Fig. 23.1
Haptic devices for interactive manipulation of anatomical structures reconstructed from three-dimensional (3D) datasets. The operator can interactively rotate and dissect a virtual specimen to study the anatomy in detail. The corresponding forces are felt through the haptic devices (a). A virtual patient in the operating theatre, with a heart reconstructed from a 3D MRI dataset (b)
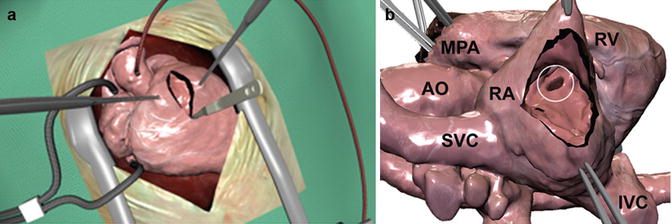
Fig. 23.2
Magnified view of the virtual patient from Fig. 23.1 in a full rendering of the surgical setting (a). Only the heart is visualized in (b) depicting a ventricular septal defect (VSD, circle). The heart was reconstructed from a 3D MRI dataset. It can be manipulated by lifting, rotation, dissection, insertion of a virtual patch, etc. using the devices displayed in Fig. 23.1. The corresponding forces to the virtual manipulations, such as performing a myocardial incision and traction of myocardial tissue using a forceps, are transmitted to the user via the haptic devices (Fig. 23.1a). AO aorta, IVC inferior vena cava, MPA main pulmonary artery, RA right atrium, RV right ventricle, SVC superior vena cava
Planning of interventions in patients with CHD, in particular surgical procedures, can be very difficult. Often surgical decisions are based on previous experience and therefore the result is very often operator dependent. Patient-specific virtual surgery simulation could improve the surgeon’s or interventionalist’s preoperative planning and training opportunities, therefore reducing the risk of cardiothoracic interventions and improve outcome. Using simulation, the optimal interventional strategy can be planned in advance and different approaches can be discussed with colleagues from other institutions with different grades of experience. The 3D model is a useful communication tool that enables the pediatric cardiac surgeon or interventionalist to discuss interventional strategies. Instead of discussing treatment options purely based on 2D imaging and 3D renderings of the extracardiac anatomy, patient-specific virtual surgery provides the surgeon with a useful tool to communicate the surgical view of the intra- and extracardiac morphology and allows testing of different strategies to repair a cardiovascular defect.
So far heart specimens were essential to understand the various complex pathologies in patients with congenital heart disease. Studies in the pathology laboratory are only possible in a few institutions worldwide, and the number of these rare specimens is very limited. A combination of virtual and physical reproduction of anatomical specimens in conjunction with demonstration of modern imaging techniques could be ideal for training in the clinical environment. As outlined in Fig. 23.3, virtual reconstruction and physical reproduction using rapid prototyping is a very interesting concept in this respect. Virtual cardiotomy could be a cost-effective supplementary technique to rapid prototyping. Different types of incisions and interventional procedures can be tested and demonstrated independently of the level of experience of the operator and without any risk for the patient. As the models are patient specific, personalized treatment strategies can be developed using this technology.
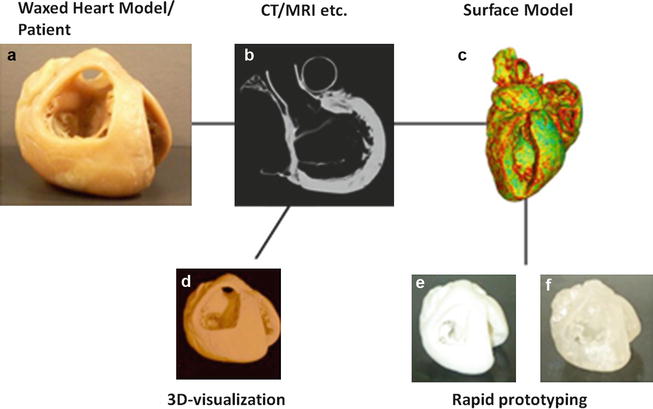
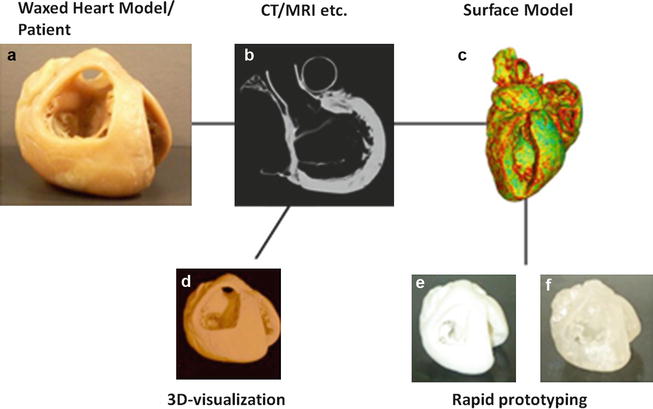
Fig. 23.3
A schematic overview of the production process from a specimen (a) via imaging (b) to virtual reconstruction (d) and physical reproduction using rapid prototyping (e, f) after creation of a STL file (c). Computed tomography was used as the imaging modality in this example, but other 3D imaging techniques can be used in the production process as well
From Imaging to 3D Modeling
As a prerequisite of virtual surgery, three steps are required:
1.
