Fig. 1
A 29-year-old female presented to the ER with severe headaches. She was found to have a small paramedian right parietal intracerebral hemorrhage. Sagittal CTA (a) shows an enlarged paramedian vein (white arrowheads). One can almost make out a nidus (black arrowhead). AP (b) and lateral (c) angiography show an enlarged anterior cerebral pericallosal artery (white arrows) and superior parietal artery (yellow arrowhead) supplying a subcentimeter bAVM (black arrowhead). There is a single paramedian superficial parietal draining vein which drains into the superior sagittal sinus. (blue arrowheads). The patient was treated with open surgical resection
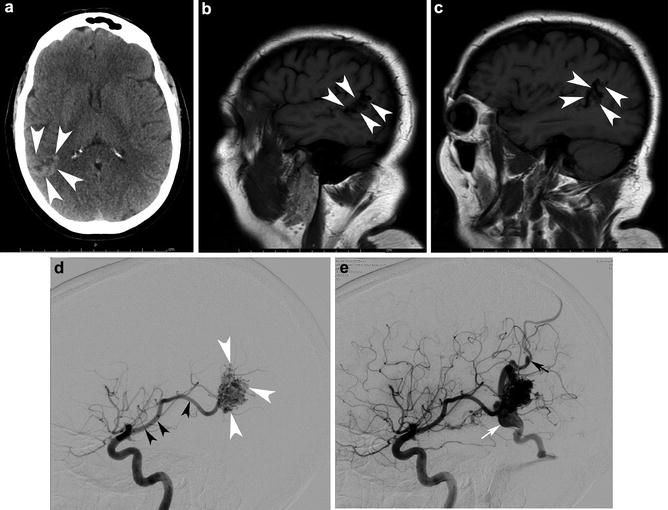
Fig. 2
A 46-year-old male was evaluated with noncontrast head CT following a seizure. Transaxial noncontrast head CT (a) shows a right angular cortical and subcortical hyperdensity (white arrowheads). Two sagittal precontrast T1-WI images (b, c) show the lesion spanning the cortices of the superior and middle temporal gyri (arrowhead) and involving the angular gyrus. Right ICA angiograms in the lateral projection in the early arterial and mid arterial phases (d and e, respectively). The mid arterial phase depicts an enlarged angular branch of the right MCA (black arrowhead) terminating within the nidus (white arrowheads). Venous drainage is into two dominant veins: the veins of Labbe and Trolard. There is a venous ectasia on Labbe (white arrow) and stenosis (black arrow) associated with the vein of Trolard
Furthermore, those variables without clear physiologic implication that were significantly associated with hemorrhage in the univariate analysis were assessed as confounders using agreement analysis. In each case, the less clearly physiologic parameter was highly associated with one of the more plausibly physiologic parameters, confirming that the less physiologic parameters were acting as confounders. Small nidal size, a characteristic without clear physiologic implication, for example, showed 80 % agreement with one draining vein. Likewise, the presence of only deep venous drainage showed an 84 % agreement with one draining vein. The absence of pial-to-pial collaterals from different distributions had a 71 % agreement with one draining vein. Deep location had a 77 % agreement with one draining vein. These results confirmed that various bAVM characteristics with less plausible physiological implications likely serve as confounders in assessing hemorrhagic risk, thereby validating the selection of parameters with potential physiological implications for the multivariate analysis [43].
Not only does this study explain many of the literature findings of bAVM features associated with hemorrhage without clear physiologic implication, it paves the way for a patient-specific analysis of bAVMs from the standpoint of natural history risk assessment [45]. The diagnostic radiologist’s role in assessing for venous stenosis, number of draining veins, and aneurysms also becomes critical. Future studies will elaborate on and refine this model. While this model uses anatomic features as physiologic surrogates, with inherent limitations, new MRI modalities have tremendous potential to directly interrogate bAVM physiology [46, 47].
Epidemiology
The nature of the bAVM disease process, physician and institutional tendency, and difficulties in case detection lead to significant bias in the available epidemiological data and result in persistent uncertainty with regard to the epidemiology and natural history of bAVMs. The first population-based study to address the incidence and prevalence of bAVMs (in addition to intracranial vascular malformations (IVMs)) as a whole was the Olmsted County study [48]. Medical records from Olmstead County, Minnesota, were reviewed for all IVMs from 1965 to 1992. This study demonstrated an incidence of 2.05 per 100,000 person-years. The vast majority (85 %) of detected bAVMs were symptomatic, and the mean age at diagnosis was ~40 years. The authors noted an increasing detection rate during the course of the study that was presumably the result of improved understanding of bAVMs and technological advances such as improved medical imaging. In a population-based study from the state of Western Australia, a considerably lower incidence of 0.89/100,000 person-years was determined with a prevalence of only 5.47/100,000 [49]. In this population, the mean age at diagnosis was ~34 years, approximately 60 % of patients presented with hemorrhage and 30 % presented with seizures. Only 2.5 % of bAVMs in this study were incidental suggesting a degree of under-detection, a metric that has implications for our understanding of natural history. The authors of the Western Australia study noted that the incidence of bAVMs rose during the study period peaking at 1.46/100,000 person-years in 1989, exceeding the population rise. However, the incidence decreased from this point in spite of increasing availability of CT and MRI. The authors conclude that this trend argues for a low incidence of occult bAVM; however, this remains speculative. Interestingly, the authors also noted that headache, as a presenting symptom, did not exceed the incidence of headache in the general population, suggesting that this is an unlikely attributable symptom of bAVMs, a controversial conclusion.
The Scottish Intracranial Vascular Malformation Study (SIVMS) [50] was the first large population-based, prospective study of bAVM epidemiology. It used multiple sources of case ascertainment over a prospective 24-month period to identify “first-ever-in-life” diagnosis of any intracranial vascular malformation in Scottish adults. In this study, the incidence of bAVM was 1.12/100,000 person-years with a hemorrhagic presentation incidence of 0.89/100,000 person-years and a nonhemorrhagic presentation incidence of 0.23/100,000 person-years. Two additional population-based studies from North America, the New York Islands AVM Study and data from the Northern Manhattan Stroke Study (NOMASS), were published in 2003 and 2002, respectively [44, 51]. The NOMASS found three patients with bAVMs out of 207 patients with first-ever intracranial hemorrhage, suggesting that bAVMs account for 1.4 % of first-ever intracranial hemorrhage in an unselected population [44]. The estimated crude incidence for first-ever bAVM hemorrhage was 0.55/100,000 person-years. However, two of the three discovered bAVMs were in the posterior fossa, disproportionately high with respect to bAVM location, thereby casting doubt as to how representative the data is and, by extension, the generalizability of the study. In spite of these concerns, the New York Islands AVM Study produced similar results [51]. The New York Islands AVM Study encountered 284 AVM patients from a total of 21,216,467 person-years of observation for an incidence of 1.34/100,000 person-years. The crude incidence for AVM-related hemorrhage of 0.51/100,000 person-years was remarkably similar to that from the NOMASS data set. The mean age of bAVM rupture was ~31 years.
