Fig. 6.1
Measurement of cortical activation during walking using NIRS system. a Subject performing a locomotor task on the treadmill. b T Cortical activation map based on the OxyHb signal changes during gait on the treadmill at 3 km/h. Broad cortical area is activated in acceleration phase, whereas only medial aspect of motor-related cortices are activated in steady speed phase
In healthy subjects, symmetrical activation in the medial sensorimotor cortex and supplementary motor area were observed during treadmill gait (see Fig. 6.1). These findings were consistent with results from a study using single-photon emission tomography [17]. Furthermore, it was also suggested that the various cortical areas play different roles in locomotor control. For example, the prefrontal cortex activated mainly during the acceleration phase of locomotion and gradually decreased at a steady speed (see Fig. 6.1, [18]). It was also revealed that the prefrontal activation change was more prominent at the higher locomotor speed, but cortical activation in the sensorimotor cortex was not associated with gait speed. Therefore, it was suggested that the prefrontal cortex was more involved in the adaptation of locomotion, but medial sensorimotor cortex was involved in the stable gait.
As for maintaining standing posture , Mihara et al. [19] reported cortical activation was associated with predictable and unpredictable postural perturbations. During the experiment, subjects were asked to maintain upright posture on the platform against the brisk forward and backward translation of the platform. Statistical analyses using general linear model with least square estimation revealed significant task-related OxyHb increases in the bilateral prefrontal cortices regardless of preceding warning cues. In the predictable condition, the supplementary motor area and the parietal association cortices were activated along with the prefrontal cortex (Fig. 6.2).
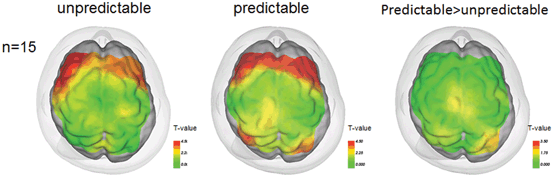
Fig. 6.2
Cortical activation associated with the postural perturbation in healthy subjects. Cortical activation mapping based on the task-related OxyHb signal increase. In the unpredictable condition, task-related OxyHb signal increase as prominent in the bilateral prefrontal cortex (left). In the predictable condition, postural perturbation evoked task-related OxyHb signal increase in the supplementary motor area and parietal association cortex as well as the bilateral prefrontal cortex (middle). SMA supplementary motor area, PFC prefrontal cortex
Based upon the findings from these previous studies of postural control , the prefrontal cortex appears to be involved in the attentional processing of postural maintenance against external perturbation [20], whereas the supplementary motor area and parietal association cortical activations were more prominent preceding warning cues, suggesting that the latter areas are involved in the intentional control of posture.
Application of Functional NIRS Study for Investigating Postural and Gait Impairment After Neurologic Disorders
Patients with stroke or movement disorders , including idiopathic Parkinson disease (iPD) and spinocerebellar ataxia (SCA) , show balance impairment and impacts on activities of daily living. In the field of rehabilitation medicine, balance impairment is one of the main therapeutic targets. As described in the previous section, fNIRS can monitor activation of the cortical networks that are vital for postural control . Figure 6.3 shows the correlation between balance function and task-related OxyHb signal changes associated with the unpredicted postural perturbation in hemiplegic stroke patients with a subcortical lesion. Significant correlation between balance function and cortical activation was observed in several cortical areas including the supplementary motor area and affected prefrontal cortex [21]. These results suggest that the recovery of balance is associated with changes in cortical activation, as the upper and lower limb recover after stroke [22−25].
