Fig. 1
Intraperitoneal carcinomatosis with ascites 5 weeks after i.p. injection of MDA-MB435S cells expressing mutant E-cadherin into nude mice. a Section of an i.p. nodule (H&E staining) with tumour cells on the serosa (arrowhead) and invasive tumour cells in the mesenteric adipose tissue (arrow). b Single tumour cells (arrows) and small cell clusters (arrowhead) in the ascites fluid showing a non-specific inflammatory reaction (modified May-Grünwald-Giemsa staining)
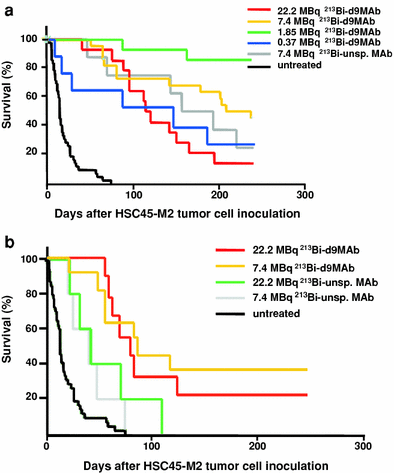
Fig. 2
Survival of nude mice following therapy with 213Bi-d9MAb a at day 1 or b at day 8 after intraperitoneal inoculation of HSC45-M2 gastric cancer cells. Mice were injected intraperitoneally with saline (untreated), specific 213Bi-d9MAb (0.37, 1.85, 7.4 or 22.2 MBq) or unspecific 213Bi-d8MAb (7.4 or 22.2 MBq)
To improve therapeutic efficacy in advanced stage peritoneal carcinomatosis fractionated 213Bi-d9MAb therapy turned out as a promising strategy. Double injections of 0.74 MBq of 213Bi-d9MAb on days 8 and 15 after tumour cell inoculation resulted in a higher therapeutic efficacy than a single injection of 1.48 MBq on day 8 (Bloechl et al. 2005).
Moreover, tumour regression after 213Bi-d9MAb therapy could be visualised non-invasively via bioluminescence imaging. For that purpose, mice were inoculated with HSC45-M2 cells stably transfected with the gene coding for firefly luciferase. As demonstrated after luciferin injection using a cooled CCD camera, 213Bi therapy on day 8 totally eliminated small tumour nodules and reduced the size of larger nodules (Buchhorn et al. 2007) (Fig. 3).
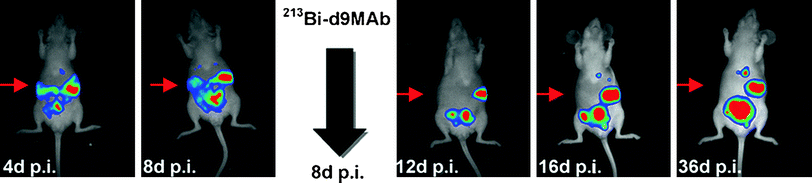
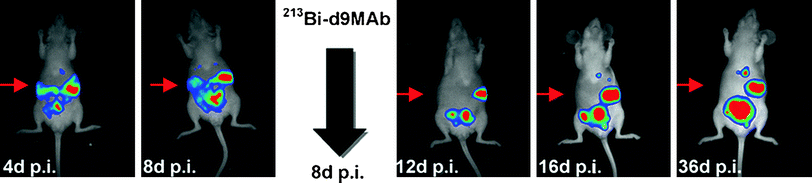
Fig. 3
Non-invasive monitoring of tumour development via bioluminescence imaging 4, 8, 12, 16 and 36 days after intraperitoneal inoculation of HSC45-M2-luc cells. At day 8 after tumour cell inoculation the mouse was treated intraperitoneally with 2.7 MBq 213Bi-d9MAb. Elimination of small tumour nodules as a result of 213Bi-d9MAb therapy is marked by red arrows
Almost 90 % of tumour-free animals that had been injected intraperitoneally with 7.4 MBq of 213Bi-d9MAb survived longer than 300 days. Following autopsy none of these mice showed any signs of organ toxicity. Therefore, activities of up to 7.4 MBq of 213Bi-d9MAb are thought to cause no toxic side effects in mice. Kidney damage was observed only after injection of 22.2 MBq of 213Bi-d9MAb. However, this activity is far beyond 1.85 MBq that proved to be the activity with the best therapeutic efficacy (Beck et al. 2007).