A Swedish population-based cohort study also reported similar findings with a bAVM incidence of 1.24/100,000 person-years [52]. Hemorrhage was by far the most common presentation seen in ~70 % with a presentation incidence of 0.87/100,000 person-years. Like others before, these authors concluded that incidental bAVMs are rare.
The issue of incidental bAVMs has been addressed, at least obtusely, by several population MRI studies. A German Air Force recruit study in which 2,536 males aged 17–35 years old underwent brain MRI as part of medical screening for flying service found bAVMs in a surprising 0.2 % [53]. While this is significantly higher than previous clinical studies, it is within the range reported in autopsy series, as summarized in a review of the epidemiology of bAVMs by Berman et al. [54]. However, several other MRI screening studies have failed to identify any patients with bAVM [55–57]. This may in part be due to the younger age of participant screened in the German study [53] in that older individuals are less likely to harbor an unruptured or otherwise asymptomatic bAVM [10]. Furthermore, the imaging parameters appear to be more rigorous in the German study.
There are considerable challenges associated with estimating epidemiology in a rare and potentially occult disease. Appropriate studies require adequate radiological and/or pathological investigation to reliably diagnose bAVMs as well as to exclude other entities that may have a similar presentation such as cavernous malformations or dural arteriovenous fistulas (dAVF) [7]. While this seems reasonably achievable, clinicians may forgo aggressive investigation due to a myriad of reasons including clinical urgency or futility. Pathological investigations may lack sufficient zeal given the minor role bAVMs may play on a population basis in the theater of intracranial hemorrhage. Representative cohorts should preferably include individuals diagnosed at large referral centers as well as small peripheral hospitals in addition to those who have died in the community. To this end, many of the larger, population-based trials would be expected to be fairly accurate. While the challenge is significant, some comfort can be taken by the relatively similar results reported by large, population-based studies [48, 58]. The epidemiology data prior to 2001 is well reviewed by Al-Shahi and Warlow [7]. The authors reported a bAVM incidence of 1/100,000 person-years and a prevalence of 18/100,000. Rupture of a bAVM was estimated to account for 1–2 % of all strokes and 4 % of intracerebral hemorrhage. This figure rises to as high as 33.5 % of hemorrhages in young adults (< age 40). Contrary to later reports, Al-Shahi and Warlow estimated that 15 % of bAVMs are asymptomatic at the time of diagnosis. However, in line with later studies, they estimated that approximately 60–70 % present with hemorrhage and 20 % with seizures. A more recent study has provided updated data [59]. Review of a 10-year period of Kaiser Permanente Medical Care Program in northern California medical records, radiology reports, and administrative databases yielded an overall bAVM detection rate of 1.42/100,000 person-years [59].
The Brain Arteriovenous Malformation
Embryology
It is likely that bAVMs develop in utero, during the vascular development of the central nervous system. An understanding of bAVM anatomy and physiology therefore requires a basic understanding of the embryology of the neurovasculature.
The neural tissue and vascular tissue develop in concert in the early embryo. Two processes are responsible for the vascularization of the central nervous system and indeed the developing fetus: vasculogenesis, the formation of the primitive endothelial cells [60], and angiogenesis, which follows vasculogenesis and is responsible for remodeling and expansion of the network [61]. While there remains some debate based on rare observations, bAVMs are generally considered to be congenital lesions that likely form, at least as immature precursors, during angiogenesis of the central nervous system [62]. It is impossible to convey the current theories on bAVM pathophysiology without at least an introduction to the concepts of vasculogenesis and angiogenesis as they pertain to the central nervous system. The following sections will attempt to cover these complicated subjects in an expedient fashion. Numerous texts and reviews are available which cover these processes in greater detail [1, 63–65].
Closure of the neural tube occurs at around 3–4 weeks gestation and signals the beginning of the embryonic stage. In regard to the morphogenesis of the cerebral circulation, the embryonic stage can be further classified into the prechoroidal, choroidal, and brachial phases. During the prechoroidal stage, blood vessels develop by way of vasculogenesis from the meninx primitiva on the neural tube surface. The meninx primitiva is the mesenchymal covering from which the dura, arachnoid, and pia mater are derived. Within vasculogenesis, the critical interaction occurs between the principal signaling molecules, vascular endothelial-derived growth factors (VEGF), and their receptors (VEGFR). This is a relatively large group of molecules (VEGF A-E), which have recurring roles in vasculogenesis and angiogenesis both in physiological and pathological states. The interaction between VEGF-A and VEGFR-2 promotes angioblasts to form angiocysts, which ultimately migrate to form the early vascular network of the meninx [60].
The neural tissue is supplied by simple diffusion from the primitive vascular plexus on its surface during the prechoroidal phase. As the cranial neural tube develops into the five vesicles (telencephalon, diencephalon, mesencephalon, metencephalon, and myelencephalon), the neural tissue becomes too abundant to be supported by simple diffusion from the peripheral vascular plexus. Support is added when the choroid develops as an invagination of the meninx from the roof of the neural tube into the central canal as the choroid is quite metabolically active and brings with it its vascular supply, thereby initiating the choroidal phase [63–65].
