Structural imaging studies of autism have documented increased total brain volume and early acceleration in brain growth. Functional MR imaging (fMRI) studies in autism have also led to the view of autism as a disorder of cortical functioning in which functional connectivity or synchronization of brain regions and cortical activity are disturbed with atypical cortical specialization.
This article focuses on the valuable and unique contributions that structural and functional imaging studies of autism have made to the understanding of the brain and mind. Structural imaging studies have documented increased total brain volume and early acceleration in brain growth. This overgrowth is particularly conspicuous in cerebral white matter, highlighting the role of white matter in neural connectivity. However, the presentation of autism as a developmental disorder is very different than a disorder associated with damage to white matter, such as cerebral palsy. The stark contrast between increased white matter volume and poor clinical and brain function highlighted the dissociation between white matter tract overgrowth and dendritic and synaptic underdevelopment. Hence, white matter tract overgrowth is not necessarily synonymous with connectivity at all histological levels. The next lesson learned was that white matter was not the whole story. A series of recent proton (1H) MR spectroscopy (MRS) studies have documented the involvement of cerebral cortex, and in fact perhaps its primacy, as the site of origin of the neurological abnormalities. These studies have served as a reminder that white matter tracts have an origin in the neuron cell body. The lack of increased white matter in the corpus callosum, rather the opposite, suggests that the primary pathophysiology is intrahemispheric and not specific to white matter. More sensitive imaging measures of brain tissue volume from voxel-based morphometry have demonstrated a consistent pattern of cortical gray and white matter volume alterations across autism and Asperger’s disorder in British and Chinese populations, using standardized methods and automated processing that can be used reliably across different laboratories. The lesson of structural imaging in the developmental disorder of autism is that, if structural anatomy is to be defined across the age span of development and brain maturation that is prolonged, large samples in small age bins, and age-, gender-, and IQ-matched control groups are necessary.
Functional MR imaging (fMRI) studies of autism have brought many insights about the brain and mind. Although there is not space here to review all such literature, autism has been instrumental in defining many neural systems related to social interaction (theory of mind and the mirror neuron system), gaze and motion processing, face identity, face emotion and emotion processing, visual perception and higher order perceptual processing and, importantly, the difference between automatic processing and deliberate conscious or cognitive processing, among others. These neural systems and others have slowly helped us to understand how people think and how the mind works, and with this understanding has come a much improved understanding of behavior in autism, and an emerging generation of intervention strategies that focus on cognitive interventions aimed at changing neural circuitry. Functional MRI studies in autism have also led to the view of autism as a disorder of functional connectivity among brain regions, and as a disorder in which cortical activity is disturbed and cortical specialization is somewhat atypical. The first level of connectivity analyses revealed a decrease in the connectivity of all lobes with the frontal lobe and an increase in right posterior activity compensating for underfunctioning regions. A related lesson to come from these studies was that brain localization cannot be inferred from a cognitive or neuropsychological task, because individuals who had autism were shown on fMRI to use alternate regions to compensate for dysfunctional brain regions such as dorsolateral prefrontal cortex during verbal working memory. The inability to do so during spatial working memory resulted in a dissociation in performance between clinically intact verbal working memory and impaired spatial working memory that was not understood until fMRI studies were completed. The functional underconnectivity appears to be a universal characteristic of the brain in autism, reflecting a disturbance in synchronization. The slightly atypical cortical localization of regions on fMRI suggests that the basic mechanisms of inhibition-excitation may also be disturbed. Much remains to be learned about the mind and brain from autism, and the knowledge of the future will be even more exciting.
In the Diagnostic and Statistical Manual of Mental Disorders-Fourth Edition (DSM-IV) , autism is the prototype disorder for the category called pervasive developmental disorders (PDDs). Of the PDDs, autistic disorder, Asperger’s disorder, and pervasive developmental disorder not otherwise specified (PDD-NOS) are informally referred to as the autism spectrum disorders (ASDs), which may affect up to 1% of children born . PDDs are defined by impairments in reciprocal social skills, in the comprehension and communicative use of verbal and nonverbal language, and by restricted and repetitive behaviors . The latter cluster of behaviors share a focus on the details of an object or category in conjunction with a failure to grasp the conceptual meaning of the object or category as a whole. All of the impairments that define the ASDs are the result of the failure to process information in the same way as typical people, producing a different perception and view of the world. PDD-NOS and Asperger’s disorder are likely milder consequences of whatever causes autism, although some argue for them being biologically distinct disorders.