In contrast, treatment with therapeutically effective activities of the β-emitter 177Lu coupled to d9MAb was not free of toxic side effects. Following treatment with 7.4 MBq of 177Lu-d9MAb 1 day after i.p. inoculation of HSC45-M2 cells 90 % of the animals survived longer than 250 days. However, in some animals lymphoblastic lymphoma, proliferative glomerulonephritis and hepatocarcinoma developed but were not observed after treatment with 213Bi-d9MAb at comparable therapeutic efficacy (Seidl et al. 2011). Therefore, 213Bi-d9MAb should be preferred for the treatment of peritoneal carcinomatosis.
Though urgently needed, initiation of a clinical trial is hampered by an inadequate number of patients at present. As mentioned above, only 20 % of diffuse-type gastric cancer patients could be included into such a study due to the restricted expression of mutant d9-E-cadherin targeted by 213Bi-d9MAb.
4 Intraperitoneal α-Particle Radioimmunotherapy of Ovarian Cancer
4.1 First Phase 1 Clinical Study
Radioimmunotherapy of ovarian cancer with β-emitter immunoconjugates such as 90Y-HMFG1 showed disappointing results in a randomised phase III study in terms of survival or time to relapse (Verheijen et al. 2006). The therapeutic potential of 211At-MX35 F(ab’)2, however, has been demonstrated in an intraperitoneal ovarian cancer mouse model (see Sect. 3.1). Therefore, a clinical phase I study was initiated enrolling nine women in complete remission after second-line chemotherapy for recurrent ovarian carcinoma (Andersson et al. 2009). MX35 recognises the sodium-dependent phosphate transport protein 2b (NaPi2b) which is overexpressed in more than 90 % of human ovarian epithelial cancers (Yin et al. 2008). Moreover, it has been shown that 125I and 131I-labelled MX35 and MX35 F(ab’)2 conjugates specifically localise to tumours of patients with intraperitoneal growth of ovarian cancer (Finstad et al. 1997; Rubin et al. 1993).
The aim of the phase I study was to determine the pharmacokinetics for assessing absorbed dose to normal tissues and investigating toxicity. For that purpose patients without macroscopic tumour growth or major adhesions, as verified by laparoscopy, were infused with 211At-MX35 F(ab’)2 (22.4–101 MBq/L) via a peritoneal catheter. Biodistribution of 211At-MX35 F(ab’)2 was monitored regularly via γ-camera scans, collection of samples of blood, urine and peritoneal fluid and toxicity was evaluated by haematology and by renal and thyroid function followed for a median of 20 months. The cumulative urinary excretion of 211At was 40 kBq/(MBq/L) at 24 h. The decay corrected 211At activity concentration in the peritoneal fluid decreased to 50 % of the initial activity concentration at 24 h. In contrast, the activity concentration in the serum increased to 6 % at 45 h and in the thyroid to 127 % (±63 %) at 20 h without blocking and less than 20 % with blocking. In all other organs no uptake of 211At could be observed. The estimated absorbed doses to the unblocked and blocked thyroid were 24.7 ± 11.1 mGy/(MBq/L) and 1.4 ± 1.6 mGy/(MBq/L), respectively. The estimated absorbed dose to the peritoneum bearing tumour tissue was 15.6 ± 1.0 mGy/(MBq/L), thus reaching therapeutically effective doses. The absorbed doses to the red bone marrow and to the urinary bladder wall were comparatively low. No adverse effects could be observed in all laboratory parameters analysed. Therefore, the results of this first clinical phase 1 study strongly suggest that intraperitoneal 211At-radioimmunotherapy of ovarian cancer patients will be efficient without significant toxicity (Andersson et al. 2009).