The brachial phase sees the emergence of the adult-type arterial pattern by a series of regressions and enlargements of preexisting transverse vessels. As the developing central nervous system exceeds the capacity of simple diffusion, vascular channels form within the parenchyma to keep up with the metabolic demands of the rapidly developing primitive brain. Initially there is vasodilation directly mediated by increases in nitric oxide (NO). Following vasodilation, there are significant increases in vascular permeability again mediated by nitric oxide, however, this time via upregulation of VEGF family molecules. VEGF acts directly on endothelial cells to effect cell-cell adhesion molecules resulting in leakage of plasma proteins. These plasma proteins facilitate the breakdown of extracellular matrix components and at the same time provide the necessary temporary scaffolding allowing migration of endothelial cells [66]. Pseudopodia are formed with a lead cell that penetrates between glial elements [67]. These early vascular channels are orientated at right angles to the pial surface with little branching.
A similar pattern occurs from the ependymal surface of the primitive ventricles. For both the more dominant ventriculopetal and the more modest ventriculofugal cerebral vascular development, VEGF is again a key factor driving the development with high VEGF levels found in the deep subventricular zone acting as a powerful chemoattractant to vascularization [60]. The migrating and dividing endothelial cells initially form solid cords of elongated endothelial cells directed toward high concentrations of VEGF, particularly in the subventricular zone. Following the formation of solid cords, there is canalization with lumen formation. This type of angiogenesis is referred to as “sprouting” and is the dominant form in the developing CNS [68]. The definitive intraparenchymal vascular pattern is not arrived at immediately. Immature vessels, which are little more than continuous vascular channels at this stage, without recognizable arterial, capillary, or venous components, undergo further selection with dominant vessels undergoing further branching, while other channels regress. To this end, an interaction between the endothelial cells and their supportive structures, namely, smooth muscle cells (SMCs) and extracellular matrix (ECM), is critical. Both the ECM and the SMCs provide a source of growth factors and supportive ligands, which lend permanency to the developing vascular structures. During both vasculogenesis and angiogenesis within the developing CNS, the VEGF family of signaling molecules plays the dominant role and in particular is critical for endothelial cell maturation and replication [60, 63–65].
In addition to these critical signaling pathways, several other molecules have been identified which play a role not only in endothelial cell signaling but also in directing the supporting SMCs and interactions with the ECM. ANG-1 interacts with the TIE2 receptor to encourage recruitment and interaction between the endothelial cell and the supporting environment. Equally important is the ANG-2 molecule, which has an antagonistic effect on the TIE2 receptor necessary for appropriate vascular remodeling to form the final organized functional arterial tree [60]. Further signaling molecules include endoglin and the related Alk1 receptors which play a role in endothelial cell to cell interactions during capillary formation and act on supporting structures, triggering mesenchymal cells to differentiate into pericytes and SMCs [69, 70]. There has been an increasing interest in these molecules as their role in pathological processes becomes more apparent, particularly given their role in genetic forms of bAVM (Osler-Weber-Rendu (also called hereditary hemorrhagic telangiectasia)) [66, 71, 72].
Pathophysiology
