest systemic source of error in radiation therapy is target delineation. Such errors can be minimized only by using site-specific delineation protocols and consensus delineation atlases. Inconsistencies in target delineation are considered to be significant sources of uncertainty in treatment planning [41]. In defining the gross tumor volume (GTV), clinical target volume (CTV), ITV, and planning target volume (PTV) when performing SBRT, the data in the International Commission on Radiation Units and Measurements (ICRU) Reports 50 [42] and 62 [43] must be taken into account.
9.4.1 GTV and CTV
The GTV (the primary tumor) may be identified using any of several imaging modalities, and should include any grossly involved lymph nodes. A CTV is an anatomically defined area (perhaps including the hilar or mediastinal lymph nodes, or a margin around a grossly visible tumor) believed to harbor micrometastases. One radiographic/histopathological study of lung parenchymal disease [44] found that GTV-to-CTV expansions of 6 mm (squamous cancers) and 8 mm (adenocarcinomas) were required to cover both the gross tumors and microscopic disease with 95 % accuracy. Grill et al. concluded that the CTV expansion required to cover microscopic extensions in 90 % of cases could be as high as 9 mm [45]. When SBRT is used, however, the GTV and CTV are often considered to be identical [46–48]. The typically very high local control rates reported after SBRT suggest that peripheral tumor components, if present, seem not to be a major cause of recurrence.
9.4.2 ITV
To address the issue of tumor motion, ICRU Report No. 62 [43] proposed use of an ITV, defined as an expansion of the CTV to explicitly reflect target motion. Several approaches have been taken to address the issue of intra- and interfraction motion, which in turn determines the ITV. Details of ITV determination for each type of beam are described in Sect. 9.3.
9.4.3 PTV
The PTV seeks to manage inaccuracies caused by daily setup of fractionated therapy, mechanical uncertainties of the equipment, and dosimetric uncertainties. When using internal surrogates, amount of marker migration and fixation rate were dependent on the procedure of implantation [38, 49, 50]. Since these may vary from center to center and, within a given center, from machine to machine, these uncertainties should also be included in a PTV.
9.5 Beam Arrangement
The goal of SBRT treatment is to ablate tissues within the PTV; destruction of such tissues is not considered to cause complications. Dose inhomogeneity inside a PTV is usually considered to be acceptable and need not be addressed as a priority during planning. Thus, a maximum point dose of up to around 160 % of the prescription dose is common in SBRT plans (Figs. 9.1, 9.2, and 9.3). Recently, treatment planning has been accomplished using standard beams, static conformal arcs, dynamic conformal arcs, IMRT, VMAT, or hybrid plans. Three-to-six non-coplanar arcs and five-to-nine (or more) non-coplanar static fields are commonly used. Such an approach optimally requires that radiation should converge on the target in as concentric a manner as possible, from many directions. Adjustment of the MLC margin is a key in terms of the dose distribution [51] (Fig. 9.1). If the MLC margin is close to the beam penumbra [Fig. 9.1 (Left)], a homogeneous PTV dose, the maximum of which is ~110 % of the prescription dose, can be achieved (Figs. 9.2 and 9.3); however, dose fall-off outside the PTV is slow. If the MLC margin is fit to or much less than the beam penumbra [Fig. 9.1 (Right)], dose fall-off outside the PTV is rapid, but the PTV dose can be inhomogeneous in that a maximum dose of ~125 % or more of the prescription dose can be attained (Figs. 9.2 and 9.3).