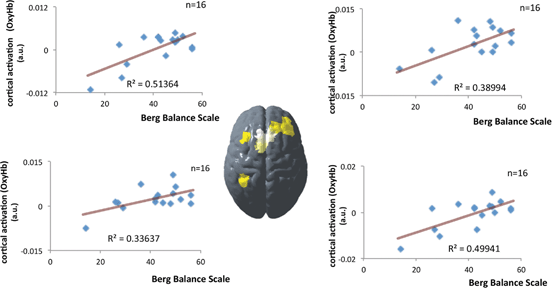
Fig. 6.3
Correlation between balance function and task-related OxyHb signal changes. Cortical region in which a significant correlation between balance function and task-related OxyHb signal changes was observed. Note that the left side of the figure represents the unaffected side of the patients. Each scatter plot represents the correlation between OxyHb signal changes and individual balance functions. BBS Berg balance scale
Functional NIRS measurements can be useful as surrogate markers of balance recovery after rehabilitation. Figure 6.4 illustrates two patients with Parkinson disease who received 4 weeks of inpatient rehabilitation and medication adjustments. Cortical activation associated with the postural perturbation increased in widespread cortical areas including the prefrontal cortex and supplementary motor area in both patients. Considering the subcortical nature of the iPD, increased cortical activation may serve a compensatory role for the subcortical dysfunction. Although there are several issues to be elucidated, these results imply that fNIRS could be used as the surrogate marker of the functional recovery or reorganization after rehabilitative interventions in various neurological disorders.
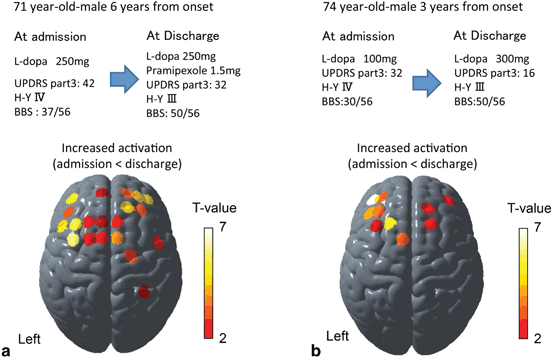
Fig. 6.4
Cortical activation change along with the balance recovery in PD patients. Postural perturbation-related cortical activation mapping change between at admission and at discharge based on the task-related OxyHb signal increase in two PD cases with inpatients rehabilitation. In both cases, cortical activation was increased as the recovery of balance function in widespread cortical area including the bilateral prefrontal, premotor, and supplementary motor area. BBS Berg balance scale
Functional NIRS as the Tool for Motor-Learning Studies
Motor learning is vital for the acquisition of motor skills in the daily lives of both healthy individuals and those with neurological disorders. Motor learning comprises motor sequence learning and motor adaptation in experimental settings [26]. Motor sequence learning is assessed by incremental acquisition of movements with repetitions whereas motor adaptation refers to ability to compensate for environmental changes. Till date, most human studies regarding motor sequence learning used PET or fMRI and investigated the neural mechanisms underlying learning of sequential movements of fingers or the feet in a supine position. However, motor learning usually occurs under postural control since most movements are executed while subjects are sitting or standing in a daily situation. As described above, because of less postural restriction, fNIRS is better suited for investigating the motor learning in daily circumstances.
Using fNIRS, Hatakenaka et al. [27] studied the cortical activation change during the pursuit rotor (PR) task in healthy subjects. A pursuit rotor (PR) is one of the tools used to evaluate motor sequence learning by measuring ability to keep a stylus on a rotating target. In the PR task, there is no need for precise control of finger movements, but it requires motor control of proximal parts of the upper extremity including the shoulder and elbow as well as postural control for sitting [28]. In this study, 18 right-handed healthy subjects performed eight repetitions of 30-s PR tasks followed by 30-s rest periods. Motor skill gains were evaluated by contact time of stylus and target. During eight repetitions of PR tasks, cortical activation in the frontoparietal cortices was evaluated with fNIRS.
Task-related OxyHb increases were observed in the sensorimotor cortices, prefrontal cortex, and premotor cortex as task performance improved. Interestingly, the center of task-related OxyHb increase was observed in the presupplementary motor area initially, but it shifted caudally to the supplementary motor area with cycle repetitions. These data suggest a different cortical role between presupplementary area and supplementary motor area in motor skill-learning process.