On gross inspection, bAVMs are composed of one or more, often enlarged, afferent arterial feeders with one or more, frequently dilated, efferent veins. The core pathophysiology in bAVMs is that of arteriovenous shunting. The degree of shunting has been categorized as either “nidiform” or “fistulous,” though these two types can coexist within a single lesion (Fig. 3) [1, 73, 74]. (It is important that the reader not confuse this distinction with dural (Fig. 4) or pial (Fig. 5) arteriovenous fistula, which in the former reflects an abnormal connection between the artery and vein within the dura and in the latter refers to an abnormal single connection within the pia, without a subpial component). Rather, this is a description of the physiologic shunting between the arterial and venous compartments of a bAVM. The nidiform type demonstrates a larger network of abnormal vascular channels leading from the arterial compartment to the venous compartment. These channels comprise the “nidus” which gives the bAVM its mass-like appearance and which adds a conduit that separates the arterial feeders from the venous outflow. The bAVMs that demonstrate a more direct physiologic shunt, and therefore possess less of a vascular network between the arterial and venous compartments, instead possess a shunt in which the artery and vein are more directly connected [75, 76]. In the end, the nidiform and fistulous designations exist not as absolutes but along a continuum. The fistulous physiology is more rare than the nidiform type, representing only around 2 % of patients in some series [6]. Interestingly, this morphological type is typically reported on the surface of the central nervous system [1].
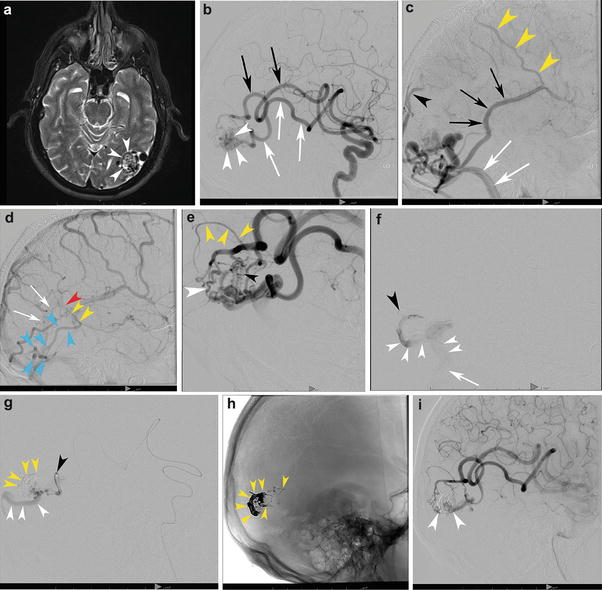
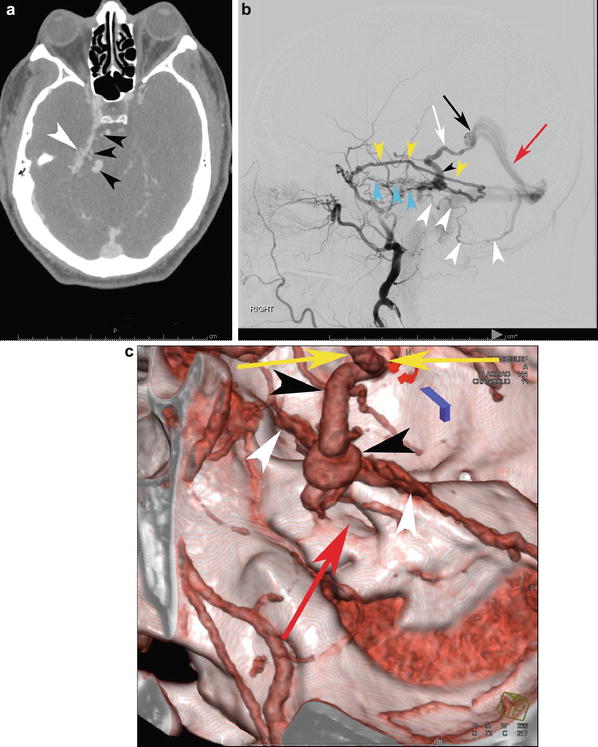
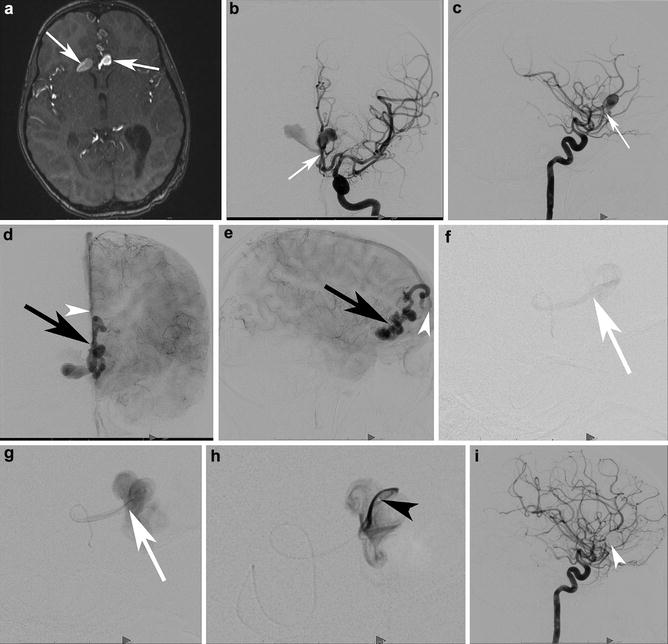
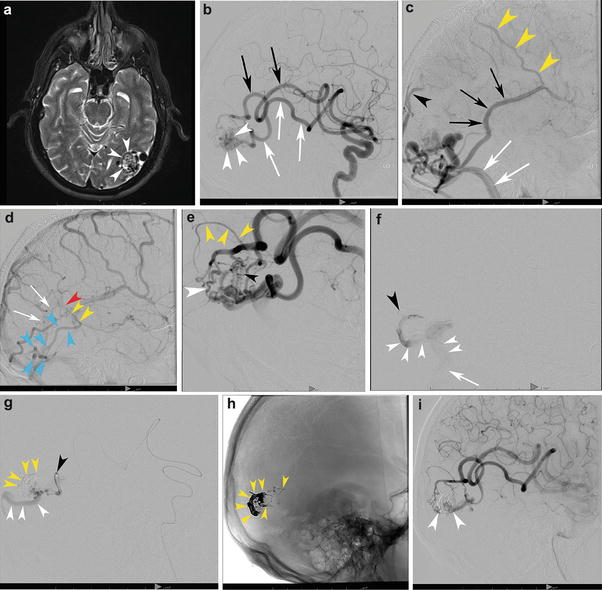
Fig. 3
A 58-year-old male with a left posterior temporal/temporo-occipital brain AVM discovered incidentally following a motor vehicle accident. T2-WI transaxial MRI (a) shows the classic appearance of the nidus within the cortex and subcortical white matter of the left temporo-occipital region (white arrowheads). The decision was made to treat the patient with embolization and surgery. (b) Left internal carotid angiography, lateral projection, in the early arterial phase demonstrates two dominant arterial feeders, both grossly enlarged: the posterior temporal (white arrows) and temporo-occipital (black arrows) divisions of the left MCA supplying the nidus (white arrowheads). The capillary (c) and venous (d) phases depict the bAVM venous outflow. Venous outflow is predominantly into the left sigmoid sinus (white arrows) as well as retrograde into the vein of Labbe (black arrows) and out via Trolard (yellow arrowheads). There is lesser outflow within cortical occipital veins and into the posterior aspect of the superior sagittal sinus (black arrowhead). (d) A superficial vein (blue arrowheads) courses along the inferior and posterior occipital lobe superficially and then heads anteriorly along the paramedian occipital lobe, draining into the basal vein of Rosenthal (yellow arrowheads), vein of Galen (red arrowhead), and straight sinus (white arrows), a unique “deep” venous drainage for an otherwise superficial bAVM. Lateral angiogram (e) following a single pedicle embolization shows both fistulous (white arrowhead) and nidiform (black arrowhead) components of the bAVM. Note the en passage supply from the parieto-occipital branch which goes on to supply the normal brain (yellow arrowheads). Superselective microcatheter angiography prior to embolization demonstrates discrete outflow into separate veins from each microcatheter injection, a multicompartmental physiology. (f) The catheter tip is depicted by the black arrowhead. Venous drainage from the bAVM (white arrowheads) is directed inferiorly into the sigmoid sinus (white arrow). (g) This pedicle opacifies a separate compartment which, in distinction, drains posteriorly along the occipital pole (white arrowheads). The catheter tip is depicted by a black arrowhead. The shadow of the glue cast can be seen (yellow arrowheads). (h) An unsubtracted lateral x-ray shows the glue cast following three pedicle embolizations (yellow arrowheads). Lateral angiogram in the arterial phase (i) shows significant devascularization of the nidus (white arrowheads) following three pedicles of embolization
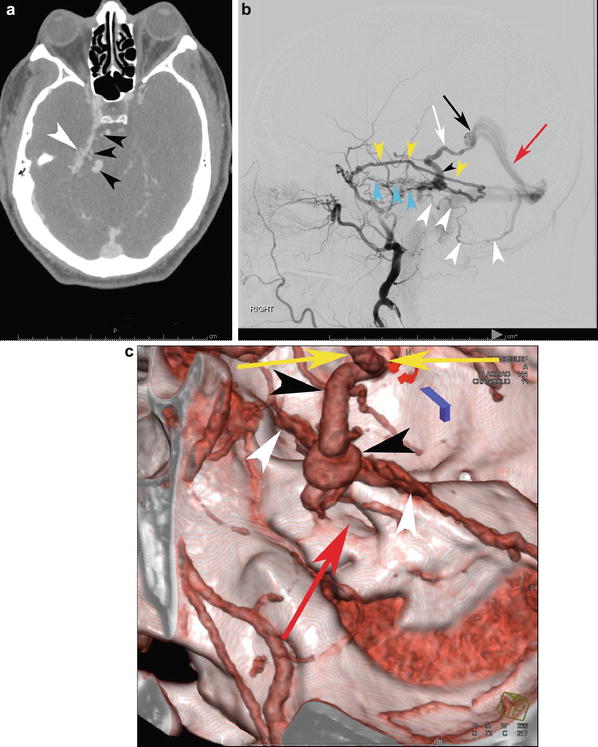
Fig. 4