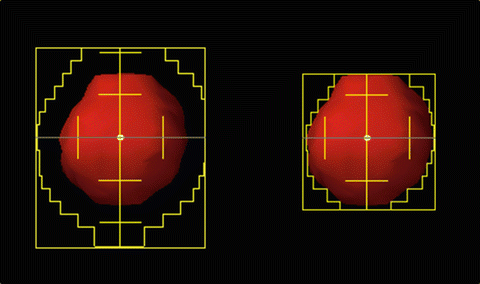
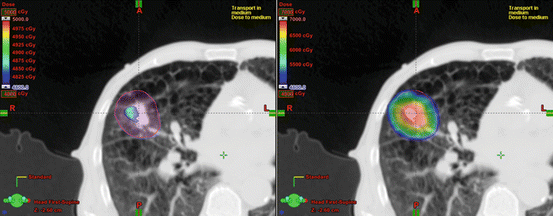
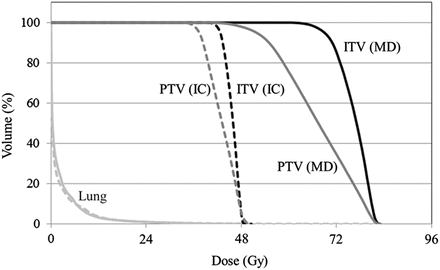
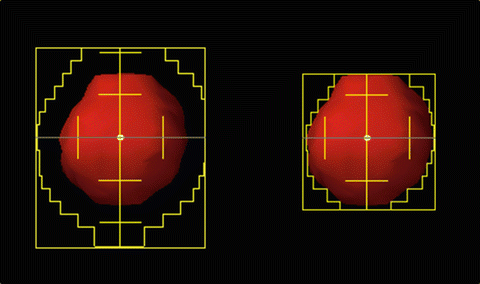
Fig. 9.1
Beam’s eye view for (Left) isocenter prescription and (Right) marginal dose prescription. For isocenter prescription, the beam’s eye view MLC aperture was often larger than the PTV. Meanwhile, the beam’s eye view MLC aperture was typically fit to or smaller than the PTV edge for marginal dose prescription
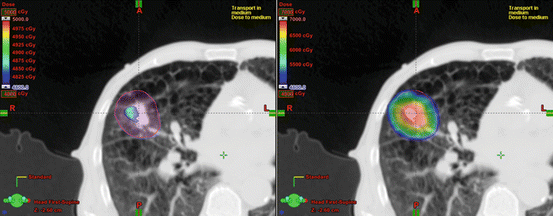
Fig. 9.2
Comparison of dose distributions. PTV is indicated by a pink sphere. Dose of greater than 48 Gy is shown. (Left) Isocenter prescription. The prescribed dose is 48 Gy at isocenter. PTV is not covered by prescribed dose of 48 Gy. (Right) Marginal dose prescription. The prescription dose of 48 Gy is given to the isodose line encompassing 100 % of the PTV
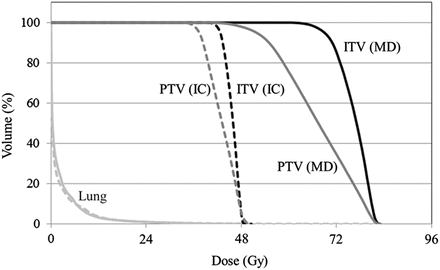
Fig. 9.3
Comparison of dose volume histograms. Marginal dose prescription (MD) vs. isocenter prescription (IC). Note that lung doses with marginal dose prescription were almost identical to those with isocenter prescription
9.6 Beam Energy
For small beams, such as those commonly used in SBRT, the higher the beam energy, the larger the beam penumbra, due to lateral transport of electrons in the medium. In a low-density medium, such as lung tissue, this effect becomes more significant. Use of a lower photon beam, such as 6-MV, available on most modern treatment platforms, affords a reasonable compromise between beam penetration and the required penumbral characteristics for SBRT lung applications.
9.7 Dose Calculation
It is preferable that CT images used for dose calculation reflect respiratory status during beam delivery. As shown in Table 9.2, dose calculation should be performed using the CT dataset that is most appropriate for each patient.
