An Overview of Magnetic Resonance Imaging
Objectives
At the completion of this chapter, the student should be able to do the following:
Key Terms
Historical Trail
If someone wanted to make an image of a patient 150 years ago, what could have been done? Actually, not much. At that time, only photography or hand-drawn images were available. Both types of such images use the narrow band of the electromagnetic spectrum called the visible light region (Figure 1-1).
Electromagnetic radiation can be characterized by any one of three parameters: energy, wavelength, and phase. The frequency is sometimes used to describe the wave character of electromagnetic radiation but it is basically equivalent to the wavelength since the wavelength is just the speed divided by the frequency and the speed of light is a constant, c (~3 × 108 m/s). Although the only electromagnetic radiation that we can sense directly is visible light, we know that the range of electromagnetic radiation extends over many orders of magnitude and different types of radiation.
How does photography work? Visible light reflects off an object, and the reflected light is detected by a medium that is sensitive to that kind of radiation—a photographic emulsion or the retina. Therefore, a photograph is made with reflected electromagnetic radiation and a suitable receptor.
Nineteenth-century physicists studying visible light detailed its wavelike properties according to how it interacted with matter (i.e., reflection, diffraction, and refraction). Consequently, visible light has always been characterized by its wavelength.
When Wilhelm Conrad Roentgen discovered x-rays in 1895, there was suddenly another narrow region of the electromagnetic spectrum from which medical images could be made. In 1901 Roentgen received the first Nobel Prize in physics for his discovery. One reason Roentgen received this award was that within 6 months, he had conducted a number of cleverly designed experiments and described x-rays much as they are known today.
Some of his experiments indicated that this “x-light” interacted as a particle, not as a wave. As a result, x-ray emissions are identified according to their energy. Although we commonly refer to kilovolt peak (kVp), it is more accurate to use kiloelectron volt (keV) to identify x-radiation.
How is an x-ray image made? Electromagnetic radiation (i.e., an x-ray beam) shines on a patient. Some of the radiation is absorbed; some of it is transmitted through the patient to an image receptor. This results in a shadow gram-like image from the transmission of electromagnetic radiation.
During the latter part of the nineteenth century, after Thomas Edison’s early work, engineers and physicists worked to develop radio communications. Electrons must oscillate in a conductor to create a radio emission. This requires the construction of an electronic circuit called an oscillator. The oscillator is the basis for radioelectronics.
The electromagnetic radiation produced by the oscillator is called a radiofrequency (RF) emission. Physicists identify this radiation according to the frequency of oscillation.
Commercial broadcasts such as AM radio, FM radio, and television (TV) are similarly identified. The AM RF band ranges from 540 to 1640 kHz, and the FM RF band ranges from 88 to 108 MHz. TV broadcast ranges from 54 to 806 MHz, which includes both VHF and UHF.
Use of the RF region of the electromagnetic spectrum to produce an image is especially spectacular. It is based on an analytical procedure called nuclear magnetic resonance (NMR) and was first called nuclear magnetic resonance imaging (NMRI). Some of the leaders in radiology were concerned about using the word nuclear around patients, since NMRI really didn’t involve any kind of ionizing radiation. As a result, that word was dropped early in the development of this imaging process, and we are left with magnetic resonance imaging (MRI).
How is a magnetic resonance (MR) image made? For a visible image, radiation is reflected from the body. For an x-ray image, radiation is transmitted through the body. For an MR image, the patient is stimulated so that electromagnetic radiation is emitted from the body. Through the use of some clever methods, the emitted signal is then detected, interpreted, and used to produce an image (Figure 1-2).
Felix Bloch
Magnetic fields associated with atoms and nuclei were first described in the 1930s. Otto Stern and Isador Rabi each received a Nobel Prize in physics for their work on atomic and nuclear magnetism. Rabi coined the term nuclear magnetic resonance.
In 1946 Felix Bloch at Stanford and Edward Purcell at Harvard independently described NMR in a solid. They shared the 1952 Nobel Prize in physics for this work. Bloch continued extensive studies with the NMR of water, thereby laying the groundwork for later developments that led to MRI.