Functional NIRS was also used to investigate the adaptation-learning process. We investigated the cortical activation changes during the adaptation of reaching with robotic devices using a 3D virtual-reality (VR) system in which the limb coordination patterns are altered [29]. In this study, seven right-handed healthy subjects participated. The upper limb training system used was mediated by electro-rheological fluid actuators with 3-degrees of free movement range [30]. The subjects are given visual feedback of the position and the movement of the gripping handle of the robot arm as the colored ball (Object) in the 3D VR space on the 13′-monitor (Fig. 6.5a). The experiment consisted of 16 cycles of alternating 12-s rest and 8-s task periods. In the rest period, the floating target (Target) was fixed at the near-lower-left corner (home position) of the VR space. In the reaching task period, the Target moved from the home position to the far-upper-right corner, and then returned to the home position in 8 s, and subjects were asked to follow the Target by moving the robot arm. The distance between the Object and the Target was indicated as color of the Object. The mean distance in each cycle was calculated as a performance measure.
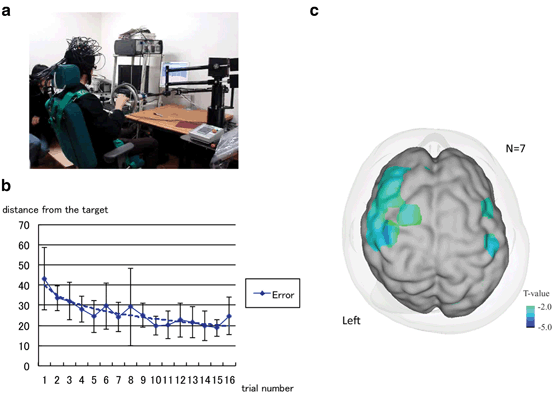
Fig. 6.5
Adaptation-learning task with the 3D robotic rehabilitation system. a Experimental setting of robotic rehabilitation system with functional NIRS. In a virtual reality space, subjects were asked to follow the target. b Averaged distance from the handle position and the target. Averaged distance gradually reduced with cycle repetitions, indicating that the subjects could adapt to the visuomotor reaching task. c Significant correlations between error and cortical activation. Cortical activation in the left premotor cortex was increased as the adaptation-learning improved
Cortical activation was measured as oxygenated hemoglobin signal change using a 50-channel fNIRS system from the frontoparietal area. Task-related cortical activation of each subject was modeled using the two orthogonal covariates [31]. The first was the Task covariate modeled as a box-car function in all task periods and the second was the Error covariate that comprised a box-car function scaled by the mean distance. The Error covariate was mean corrected and orthogonalized with respect to the first covariate. Both covariates were convolved with the canonical hemodynamic response function and used in a GLM analysis . Group analysis was performed using the random-effects model .
Mean distance for the seven subjects gradually reduced with cycle repetitions, indicating that the subjects learned to adapt to the visuomotor reaching task in VR space. Group analysis of fNIRS signals showed significant main effects in the bilateral prefrontal, bilateral premotor, and left primary sensorimotor area. Significant negative correlations to the Error covariate were also found in the left prefrontal and premotor area (see Fig. 6.5). Although broad cortical networks were involved in the visuomotor adaption learning of reaching task, the prefrontal and premotor area were primarily involved in the early stage of the adaptation process.