Image acquisition
2.
Image segmentation
3.
Three-dimensional modeling
A visual illustration of these three steps is provided in Fig. 23.3 and a dedicated section for each step is provided below. The description is intended as an overview of one possible approach to obtain a model suitable for virtual surgery.
It is important to understand that each of these steps is some kind of interpretation of the image data and therefore some errors may be introduced in comparison to the original anatomical structure. These errors can be minimized by improved image quality, e.g., by raising image resolution and improving contrast. In case of virtual surgery, which is performed to predict exact results of a surgical procedure, these errors must be considered when evaluating surgical outcome. Because of this surgeons and interventionalists should be critical of simulation results and be prepared for variations during the actual procedure.
Image Acquisition
The initial step towards obtaining an accurate model for simulation of virtual surgery is acquisition of an image dataset with high resolution and contrast for optimal 3D modeling of the morphology of the congenital heart defect. Frequently an end-diastolic scan is preferred from a surgeon’s point of view, as it is closest to the state of the heart on the heart-lung machine [4]. However, the dynamic component of a beating heart is not displayed with this approach. Novel 3D techniques allow acquisition of at least two cardiac phases within one cardiac cycle (end systole and mid diastole) [5]. A complete time-resolved dataset of the cardiac cycle would be ideal [6]. Each individual phase could then be segmented and the results of an intervention could be modeled on a beating heart model.
The present chapter focuses on the concept of virtual interventions in open-heart surgery. Therefore it is assumed that a single-phase three-dimensional (3D) scan in end diastole should be sufficient. Currently multidetector computed tomography (MDCT) or magnetic resonance imaging (MRI) is used for a 3D virtual surgery dataset. MRI as a radiation-free and noninvasive method is most commonly used in patients with CHD in clinical routine. Therefore our examples in this book chapter are based on MRI datasets.
3D MRI
A range of publications is dedicated to 3D MRI. Early research focused on delineating the vasculature structures using contrast-enhanced imaging [7, 8]. As data are usually acquired within one breath hold, ECG triggering is not used to shorten data acquisition time. Therefore intracardiac structures are not imaged adequately. Respiratory-gated and ECG-triggered sequences allow the acquisition of whole-heart MRI datasets suitable for segmental morphological analysis [5, 9]. It was initially evaluated in adolescents and adults with congenital heart disease [4]. After further technical developments, now intracardiac structures in infants and children can be imaged and used for 3D modeling [5, 10]. Recently new intravascular contrast agents have enabled contrast-enhanced imaging triggered to the cardiac cycle. These improvements enable 3D MRI to be acquired in all age groups in clinically acceptable time frames [11, 12]. Figure 23.4a illustrates the current standard image quality obtainable for the dataset underlying the 3D reconstruction demonstrated in Figs. 23.1 and 23.2.
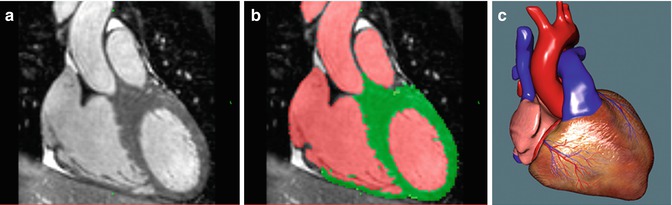
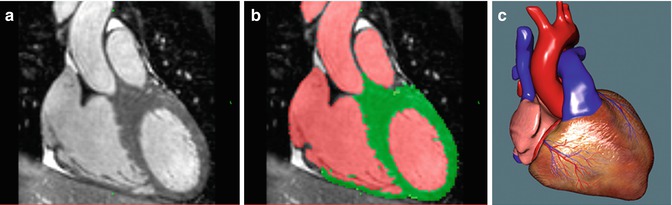
Fig. 23.4
A three-step process to create a model for virtual surgery is shown: 3D imaging (a), semiautomatic segmentation (b), and 3D reconstruction of a virtual model for visualization and simulation (c)
Image Segmentation
Tissue and blood pool need to be segmented to create suitable models for virtual surgery. With the current sequences used, the vessel walls are indistinguishable from the blood lumen and surrounding tissue. Therefore the myocardium and blood pool are segmented. Vessel walls are created by software unless the blood pool is already bordered by the myocardium.