A 53-year-old male presented to the ER for worsening episodes of dizziness. CTA was performed (a) which demonstrated increased vascularity abutting the right petrous ridge (white arrowhead) with a few enlarged enhancing curvilinear structures adjacent to the pontomedullary junction on the right (black arrowheads). This could easily be confused for a bAVM given its almost-nidiform appearance. (b) Catheter angiography (right external carotid anterior trunk in the arterial phase) shows a dural arteriovenous fistula along the right petrous ridge with dominant supply from the petrosquamosal (yellow arrowheads) and basal tentorial (blue arrowheads) branches of the right middle meningeal artery. Dominant venous outflow is into the petrosal vein (black arrowhead) into the basal vein of Rosenthal (white arrow) which drains out via the vein of Galen (black arrow) and straight sinus (red arrow). Additionally, there is infratentorial drainage into a superficial cerebellar hemispheric vein (white arrowheads). Reformatted CTA can assist with surgical planning (c). The vascular fullness along the petrous ridge is apparent (white arrowheads). The petrosal vein courses toward the viewer in this projection (black arrowheads) into the basal vein of Rosenthal (at the top of the image, yellow arrows). The internal auditory canal (red arrow) is included as a helpful osseous landmark for surgery
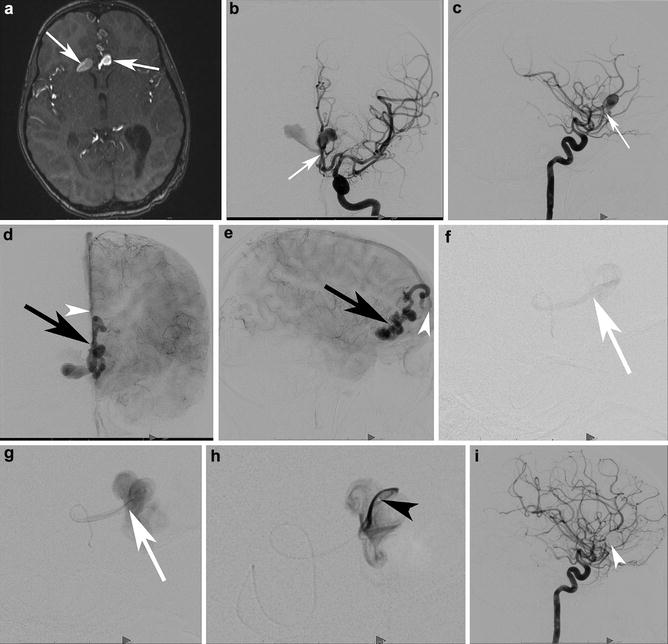
Fig. 5
A 6-year-old girl presented with subarachnoid hemorrhage and intraventricular hemorrhage. Transaxial time-of-flight MRA (a) demonstrates unusual ectasias or aneurysms in the region of the ACAs, one oriented towards the right and the other to the left (white arrows). Left internal carotid AP (b) and lateral (c) angiograms in the early arterial phase demonstrate a rapid early opacification from a small frontal branch of the ACA, arising from the right ACA (white arrow shows the junction of the artery and vein). Capillary phase AP (d) and lateral (e) angiograms demonstrate the early opacification of a paramedian cortical vein with multiple ectasias (black arrow), draining into the anterior aspect of the superior sagittal sinus (white arrowhead). The individual feeding artery was catheterized and an arteriogram was performed (f, g). Note the absence of a nidus or subpial component. The artery drains directly into the ectatic vein (junction marked with a white arrow). The microcatheter was advanced across the fistula and into the ectactic venous outflow. (h) depicts the microcatheter tip within the first venous ectasia (black arrowhead). The fistula was then coiled. Follow-up internal carotid angiogram in the lateral projection (i) demonstrates no residual shunting. The coil mass is positioned within the ectactic primary draining vein, extending proximally into the arterial supply (white arrowhead)
Brain arteriovenous malformations are found in the supratentorial compartment in the overwhelming majority (>90 %) and demonstrate a predilection for the MCA territory [6]. Deep structures are involved in approximately 15 % of cases [43]. In approximately 50 %, there is deep venous drainage [77].
There are many ways of categorizing and understanding bAVMs. Some authors have suggested that there is a degree of predictability in both the pattern of arterial supply and venous drainage based on the location of the bAVM [1]. This is useful to keep in mind when interpreting the imaging appearance of lesions, particularly in addressing arterial supply and venous drainage. Cerebral bAVMs may be topographically divided into those that reach the cortex, those restricted to a deep location, and those involving the choroid [1]. Lesions involving the cortex are further classified into four categories:
1.
Cortical lesions are on or reach the cortex and supplied exclusively by cortical arteries with only superficial venous drainage. It should be noted that the basal veins of Rosenthal are not, anatomically speaking, deep cerebral veins as they lie on the brain surface and may drain cortical bAVM (i.e., temporal lobe lesions). They are, however, considered deep veins from the neurosurgical point of view given their relatively inaccessible location and, when involved in the surgical treatment of bAVMs, have higher associated morbidity. Cortical lesions have also been referred to as sulcal bAVMs.
2.
Cortico-subcortical bAVMs, also referred to as gyral bAVM, are supplied by cortical arteries and drained by cortical veins. However, they may also drain into the deep venous system if the transcerebral venous pathways remain open (Figs. 6 and 7). Unusual cortical pathways into the medial temporal cortical venous drainage can also lead to deep venous drainage.