5 Future Prospects
Application of α-emitter immunoconjugates for targeted therapy of peritoneal carcinomas is promising especially in eradication of single disseminated tumour cells or small tumour cell clusters due to the short range and high linear energy transfer of α-particles. Nevertheless, α-emitters play still a minor role for use in radioimmunotherapy compared to β-emitters. This is particularly due to the good commercial availability of β-emitters such as 131I, 171Lu, 90Y or 186Re. In contrast, α-emitters are not commercially available and are usually provided in small activity amounts only by a few institutes worldwide in the course of scientific co-operations. The activity of 225Ac/213Bi that can be prepared from 229Th sources amounts to approximately 3.1 GBq per month worldwide. Future production of 225Ac in cyclotron facilities will possibly increase the available activity per month by a factor of ten (Apostolidis et al. 2005b). For elevation of the 211At production new cyclotron facilities with adequate acceleration energies are needed. In the Accelerator for Research in Radiochemistry and Oncology at Nantes Atlantic (ARRONAX) large-scale production (several GBq) of 211At will start by the end of 2012 (Haddad et al. 2011). Another aim is to improve stability of α-emitter conjugates by use of appropriate chelating agents, especially for α-emitters that emit multiple α-particles such as 225Ac. With regard to the future clinical application of α-emitter conjugates also reliable models in terms of pharmacokinetics and dosimetry are needed, including the aspects of cellular microdosimetry (Chouin and Bardiès 2011; Sgouros et al. 2011).