Bloch is to MRI what Roentgen is to x-ray imaging, and Bloch is known as the father of MRI. As a theoretical physicist, Bloch proposed some novel properties for the atomic nucleus, including that the nucleus behaves like a small magnet. He described this nuclear magnetism by what are now called the Bloch equations (see Appendix A).
Bloch’s equations explain that a nucleus, because it spins on an imaginary axis, has an associated magnetic field. This field is called a magnetic moment. Nucleons that have charge (e.g., protons) and that spin have even stronger magnetic fields.
Experimental verification for the Bloch equations did not come until the early 1950s. By 1960 several companies were producing analytical instruments called NMR spectrometers. During the 1960s and 1970s, NMR spectroscopy (see Chapter 8) became widely used in academic and industrial chemistry research. Such use of NMR enabled investigators to determine the molecular configuration of a material from the analysis of its NMR spectrum.
Damadian and Lauterbur
In the late 1960s engineer-physician Raymond Damadian, while working with NMR spectroscopy, showed that malignant tissue has a different NMR spectrum from normal tissue. Furthermore, he showed that the parameters associated with NMR (i.e., proton density, spin-lattice relaxation time, and spin-spin relaxation time) differ between normal and malignant tissue. Damadian produced a crude NMR image of a rat tumor in 1974 and the first body image in 1976. That image took almost 4 hours to produce.
At this same time Paul Lauterbur, an NMR chemist at State University of New York in Stony Brook, developed the first imaging method using NMR that is similar to what is used today. He called this method zeugmatography, which was sort of Greek for saying that this imaging method requires a whole bunch of magnetic fields whizzing and buzzing around. Meanwhile in Nottingham, England, Peter Mansfield, a solid-state physicist, was engaged in similar research and eventually developed the echo-planar MRI method that is used for functional MR neuroimaging today. In 2003 Lauterbur and Mansfield shared the Nobel Prize in physiology and medicine “for their discoveries concerning magnetic resonance imaging.”
In fact, a large number of scientists made significant contributions to the early development of MRI, among them James Hutchinson and William A. Edelstein at the University of Aberdeen, David Hoult at Oxford University, Ian Young at EMI Laboratories, and Waldo Hinshaw and E. Raymond Andrew, both at the University of Nottingham. These gentlemen have all received numerous high scientific and engineering honors for their contributions to the field.
Why Magnetic Resonance Imaging?
When a plain radiograph of the abdomen is placed on a view box for interpretation, what can be seen? Not much. The image is gray and flat and shows little detail. A conventional tomogram or an angiogram may be done to improve image contrast.
Contrast Resolution
If such an image is unsatisfactory, what else can be done? A computed tomography (CT) image can be requested. The principal advantage of CT imaging over radiographic imaging is superior contrast resolution, the ability to image differences among low-contrast tissues. Contrast resolution allows visualization of soft tissue with similar characteristics, such as liver–spleen or white matter–gray matter.
The spatial resolution of a CT image is worse than that of radiographic imaging because it is digital and limited by pixel size. Likewise, the spatial resolution of MRI is worse than that of radiography. However, the contrast resolution is even better with MRI than with CT.
Spatial Resolution
Spatial resolution refers to the ability to identify an object, usually a small, dense object like a metal fragment or microcalcification, as separate and distinct from another object. Table 1-1 shows representative values of spatial resolution and contrast resolution for various medical imaging devices.