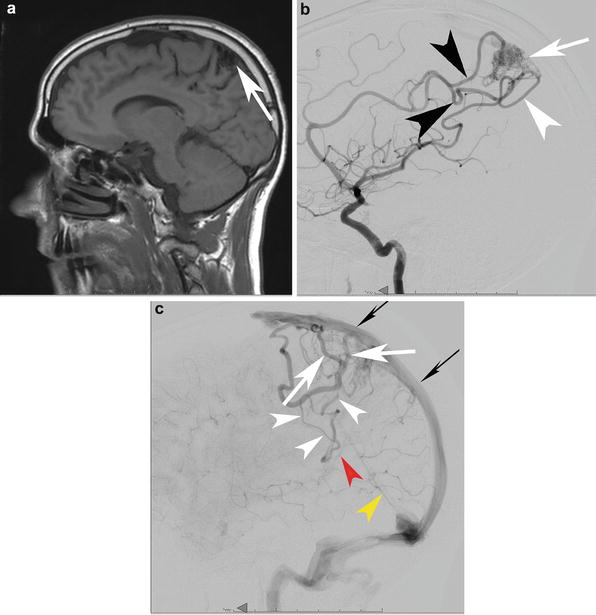
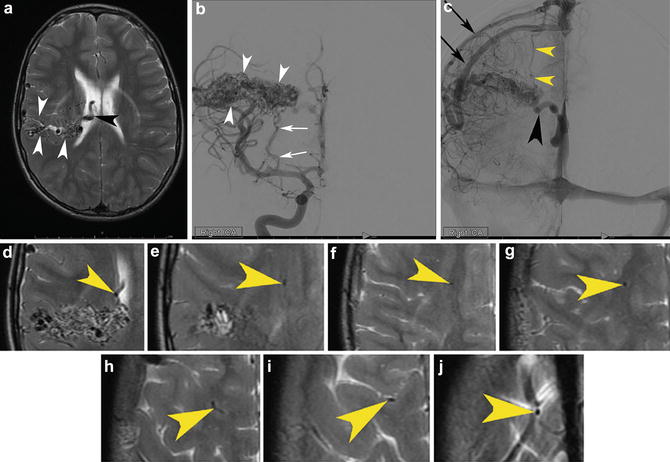
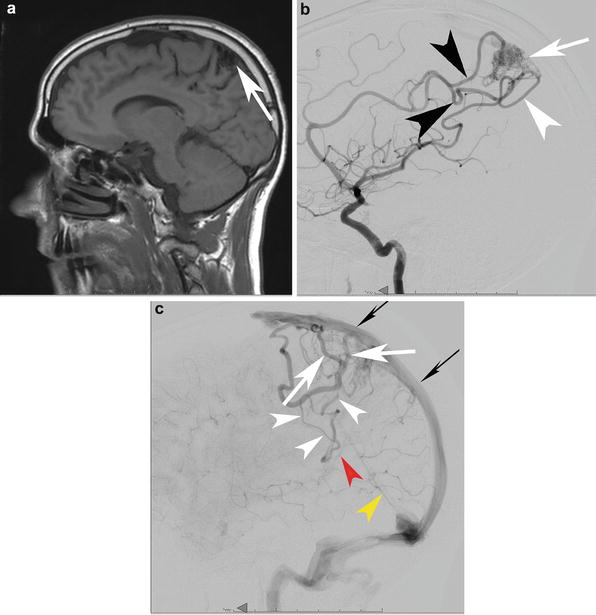
Fig. 6
A cortico-subcortical bAVM in a 33-year-old patient found to have a bAVM during a work-up for headaches. (a) A sagittal T1-WI precontrast MRI depicts the cortical and subcortical bAVM (white arrow). Lateral angiography from a right ICA injection in the mid arterial (b) and late arterial (c) phases depicts a parietal cortical and subcortical bAVM (white arrow) with supply from the ACA parietal divisions (black arrowhead) and MCA parietal division (white arrowhead). The venous outflow is depicted in (c). There is superficial venous drainage into the superior sagittal sinus which might be expected in a parasagittal bAVM such as this one (white arrows depict the venous outflow into the superior sagittal sinus (skinny black arrows)). More unusual is the transcerebral pathway that remains open in this case and leads to deep venous drainage despite the relatively superficial nidus (white arrowheads). Finally, it drains into the vein of Galen (red arrowhead) and straight sinus (yellow arrowhead). These latter two veins barely opacify because of the relative paucity of opacified blood coming through the transcerebral venous conduit relative to the large amount of unopacified blood coming through both cerebral hemispheres (it is still the late capillary phase on the right– the side of the injection.) into the deep venous system
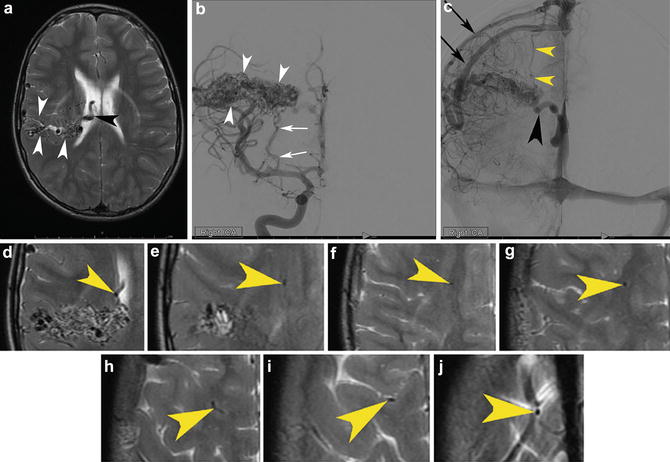
Fig. 7
An 11-year-old boy was sent for an MRI as part of an evaluation for with headaches. MRI was performed which revealed a right parietal corticoventricular brain arteriovenous malformation with superficial and deep venous drainage and measuring 5.1 × 2.0 × 2.0 cm in maximum transverse, craniocaudal, and AP dimensions, respectively. (a) Transaxial T2-WI images demonstrate the classic flow voids extending from the cortex to the ependymal surface (white arrowheads). The large flow void within the ventricle represents a deep vein (black arrowhead). Right internal carotid angiography in the early arterial (b) and later arterial (c) phases shows the nidus extending from the cortex to the ependymal surface (white arrowheads). Note the classic perforator supply from the lateral lenticulostriate arteries (white arrow). The late arterial phase clearly depicts both superficial (black arrow) and deep (black arrowhead) drainage. In addition, there is an unusual transcerebral vein which drains from the deeper aspect of the lesion into the superficial venous system (yellow arrowhead). That transcerebral vein is visible on the MRI T2-WI sequence (magnified view (d–j) going from the level of the ventricle sequentially to the level of the vertex), a testimony to the excellent soft tissue contrast that MRI affords. It would be difficult, however, to see this finding prospectively in the absence of the catheter angiogram (yellow arrowheads)
3.