Despite these obstacles that impair routine application of α-emitter conjugates in targeted therapy, it appears worthwhile to follow the pursued path. The cytotoxic potential of α-emitters is unique also toward hypoxic tumour cells and cells that are resistant to chemotherapy. Therefore, α-emitters are indispensable with regard to optimisation of strategies for tumour therapy.
References
Andersson H, Palm S, Lindegren S, Bäck T, Jacobsson L, Leser G, Horvath G (2001) Comparison of the therapeutic efficacy of 211At- and 131I-labelled monoclonal antibody MOv18 in nude mice with intraperitoneal growth of human ovarian cancer. Anticancer Res 21:409–412PubMed
Andersson H, Cederkrantz E, Bäck T, Divgi C, Elgqvist J, Himmelman J, Horvath G, Jacobsson L, Jensen H, Lindegren S, Palm S, Hultborn R (2009) Intraperitoneal alpha-particle radioimmunotherapy of ovarian cancer patients: pharmacokinetics and dosimetry of (211)At-MX35 F(ab’)2—a phase I study. J Nucl Med 50:1153–1160PubMedCrossRef
Bloechl S, Beck R, Seidl C, Morgenstern A, Schwaiger M, Senekowitsch-Schmidtke R (2005) Fractionated locoregional low-dose radioimmunotherapy improves survival in a mouse model of diffuse-type gastric cancer using a 213Bi-conjugated monoclonal antibody. Clin Cancer Res 11(19 Pt 2):7070s–7074sPubMedCrossRef
Borchardt PE, Quadri SM, Freedman RS, Vriesendorp HM (2000) Intraperitoneal radioimmunotherapy with human monoclonal IGM in nude mice with peritoneal carcinomatosis. Cancer Biother Radiopharm 15:53–64PubMedCrossRef
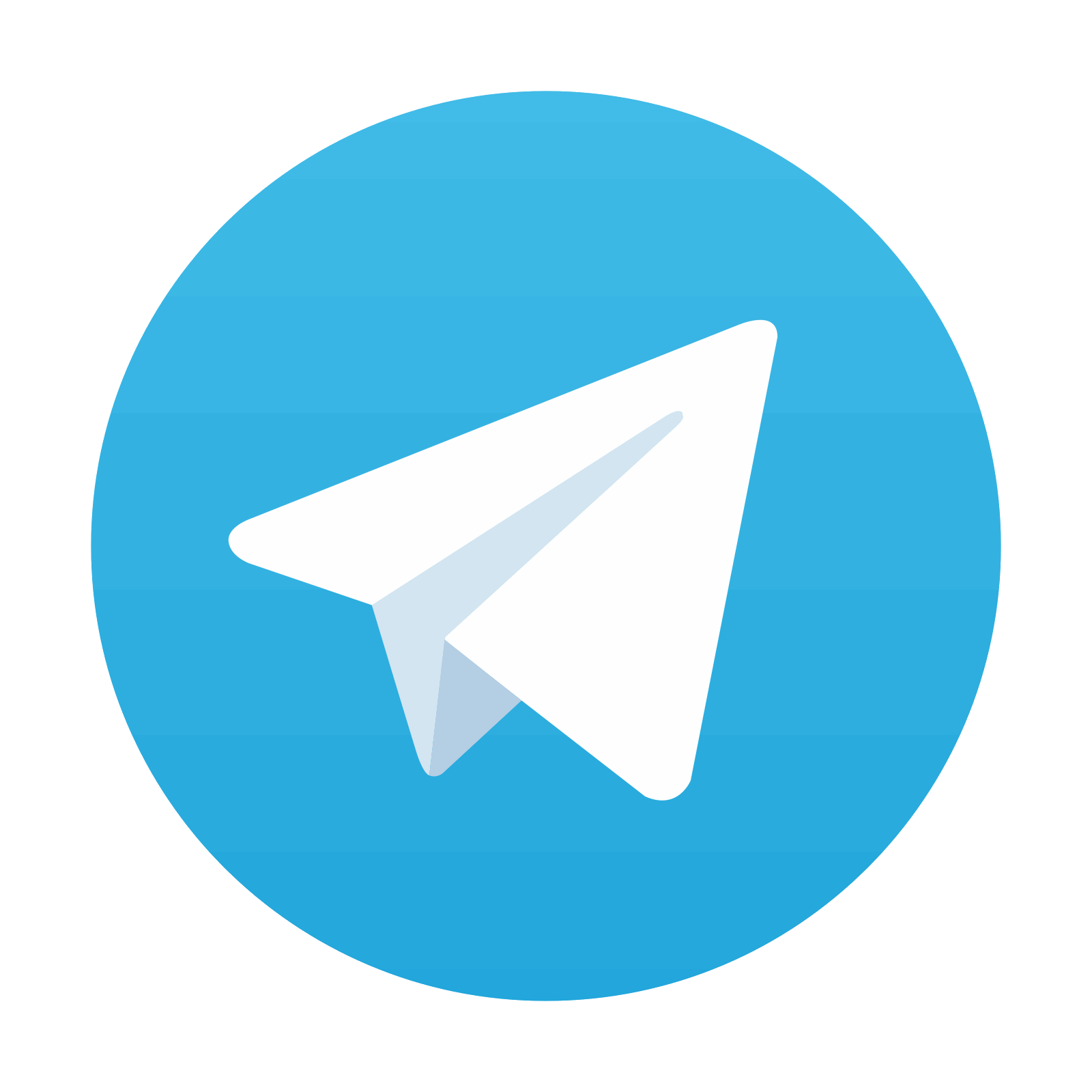
Stay updated, free articles. Join our Telegram channel

Full access? Get Clinical Tree
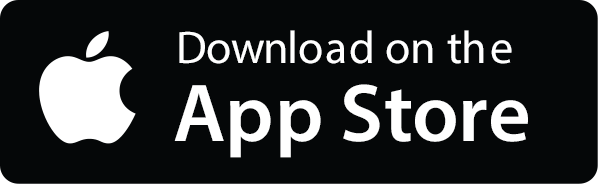
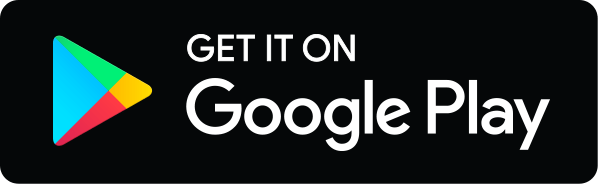