Neuroimaging studies have contributed substantially to the understanding of the neurobiology of autism and, in particular, to understanding the important distinction between developmental neurobiological disorders and acquired disorders. Findings in autism have given proof to the statement that in the brain, “The way things are broken is not necessarily the way they are made” (Antonio Damasio, personal communication, 1990). The study of autism has also contributed to the understanding of neural systems supporting higher-order cognitive and neurologic processes, and of the neural basis of complex behavior, automatic thought, and the experience of emotions (feelings); eg, the mind.
The development of the present day understanding of the neural basis of the ASDs was initially very slow, but has rapidly accelerated over the past 15 years. The first 30 years of neurobehavioral research in autism (1950s–1970s) was marked by erroneous analogies to adult neurological conditions secondary to acquired brain damage, and by simple cognitive models for the abnormal complex behavior of autism, without supporting data. The emergence from this phase began with computerized axial tomographic (CT) studies that demonstrated the connection between gross structural brain abnormalities and the coexistence of associated causes of brain damage in subjects being diagnosed with autism at that time . Thereafter, it became standard research practice to exclude subjects from research studies with causes for brain dysfunction other than autism. Of equal importance, structured research instruments, such as the Autism Diagnostic Interview (ADI) and the Autism Diagnostic Observation Schedule (ADOS) , were developed and introduced with worldwide uniformity in training and reliability procedures, which substantially improved the specificity and sensitivity of autism and autism spectrum diagnoses and standardized diagnosis across research sites. These contributions to subject characterization provided the foundation for the emergence of the contemporary neurobiology of autism.
The imaging contributions to the understanding of the brain and mind in autism can be divided into those arising from structural imaging studies and those arising from functional imaging research. Although these advances have come from the combined contributions of multiple methodologies, imaging has certainly made valuable and unique contributions. These contributions are the focus of this article.
The enlarged brain and early brain overgrowth: the wake-up call
The contemporary neurobiology of autism began with the recognition that the brain in most young children who have autism is too large. The initial characterization of brain size and growth in autism took a decade or more, and relied heavily on head circumference data. Ultimately, head circumference studies in autism defined a group mean head circumference (HC) at the 60th through 70th percentile relative to population norms, and disproportionate to height (HT) and weight (WT); 15% to 20% of the autism group were found to have macrocephaly (HC >99th percentile) . Although larger HC for HT was the most common finding and true for the group as a whole, it was not universal: HC proportionate to HT, and HC less than HT were also frequent in autism . Thus, many head growth trajectories were found to be consistent with autism. Retrospective and prospective HC data from very young children who have autism found onset of accelerated head growth by or before 12 months of age , and macrocephaly in 15% to 20% by 4 to 5 years of age . Growth abnormalities have not been detected at birth relative to population norms.
Structural MR imaging studies confirmed the increase in total supratentorial brain volume (TBV) in autism suggested by the increase in head circumference. The increase in TBV was documented as early as 2 to 4 years of age , the earliest age of clinical recognition, and found to persist into childhood but not adolescence . The rate of brain growth in autism accelerated early in life, then decelerated and plateaued, resulting in changes in brain and head size. These data, however, are not sufficient to define the age of crossover to normal HC and TBV in autism, or to adequately define the heterogeneity in growth trajectories and TBV within the autism population. Cross-sectional data suggested that increased TBV likely persisted until the end of the first decade of life ; however, longitudinal studies of preschool children who have autism have suggested that increased TBV may not persist past 5 or 6 years of age . There are a subset who are macrocephalic throughout life, but this appears to be a separate phenomenon.
The onset of brain overgrowth precedes or coincides with the presentation of the signs and symptoms of autism, indicating that the overgrowth is part of a pathologic process that disrupts brain structure and function, and thus leads to autism. The actual acceleration of growth suggests that the underlying process is related to active growth of central nervous system tissue, rather than a passive process, such as inhibition of pruning. Early in life, increased brain volume is clearly associated with loss of function; however, it is difficult to interpret the significance of changes in regional brain volumes reported at later ages.