Table 9.2
Ideal CT images used for dose calculation prior to use of particular beam delivery techniques
Beam delivery | Respiratory status during beam delivery | CT images |
---|---|---|
Inhibiting respiratory movement with abdominal compression | Forced shallow breathing | AIP or slow CT images with abdominal compression |
Breath-holding | Breath-holding | Breath-holding |
Respiratory gating | Free breathing (w/o abdominal compression) | Phase-specific images of 4D CT |
Real time tumor tracking | Free breathing (w/o abdominal compression) | Phase-specific images of 4D CT |
The dose calculation algorithm (including a heterogeneity correction), and the grid size for calculation, used in any treatment planning system, affect the accuracy of the calculated dose distribution. Inaccurate dose calculation causes large discrepancies between planned and actually delivered doses; therefore, it is recommended that high-precision dose calculation algorithms with fine grid sizes be employed. The technical details of dose calculation have been discussed in this chapter.
9.8 Normal Tissue Dose Tolerance
Normal tissue dose limits for SBRT differ considerably from those of conventional radiotherapy because the dose/fractionation schemes are extreme. Thus, normal tissue dose limits for SBRT should not be directly extrapolated from conventional radiotherapy data. Particular attention should be paid to fraction size, total dose, time elapsing between fraction deliveries, and overall treatment time; these are important radiobiological factors that need to be maintained within clinically established parameters (often described in the SBRT literature). This issue assumes particular importance when planning new hypofractionated schedules and trials for which no reliable mechanism has yet been established to estimate the radiobiological effects. Therefore, in a clinical trial situation, not only fraction size, but also treatment frequency and overall treatment time, should be maintained for all patients throughout the entire trial, to obtain reliable outcome data. Critical organ tolerance doses based on SBRT experiences appearing in the evolving peer-reviewed literature must be respected [52, 53].
9.9 Treatment Plan Reporting
SBRT treatment plans often use a large numbers of beams, unconventional dose fractionations, and varying delivery frequencies. It is critical to accurately communicate the details of the treatment plan and the execution thereof to the treatment team. The quality of planned SBRT dose distributions can be evaluated using parameters characterizing target coverage, dose homogeneity, dose delivered outside of the defined target, and the volume of normal tissue exposed to lower doses. Simple methods of describing these parameters may use combinations of DVHs for different organs and tables showing dose allocations to different subvolumes of such organs. The following metrics are relevant: “respiratory motion range”, “dose calculation algorithm”, “prescription dose”, “prescription ICRU reference point or dose/volume”, “number of treatment fractions”, “total treatment delivery period”, “target coverage”, “heterogeneity index”, “conformity index”, and “dose to organs at risk”.
References
1.
Keall PJ, Mageras GS, Balter JM, Emery RS, Forster KM, Jiang SB, et al. The management of respiratory motion in radiation oncology report of AAPM Task Group 76. Med Phys. 2006;33:3874–900. doi:10.1118/1.2349696.PubMedCrossRef
2.