< div class='tao-gold-member'>
Only gold members can continue reading. Log In or Register a > to continue
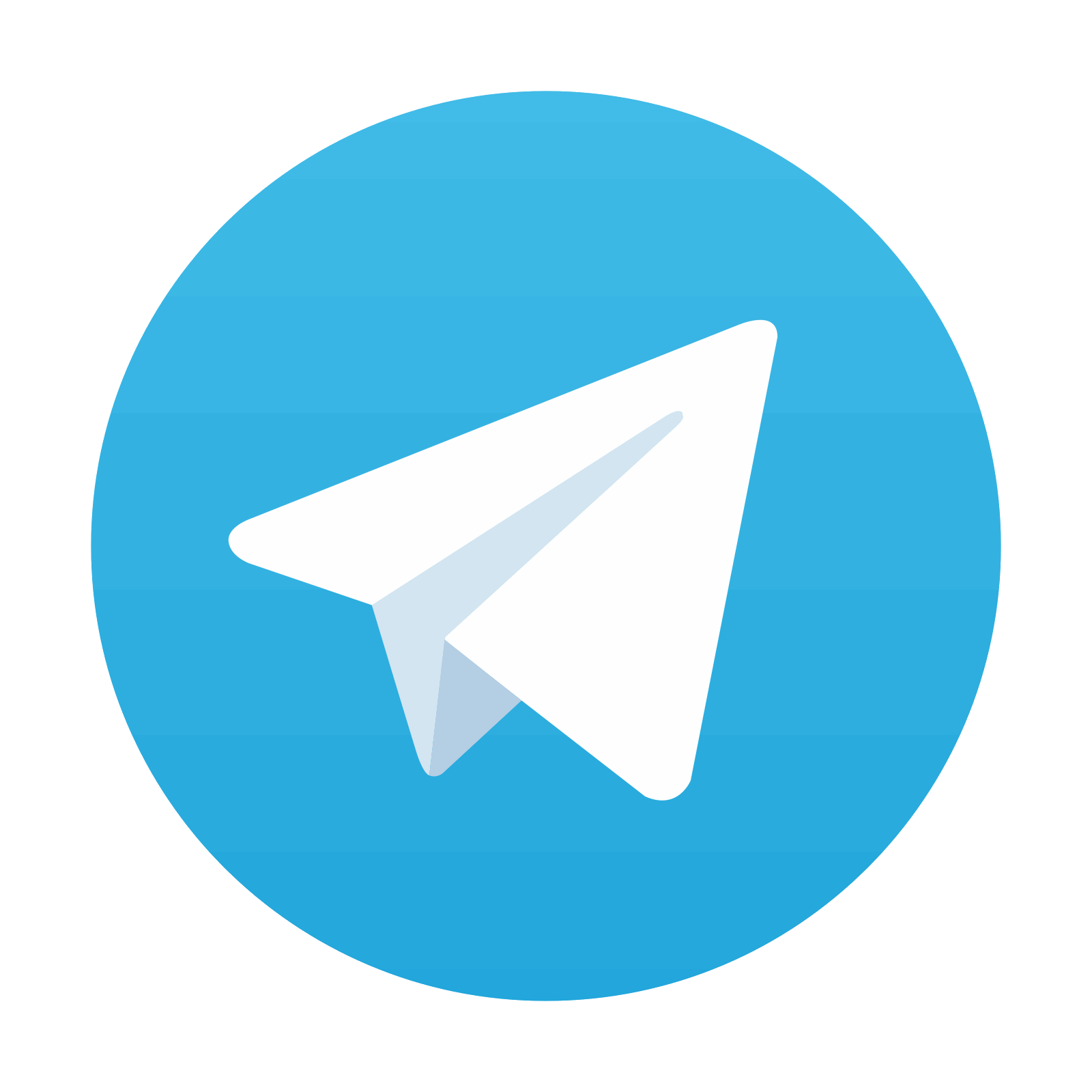
Stay updated, free articles. Join our Telegram channel

Full access? Get Clinical Tree
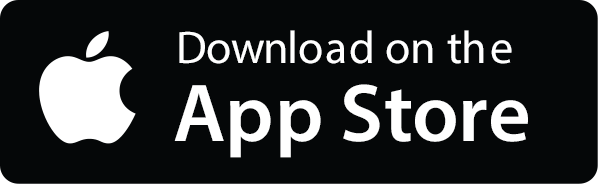
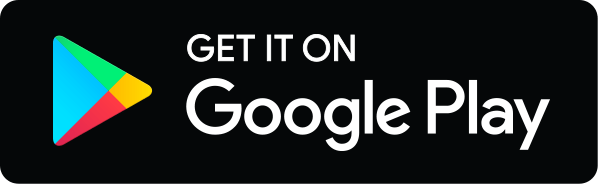