Developmental differences in white matter
The tissues contributing to the overall increased TBV were total cerebral white matter and total cortical gray matter; the latter contribution varying with the parcellation program used. One small but very important study for its impact on the understanding of the white matter volume changes in autism involved 6- to 11-year-old boys who had autism (n = 13) and did not have autism (n = 28: 14 developmental language disorder, 14 typically developing). The study examined the parcellation of cerebral white matter into an outer zone of radiate white matter composed of intrahemispheric corticocortical connections and an inner zone of bridging and sagittal components. The inner zone of white matter, including the corpus callosum and internal capsule, showed no volume differences. The volume of the outer radiate white matter was increased in all cerebral lobes, but with a frontal predominance. These findings were interpreted as evidence of overgrowth of short- and medium-range intrahemispheric corticocortical connections (fiber pathways), with no detectable involvement of interhemispheric connections or connections between cortex and subcortical structures . It is important to recognize that the white matter fiber pathways may be larger without actual dendritic or synaptic connections having been established, or if established, without being functional. Hence, the appropriate interpretation of the structural findings is that the white matter pathways are enlarged, not that connectivity per se is increased. It could be inferred that connectivity is likely affected, but its functional status and the status of synapses cannot be discerned from this gross measurement. It is possible that there is an overgrowth of white matter projections because functional synapses are not being established.
Another recent study examined cortical connectivity by comparing gyral and sulcal thickness as indices of short and longer distance cortical connections . This study found an overall increase in cortical thickness in a sample of high-functioning 8- to 12-year-old boys who had autism compared with typical boys. Cortical thickness was greater (analogous to increased volume of outer radiate white matter) in sulci (long connections) than gyri (short vertical connections), analogous to the findings of Herbert and colleagues .
The broader importance of these white matter findings is that they demonstrated that developmental pathology could present very differently from damage to white matter, which traditionally manifested as cerebral palsy and prominence of long tract signs. Autism became a lesson in the expression of developmental pathology of white matter as alterations in brain connectivity, absence of long tract signs, and increased brain size.
Cross-sectional imaging studies in autism have reported a reduction in the size of the corpus callosum, although the segment reported to be affected has varied . In some studies, the decrease in cross-sectional area was only present relative to total brain volume in the autism group ; in other studies, there was an absolute decrease in corpus callosum area independent of brain volume . The contrast between the increase in intra hemispheric white matter volume and the lack of change or decrease in corpus callosum size was notable, and indicated that the neurobiological process affecting intrahemispheric white matter was not impacting interhemispheric white matter. A key question for structural imaging studies is: to what brain structure should the corpus callosum be compared with to determine if its size is abnormal? Because TBV in autism is influenced by its largest component (cerebral white matter), which has no relation to the origin of the corpus callosum, is this an appropriate comparison or the most appropriate comparison?
A study using diffusion tensor imaging (DTI) also found abnormalities in the corpus callosum in individuals who had high-functioning autism and typically developing controls . Functional anisotropy (FA) values were significantly lower in the overall corpus callosum, genu, and splenium of individuals who had high-functioning autism. Additionally, the autism group displayed higher mean diffusivity measurements relative to controls in the overall corpus callosum, genu, and midbody. This study also found that a cluster of individuals who had autism and who had the highest mean diffusivity and lowest FA also had the slowest processing speeds . The relationship of structure and processing speed to cognitive deficits was not demonstrated, but this was a first step toward relating corpus callosum structure to interhemispheric processing alterations in autism. Although there is ample evidence of intrahemispheric processing deficits in autism, the status of interhemispheric processing mediated by corpus callosum remains an unanswered but significant research question. It is not a simple question to address, because the degree of intrahemispheric processing deficit makes it difficult to tease out any additive affect of interhemispheric processing.