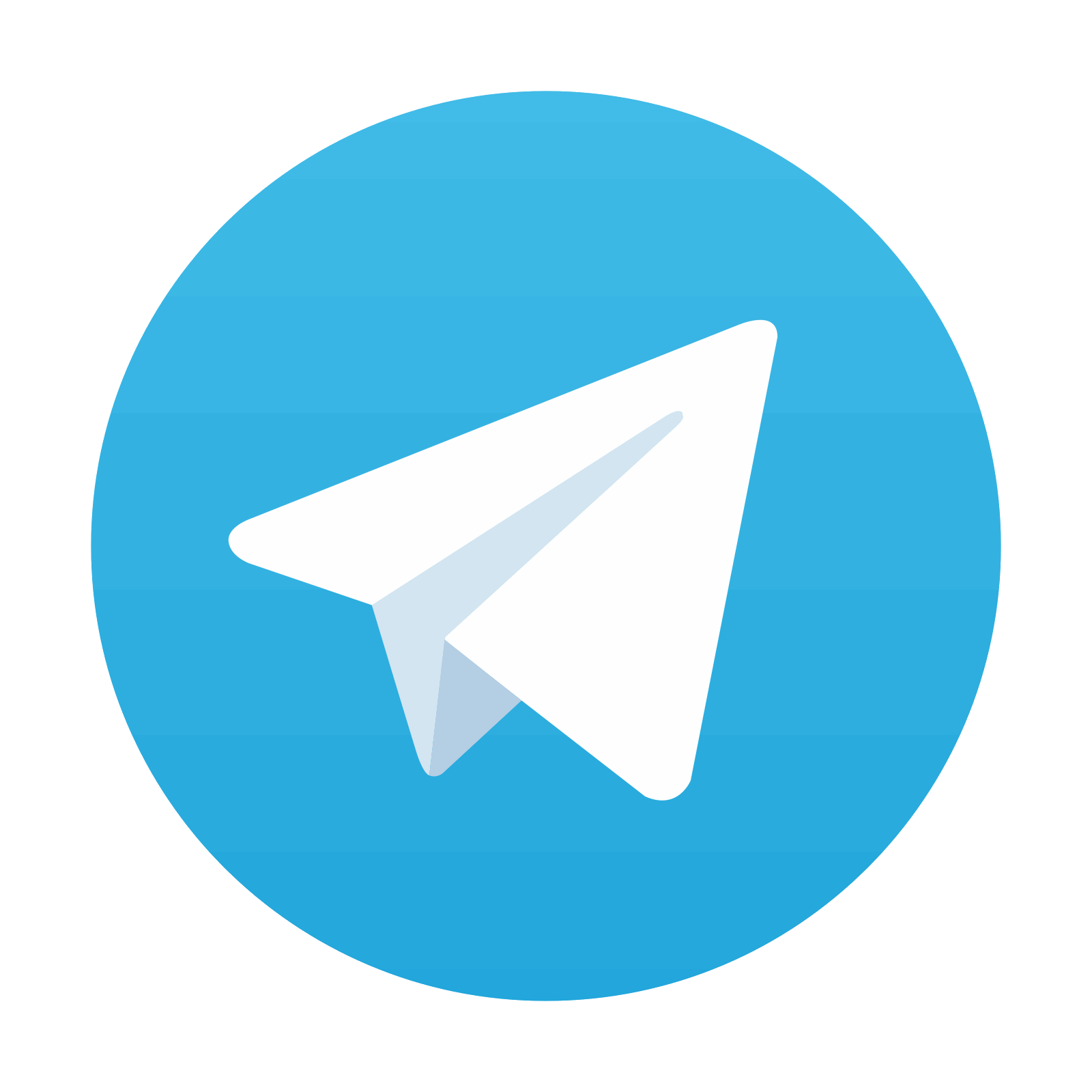
Stay updated, free articles. Join our Telegram channel

Full access? Get Clinical Tree
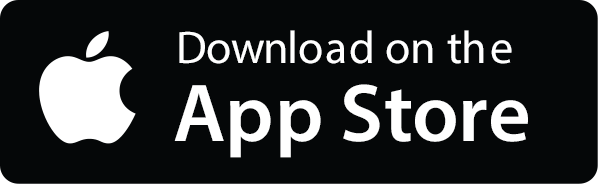
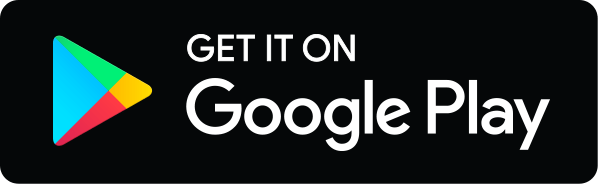