Corticoventricular bAVMs extend from the cortex to the ventricular surface (Figs. 7 and 8). These lesions often demonstrate the classic pyramidal morphology of bAVM with a broad cortical surface and apex pointing to or indeed reaching the ventricular margin or subventricular zone. These lesions will be supplied by both cortical and perforating arteries and have both superficial and deep drainage.
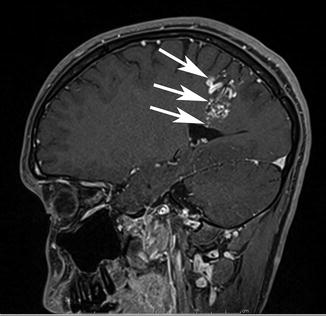
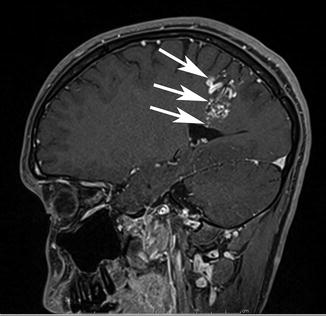
Fig. 8
A sagittal postcontrast image to the right of midline demonstrating a classic pyramidal or wedge-shaped corticoventricular bAVM in a 33-year-old, discovered during a work-up for headache. Note the excellent soft tissue contrast on MRI and the precise anatomic localization
4.
Corticocallosal bAVMs share many similarities with corticoventricular lesions, having both deep and superficial draining veins. The arterial supply to the corpus callosum is derived from cortical vessels; however, it may appear to represent perforators, particularly anteriorly and at the splenium [1, 78].
Deep lesions can be found in any structure of either the supra- or infratentorial compartments (Fig. 9). They are exclusively supplied by perforating arteries and drained via the deep venous system. These lesions obviously pose the greatest challenge to open surgical treatment. Choroidal bAVMs are of particular importance to prospectively identify as their extra-axial location makes them both surgically and endovascularly accessible. This extra-axial location can be challenging to identify on cross-sectional imaging in extensive lesions or in those lesions that do not respect anatomical boundaries. The key to appreciating this topographical subtype of bAVM is in identifying supply by choroidal and subependymal arteries arising from the circle of Willis including the basilar tip. Cortical arterial supply is not a feature of these lesions. Drainage is typically via ventricular veins but may also involve transcerebral veins. While these features are largely reliable, it must be remembered that bAVMs are dynamic lesions capable of evolution and adaptation. This is particularly true for venous drainage which may shift significantly if previously established drainage undergoes thrombosis or becomes stenotic [1].
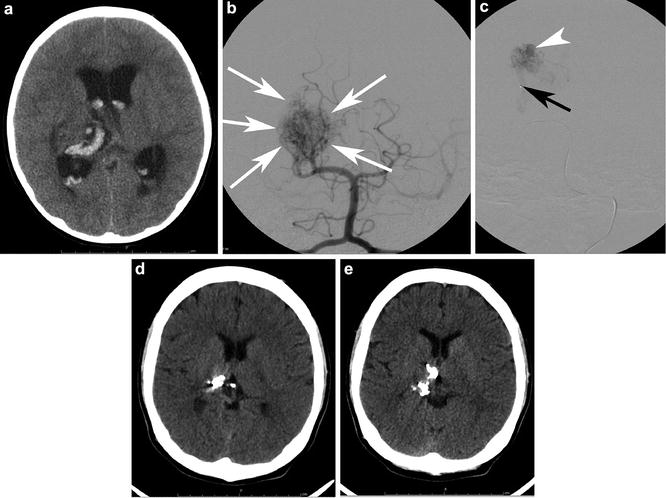
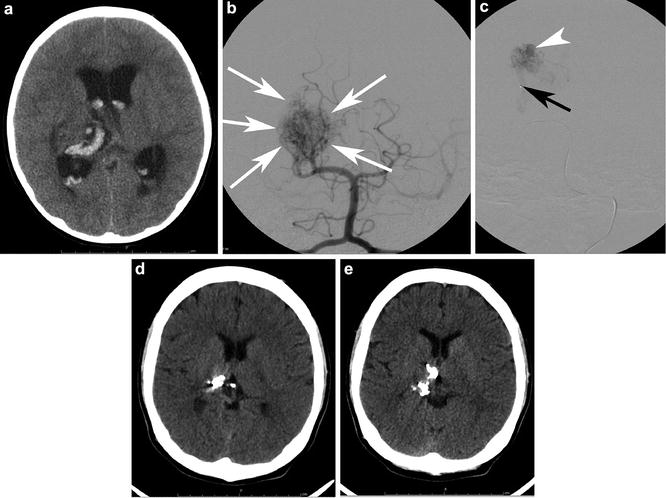
Fig. 9
A 16-year-old with a history of a ruptured diffuse thalamic bAVM. (a) depicts the intracerebral and intraventricular acute hematoma. Note the hydrocephalus. The patient was sent for catheter angiography and possible embolization. AP Towne projection from a left vertebral injection (b) shows a diffuse thalamic and ventricular nidus (white arrows) with supply from right posterior cerebral artery (PCA) perforators and the posteromedial choroidal artery. The patient was sent for embolization (c) to temporize the lesion for Gamma Knife therapy. The microcatheter tip is depicted by the black arrow. A pseudoaneurysm (white arrowhead) was successfully targeted. Two sequential transaxial slices from a noncontrast head CT (d and e) acquired 4 years later show the onyx (embolic) cast, the very hyperdense curvilinear material in the right thalamus and posterior body of the right lateral ventricle. The patient has been stable neurologically
Histology and Histopathology
Microscopic examination of bAVMs demonstrates abnormal vessels from both the arterial and venous compartments, with still further vessels that do not easily fit into either venous or arterial categories [62]. While it is commonly stated that the bAVM nidus lacks brain parenchyma, gliotic neural tissue is often seen at the margin and even within the lesions [61, 79]. There is frequently evidence of microhemorrhage that does not have a clinical event correlation and thus implies subclinical extravasation [79]. Likely related to this phenomenon, evidence of calcification can be seen within bAVM vessel walls and has even been reported within the adjacent parenchyma [80]. This calcification is more easily identified histologically [80], although may occasionally be identified on imaging [81]. Clusters of abnormal, muscularized arteries become increasingly frequent as one approaches the site of shunt [62]. One of the hallmarks of these abnormal vessels is the marked variability in the wall thickness, particularly involving the media. There is both thickening and focal thinning of the vascular wall with medial fibrosis and duplication and degradation of the elastic lamina [62]. The media itself may be partially or completely absent in sections or alternatively demonstrate marked structural disarray with separation into an inner and outer coat by an aberrant elastic lamina [61, 62, 79, 80]. Veins often have an “arterialized” appearance with lack of elastin and hypertrophy of the smooth muscle layer [69]. However, in sections there may be near complete loss of normal venous mural architecture, being apparently replaced by the fibrous tissue [62, 79].