Developmental differences in white matter
The tissues contributing to the overall increased TBV were total cerebral white matter and total cortical gray matter; the latter contribution varying with the parcellation program used. One small but very important study for its impact on the understanding of the white matter volume changes in autism involved 6- to 11-year-old boys who had autism (n = 13) and did not have autism (n = 28: 14 developmental language disorder, 14 typically developing). The study examined the parcellation of cerebral white matter into an outer zone of radiate white matter composed of intrahemispheric corticocortical connections and an inner zone of bridging and sagittal components. The inner zone of white matter, including the corpus callosum and internal capsule, showed no volume differences. The volume of the outer radiate white matter was increased in all cerebral lobes, but with a frontal predominance. These findings were interpreted as evidence of overgrowth of short- and medium-range intrahemispheric corticocortical connections (fiber pathways), with no detectable involvement of interhemispheric connections or connections between cortex and subcortical structures . It is important to recognize that the white matter fiber pathways may be larger without actual dendritic or synaptic connections having been established, or if established, without being functional. Hence, the appropriate interpretation of the structural findings is that the white matter pathways are enlarged, not that connectivity per se is increased. It could be inferred that connectivity is likely affected, but its functional status and the status of synapses cannot be discerned from this gross measurement. It is possible that there is an overgrowth of white matter projections because functional synapses are not being established.
Another recent study examined cortical connectivity by comparing gyral and sulcal thickness as indices of short and longer distance cortical connections . This study found an overall increase in cortical thickness in a sample of high-functioning 8- to 12-year-old boys who had autism compared with typical boys. Cortical thickness was greater (analogous to increased volume of outer radiate white matter) in sulci (long connections) than gyri (short vertical connections), analogous to the findings of Herbert and colleagues .
The broader importance of these white matter findings is that they demonstrated that developmental pathology could present very differently from damage to white matter, which traditionally manifested as cerebral palsy and prominence of long tract signs. Autism became a lesson in the expression of developmental pathology of white matter as alterations in brain connectivity, absence of long tract signs, and increased brain size.
Cross-sectional imaging studies in autism have reported a reduction in the size of the corpus callosum, although the segment reported to be affected has varied . In some studies, the decrease in cross-sectional area was only present relative to total brain volume in the autism group ; in other studies, there was an absolute decrease in corpus callosum area independent of brain volume . The contrast between the increase in intra hemispheric white matter volume and the lack of change or decrease in corpus callosum size was notable, and indicated that the neurobiological process affecting intrahemispheric white matter was not impacting interhemispheric white matter. A key question for structural imaging studies is: to what brain structure should the corpus callosum be compared with to determine if its size is abnormal? Because TBV in autism is influenced by its largest component (cerebral white matter), which has no relation to the origin of the corpus callosum, is this an appropriate comparison or the most appropriate comparison?
A study using diffusion tensor imaging (DTI) also found abnormalities in the corpus callosum in individuals who had high-functioning autism and typically developing controls . Functional anisotropy (FA) values were significantly lower in the overall corpus callosum, genu, and splenium of individuals who had high-functioning autism. Additionally, the autism group displayed higher mean diffusivity measurements relative to controls in the overall corpus callosum, genu, and midbody. This study also found that a cluster of individuals who had autism and who had the highest mean diffusivity and lowest FA also had the slowest processing speeds . The relationship of structure and processing speed to cognitive deficits was not demonstrated, but this was a first step toward relating corpus callosum structure to interhemispheric processing alterations in autism. Although there is ample evidence of intrahemispheric processing deficits in autism, the status of interhemispheric processing mediated by corpus callosum remains an unanswered but significant research question. It is not a simple question to address, because the degree of intrahemispheric processing deficit makes it difficult to tease out any additive affect of interhemispheric processing.