It is out of the scope of this chapter to discuss various segmentation algorithms. For the present work a marker-based segmentation algorithm was applied [1]. The user inserts a number of colored markers in the blood pool, myocardium, and background, respectively. One color is dedicated for each category. From this input the software computes and visualizes a corresponding segmentation in real time. This procedure can be carried out in just a few minutes, assuming a reasonable image quality has been obtained. Unfortunately it is usually more time consuming to subsequently verify and manually correct residual segmentation errors. A previous study showed that about 1 h for segmentation was required for a suitable model for surgical simulation [3]. Improvements of segmentation software, image contrast, and resolution will reduce the time for creating models for virtual surgery. Figure 23.4b shows a segmented slice corresponding to the non-segmented slice seen on the left (Fig. 23.4a
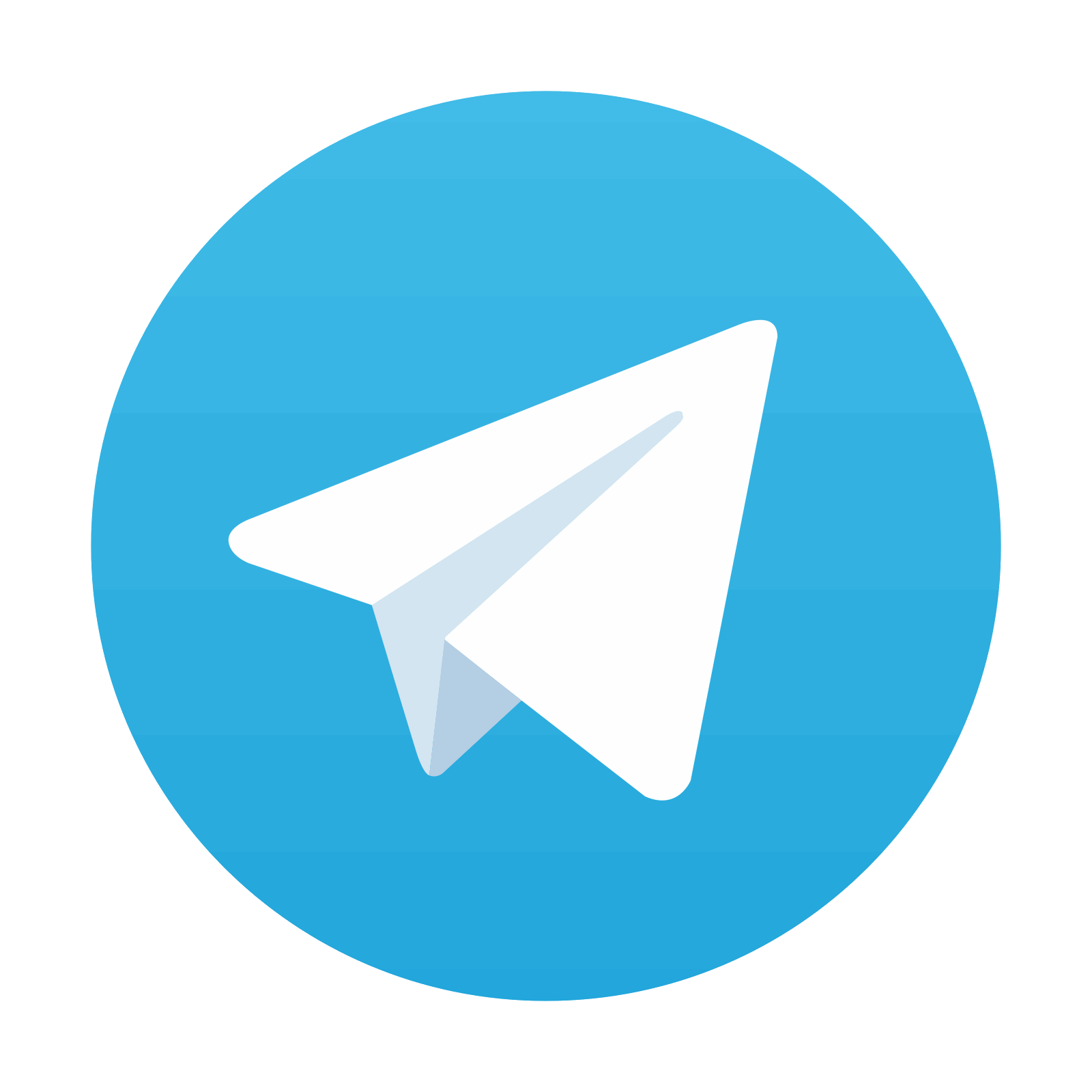
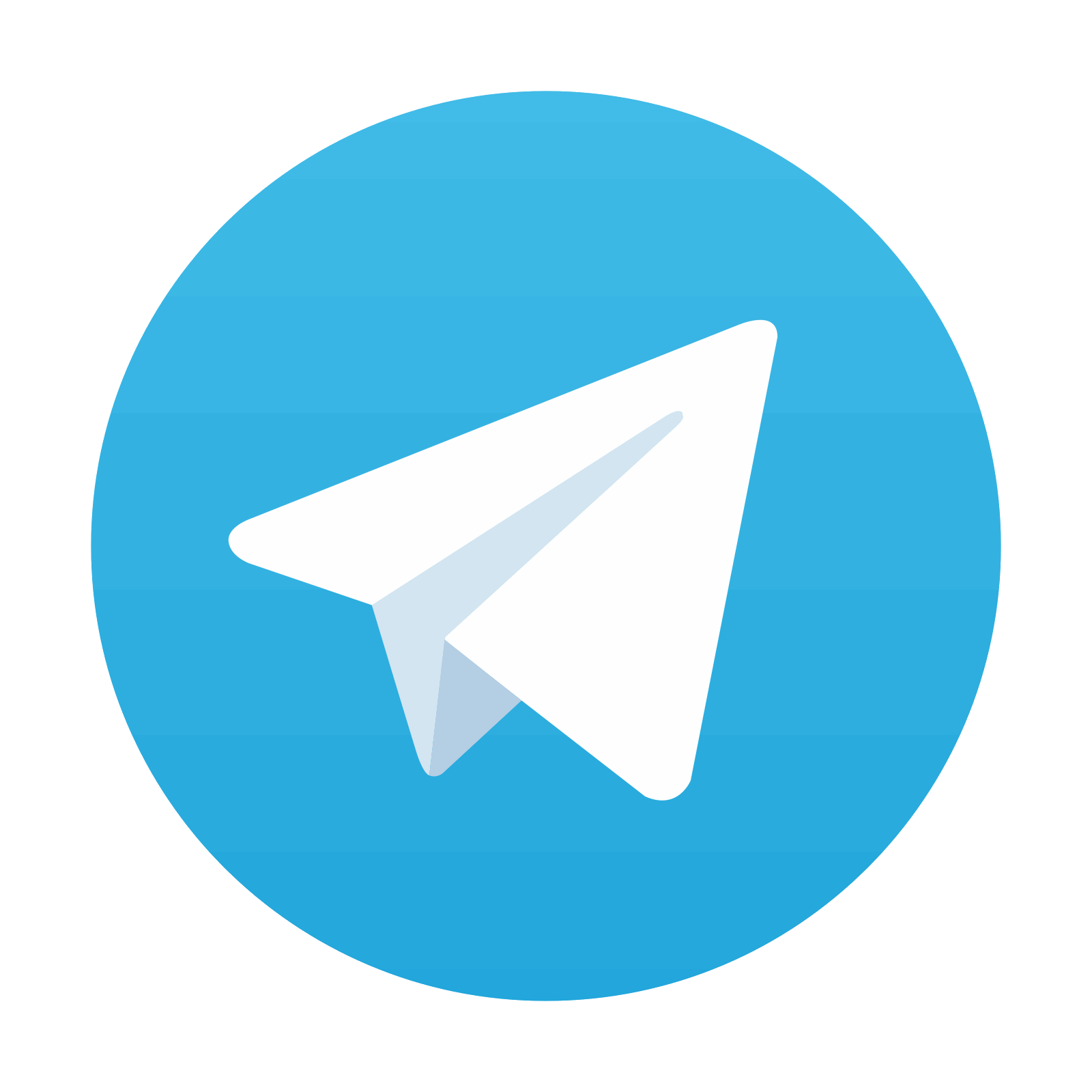
Stay updated, free articles. Join our Telegram channel

Full access? Get Clinical Tree
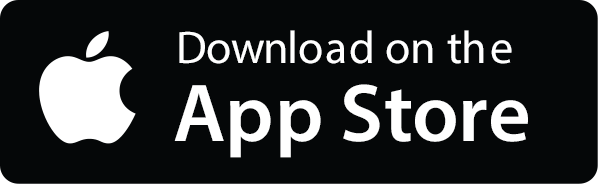
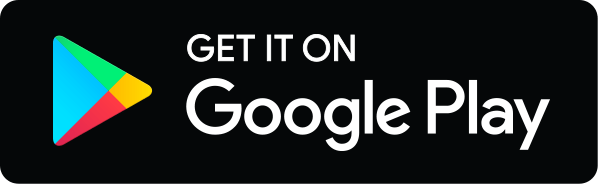