Seppenwoolde Y, Shirato H, Kitamura K, Shimizu S, van Herk J, Lebesque JV, et al. Precise and real-time measurement of 3D tumor motion in lung due to breathing and heartbeat, measured during radiotherapy. Int J Radiat Oncol Biol Phys. 2002;53:822–34. doi:10.1016/S0360-3016(02)02803-1.PubMedCrossRef
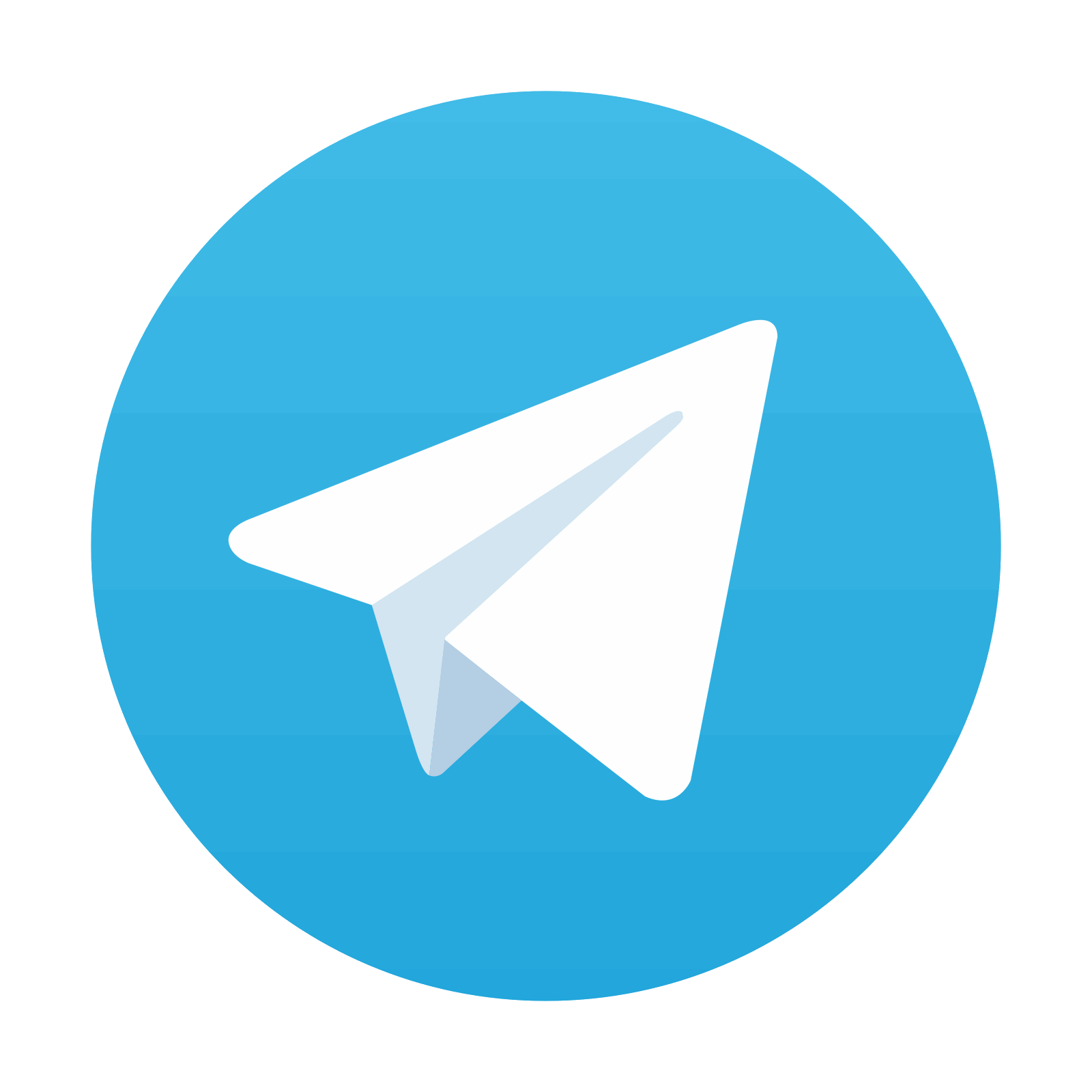
Stay updated, free articles. Join our Telegram channel

Full access? Get Clinical Tree
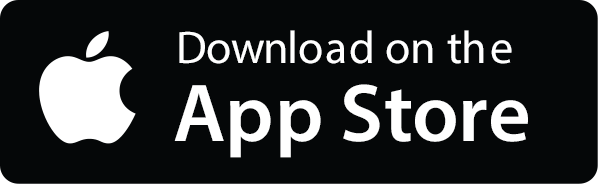
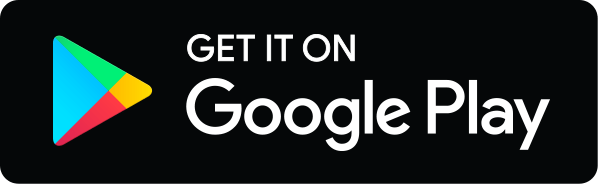