Longitudinal studies
To the authors’ knowledge, there are currently three longitudinal studies of preschoolers who have autism . Although the sample sizes for these studies are still relatively small (n = 30 who have autism ; n = 51 who have ASD ; n = 29 who have autism plus n = 16 who have PDD-NOS ), all three studies reported increased TBV or total cerebral volume (TCV) at the 2- to 4-year age point. Two of the studies have reported an increase in cerebellar volume , but the study of the youngest cohort did not find an increase in cerebellar volume . Definitive diagnosis of the subjects in the latter study has not been completed, however, and so subjects who have PDD-NOS have not been separated from those who have autism. The first study reported increased cerebral white (18%) and gray (12%) matter and increased cerebellar white matter (39%) volumes . The study involving the ASD group reported increased TCV and increases in TCV and in bilateral hippocampal and amygdala volumes that were proportionate to the increase in TCV . In the subgroup who had autism in this study, the amygdala enlargement was disproportionate to the increase in TCV. At follow-up at 6 to 7 years of age, an increase in amygdala volume was still present, but hippocampal volume and TCV were the same as controls . The third study reported a significant increase in cerebral volume in their ASD group, but not cerebellar volume . The increase in volumes in the cerebral hemispheres involved both gray and white matter, and was generalized rather than regional. Although informative, these three studies have reported data on only a few structures, and only one study has reported data at the first follow-up . It will be most interesting to find out about total and regional cortical gray and white matter volumes, total and regional area measurements of the corpus callosum, the status of the basal ganglia in all three samples, and the findings at the first and second follow-up times.
Individual structures and nonuniform growth
The increase in brain volume in children who have autism is not uniform. One study of 17 7- to 11-year-old nonretarded boys who had autism and 15 typical boys approached this issue by examining clusters of structures that covaried in size, revealing a cluster that was increased in volume compared with matched controls (cerebral white matter), a cluster that was no different in volume from controls (caudate, globus pallidus-putamen, dicencephalon, cerebellum, brainstem), and a cluster that was decreased in volume (cerebral cortex, hippocampus-amygdala). The findings highlighted an apparent dissociation between white and gray matter and between cerebral cortex-hippocampus-amygdala and other gray matter structures. The approach was interesting and the results provocative. It would be enlightening to see the same approach applied to imaging data from preschoolers who have autism, and adolescents, adults, and an age-matched, mentally retarded group who have autism, in order to determine age and severity influences on these associations.
Structural imaging of hippocampus, amygdala, and basal ganglia
Many brain structures have been measured in autism, but most have been the subject of only a few small studies, and outcomes have been inconsistent . The hippocampus, amygdala, and basal ganglia are three brain structures that have particular relevance to the symptoms of autism and show at least some consistency in reported findings.
The hippocampus is especially relevant to memory and learning, and neuropsychologic studies of autism have consistently demonstrated abnormalities in memory when use or detection of an organizing strategy was required to support memory . The amygdala has been of particular interest in autism because of its role in emotion and its potential relationship to attachment, emotion dysregulation, affect, and the experience of emotion. Cross-sectional volumetric MR imaging studies of the hippocampus and amygdala have been confined largely to individuals over the age of 6 years, and results from individuals over the age of 12 years have represented all possible outcomes. A cross-sectional study of 7- to 18-year-olds who had ASD (71 males who had ASD: 19 low-functioning autism, 27 high-functioning autism, 25 Asperger’s disorder) and 27 controls reported no difference in TBV, but did report bilateral enlargement of the amygdala in the 7- to 12-year-old low-functioning autism, high-functioning autism, and Asperger’s disorder groups of the same magnitude as that reported by a similar study . The study reported no differences from controls in the 13- to 18-year-old groups. It is also notable that this study found that the ASD groups started with an amygdala volume that was 13% to 17% larger than controls, but did not exhibit the 40% increase in volume seen in the second decade in the normal controls in this and other studies . Hence, developmental dynamics of the amygdala in autism from childhood to adulthood did not follow a normal pattern. The study also reported a 9% to 12% increase in the volume of the left and right hippocampus in the autism groups, which persisted across the age spans (although there was no developmental increase in the size of this structure in the normal control group, as was seen with the amygdala) .
Another study reported a decrease in amygdala-hippocampal volume in 17 individuals aged 7 to 11 years who had autism . Other studies, in individuals over the age of 12 who had autism reported either an increase in amygdala volume , a decrease , or no difference .
Five structural imaging studies reported data on hippocampal volume in subjects over the age of 12 years . Three of these studies found no difference , one study found an absolute and proportional decrease in bilateral hippocampal volume , and one study found an absolute increase proportional to TBV . A sixth study spanned the first 2 decades and found a relative increase in right and left hippocampal volume in individuals who had autism . A more recent study of individuals who had autism, aged 6 to 16 years (mean 9.5 ± 3.3 years), demonstrated a decrease in the right medial posterior hippocampus .
The 13 studies of the hippocampus or amygdala were frequently limited by small samples (8 that had <20 autism subjects), a broad age range, lack of control and subject group matching for males and females, inconsistent anatomic measurement methods across studies, and lack of IQ-matched control groups. All three longitudinal studies of preschoolers with autism have reported increased TBV . One of these studies reported increases in bilateral hippocampal and amygdala volumes, and it seems likely that the remaining two will confirm an increase in amygdala if not hippocampal volume in their preschool samples. The age at which volumes “normalize” will not be clear until the longitudinal studies report data on subsequent time points and limitations of their control groups are addressed at follow-up.
The basal ganglia have been of interest because of their proposed relationship to repetitive behaviors. One study of individuals who had autism (n = 35) reported an increase in caudate volume proportionate to total brain volume that correlated with complex repetitive motor behavior scores from the ADI; this finding was replicated in a second smaller sample (n = 13) who had autism. A second study (n = 15 who had autism) found an increase in the volume of globus pallidus but not caudate, and another (n = 40) found no difference in size compared with normal controls.
Voxel-based morphometry
Most structural imaging studies to date have used region-of-interest manual tracing methods that have low interlaboratory reliability . A few laboratories have switched to automated voxel-based morphometry, which is more sensitive to subtle differences and can be standardized across laboratories.
A comparison MR imaging study of 21 adults who had Asperger’s disorder and 24 age- and gender-matched controls found no differences in regional brain volume differences using manual tracing methods .When voxel-based morphometry methods were applied to these same subjects, however, significant reductions in gray matter volume in frontostriatal and cerebellar regions were identified. In addition, there were an increases in white matter around the basal ganglia and in the left hemisphere. A third finding was that controls exhibited age-related changes in the volume of the cerebral hemispheres and in gray matter, but the Asperger’s group did not. A follow-up study extended this study substantially and demonstrated a consistent pattern of findings across age, severity of autism, race, and ethnicity once sensitive standardized methods were used (eg, voxel-based morphometry) .
This second study of 17 Chinese children who had autism and 17 age-matched typical controls, all with normal IQ scores, mapped regional gray and white matter volumes across the brain . Predicting that volumes of interconnected regions would correlate positively, the study authors analyzed connectivity using correlational analyses. The autism group had significantly less total gray matter volume, but no change in whole brain volume. The subjects also exhibited regional brain reductions within the fronto-striatal gray matter and parietal-temporal cortices. Analyses revealed fewer and less positive cortico-cortical and cortico-subcortical gray matter correlations in the autism group compared with the control group, similar to an early report based on positron emission tomography (PET) in autism . Together, the two studies demonstrate a consistent pattern of imaging alterations in ASD across age, IQ, race, and ethnicity.
MR spectroscopy as a tool for understanding biologic processes
In vivo proton MRS has been used to investigate hypotheses about the cellular histopathology of autism, resulting in an interesting series of results. One study set out to test the hypothesis that the increase in total cerebral volume observed in preschool children who have autism was the consequence of reduced apoptosis, or reduced synaptic pruning early in brain development. This hypothesis predicted increased chemical concentrations and shorter relaxation times caused by decreased mobility of densely packed brain molecules. The study involved 45 3- to 4-year old children who had ASD, 13 children who had typical development, and 15 children who had developmental delay. All groups were age-matched; the ASD and developmentally delayed groups were matched on mental age but not gender, and the ASD and typical development groups were matched on gender but not mental age. The study revealed a generalized pattern of reduced (not increased) brain chemical concentrations and prolonged (not shortened) transverse (T 2 ) relaxation times. These results did not support reduced apoptosis or pruning as the mechanism for increased brain volume. Rather, the findings were more consistent with reports of increased minicolumns with reduced cellularity, as described in the many regions of cerebral cortex by Casanova and colleagues . Because the study was unable to determine whether gray or white matter was specifically affected, however, two additional studies were completed .
In a subsequent analysis of the data from these same 3- to 4-year old cohorts , using cerebral volume as a covariate, the study found a distinct pattern of specific chemical alterations in gray matter in ASD that were distinct from both the typical development and developmental delay groups. The ASD group exhibited decreased gray matter concentrations of choline-containing compounds (Cho), creatine and phosphocreatine (Cre), n-acetylaspartate (NAA), and myoinositol (mI), and prolonged Cho T 2 relaxation time compared with the typical development group. Compared with the developmentally delayed group, the ASD group had decreased gray matter, Cho, and mI concentrations. The ASD and developmentally delayed children exhibited the same pattern of decreased NAA and mI concentrations in white matter, suggesting that the white matter findings were nonspecific effects of developmental delay . These findings provided evidence of abnormalities in cortical gray matter early in life in autism that were distinct from abnormalities in children who have nonautism developmental delay. The findings suggested decreased cellularity or density. Interestingly, because synaptosomes express high levels of chemicals by MRS and shorter T 2 times, these findings were consistent with a reduction in dendritic arborization and accompanying decreased synaptosome density.
A third study of these 3- and 4-year-old children examined T 2 relaxation in cortical gray matter, proposing that if enlarged cerebral volume were caused by an acceleration of normal brain growth, then gray and white matter T 2 would be decreased in the ASD children relative to the typical development children, reflecting a more advanced stage of brain development, and the developmentally delayed children would be expected to have prolonged T 2 consistent with more immature development. The study revealed prolonged whole-brain cortical gray matter T 2 in the ASD children, but no differences in whole-brain white matter T 2 compared with the typical development children. The developmentally delayed children exhibited prolonged T 2 in both gray and white matter . These findings did not support an acceleration of the normal brain maturation processes as the cause of the early increase in brain volume in autism. Furthermore, these findings suggested that the triggering or primary event might have been neuronal (eg, in cerebral cortex), and that the white matter changes were expressions or consequences of that event.
A recently published study provided confirmation of cortical involvement and also implicated glutamatergic mechanisms in autism. Using 1H MRS methods similar to those of Friedman and colleagues , DeVito and colleagues used partial volume regression (all valid voxels used for calculating metabolite concentrations) in a pilot study of 26 boys aged 6 to 17 years who had autism, with IQs above 70. These were group-matched to 29 typical 6- to 16-year-old boys. Using a multivariate linear regression model to determine if abnormalities were localized to specific lobes of the brain, the autism group exhibited reduced NAA and glutamine-glutamate (Glx) levels in cortical gray matter, and no differences in metabolite levels in overall cortical white matter. Metabolite levels were examined in frontal, temporal, and occipital gray matter, cerebral white matter, and cerebellum. These analyses revealed lower NAA concentrations in frontal and occipital gray matter, and a trend for reductions in NAA in temporal cortex (0.07), cerebral white matter (0.06) and cerebellum (0.06). Of particular interest, there were lower G1x levels in frontal and occipital gray matter and in cerebellum, and a trend for reduced Glx in temporal lobe (0.07). Also of note, the controls had a strong negative correlation between age and gray matter and G1x, but the autism subjects did not. Similarly, controls showed significant negative correlations between age and concentrations of frontal, temporal, occipital and cerebellar NAA, but autism subjects only showed negative correlations between age and NAA in cerebellum. The widespread cortical gray matter reduction in NAA concentrations in this 6- to 17-year-old group of individuals who had autism supported continuation of neuronal dysfunction or reduction in neuronal number past the preschool age, despite “normalization” of brain volume . This finding provided further support for the involvement of cerebral cortex in autism. This study was also important for the attention it drew to abnormalities of the glutamatergic system, although others had hypothesized a role for disturbances in this system in the developmental neurobiology of autism , and some groups had provided evidence of its involvement .
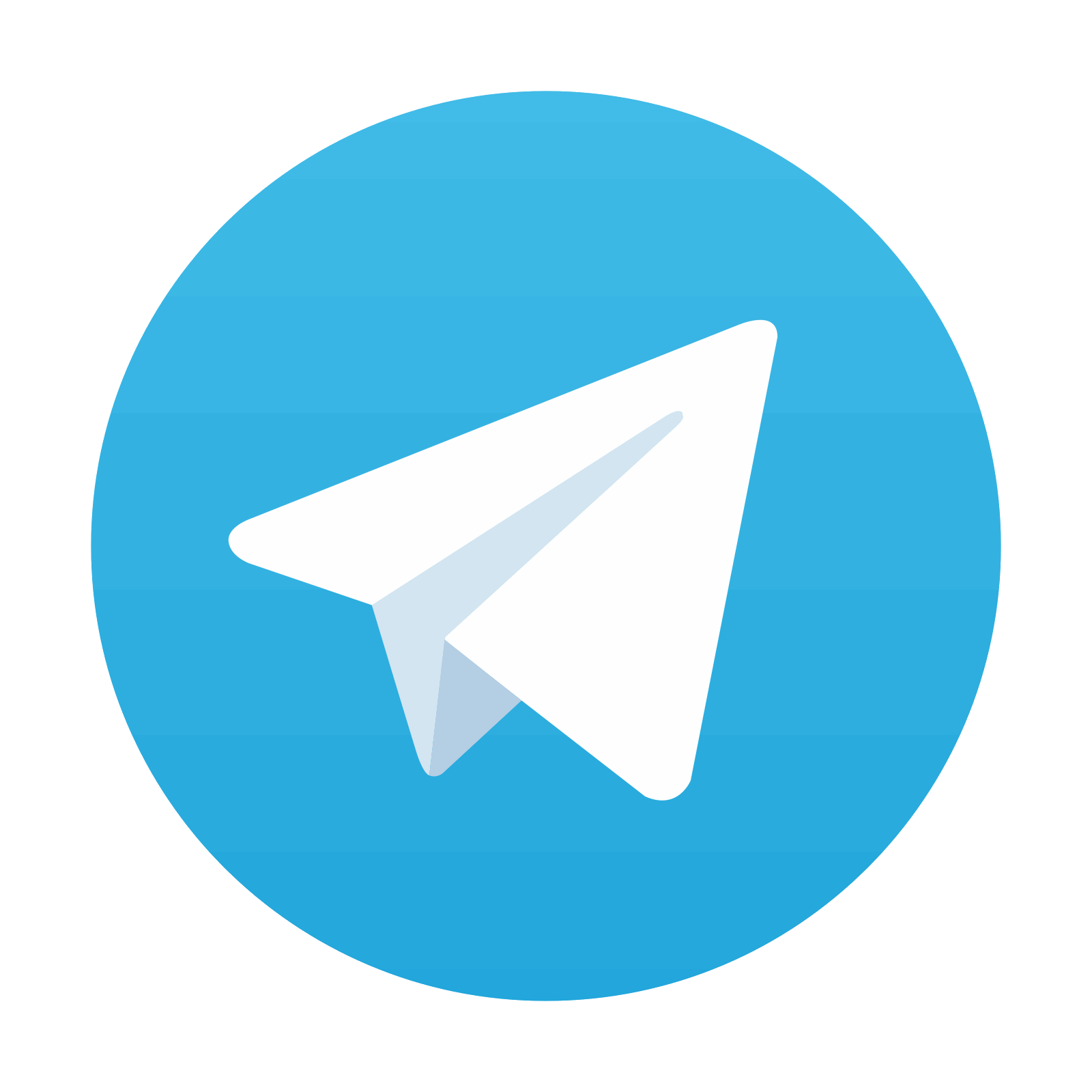
Stay updated, free articles. Join our Telegram channel

Full access? Get Clinical Tree
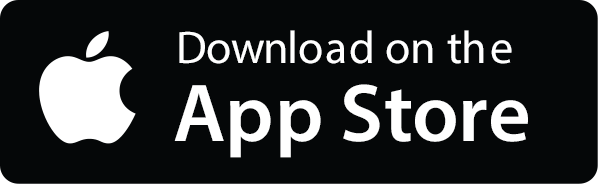
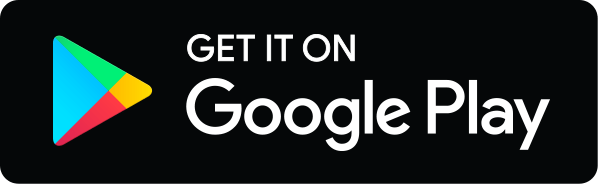