The vast majority of bAVMs are sporadic; however, bAVMs associated with genetic syndromes have lead to insight regarding the roles that various genes play in vascular development and in the development of bAVMs [1, 61]. It comes as little surprise that many genes with a role in either vasculogenesis or angiogenesis demonstrate altered levels of expression of functional proteins in bAVMs.
Studies into hereditary hemorrhagic telangiectasia (HHT) (Rendu-Osler-Weber syndrome) 1 and 2 have provided some insights into the disrupted pathways that may be involved in bAVM formation. Hereditary hemorrhagic telangiectasia is an autosomal dominant condition of vascular dysplasia involving multiple organ systems. The clinical symptoms in HHT are caused by direct arteriovenous connections without an intervening capillary bed. This results in multiple mucocutaneous telangiectasias particularly about the oral cavity, fingertips, and within the nasal cavity. The latter leads to recurrent epistaxis, which is a common presenting symptom. In addition to these superficial telangiectasias, there are visceral AVMs involving, for example, the lungs, liver, and gastrointestinal tract in around one third of patients. Cerebral AVMs are seen in around 10 % of patients; however, they are probably under-recognized [82]. A mutation of endoglin, a component of the transforming growth factor (TGF) receptor complex, is responsible for HHT1 [61, 82]. The gene responsible for HHT2 has also been identified: ALK1, which belongs to the TGF receptor superfamily [61, 82]. Transgenic mice carrying homozygous defects in endoglin die in utero with defective vascular remodeling. Heterozygous mice demonstrate a phenotype consistent with HHT1 including the development of bAVMs [70]. Endoglin appears critical for normal capillary remodeling and extension, being highly expressed during capillary extension prior to the commencement of blood flow. It has been suggested that failure of this process leaves the newly formed capillaries vulnerable to opportunistic micro-shunts at the time of the first blood flow, which, over time, develop into bAVMs [83].
While these genes play a known role in hereditary bAVMs, they may very well play a role in sporadic bAVMs as well. In fact, a study from 2005 found an association between a common polymorphism of the ALK1 and sporadic bAVMs [84], suggesting that these genes may play a larger role in sporadic bAVMs than previously believed. Gene expression studies have identified numerous genes with either increased or decreased levels of expression in bAVMs compared with normal cerebral vasculature [85]. However, the challenge remains to identify potential therapeutic targets. Interestingly, increased VEGF and VEGF receptor expression has been reported following partial embolization of bAVMs [86, 87]. Given VEGF’s role in angiogenesis, this may be central to the dynamic and recurrent nature of bAVMs and is an attractive target for therapeutic intervention in combination with endovascular treatments. Figure 10 depicts a recurrent bAVM 9 years following what was thought to be curative microsurgical resection.
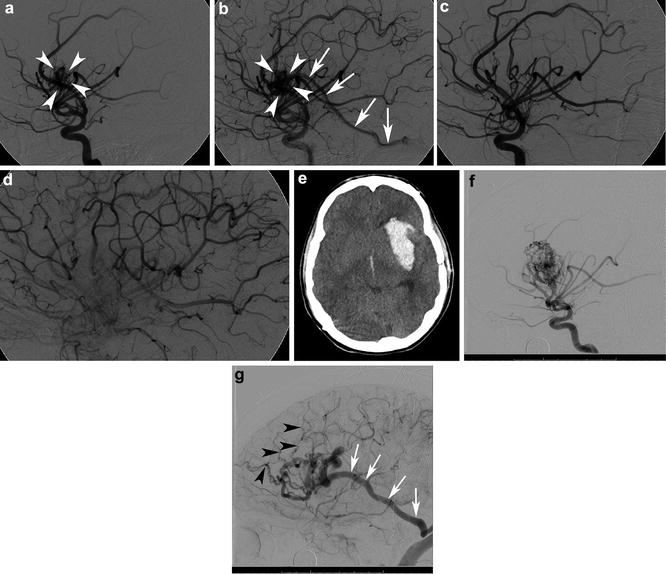
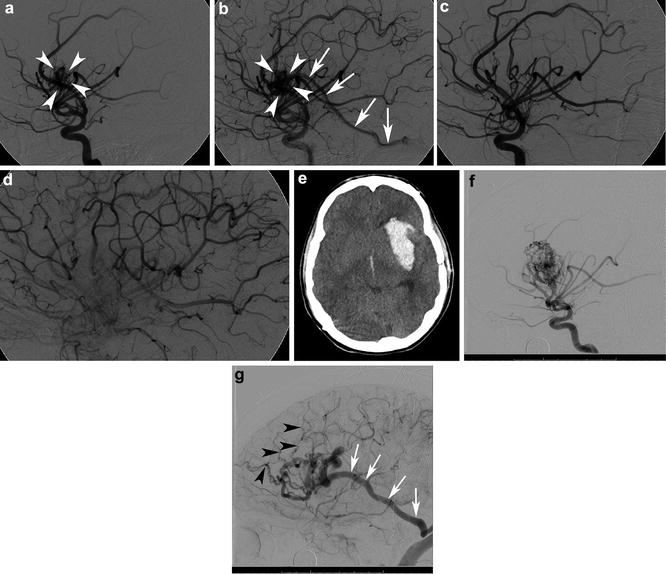
Fig. 10
A 16-year-old male who presented with acute onset of severe headache found to have a 2 cm left insular bAVM. Lateral angiogram in the early arterial (a) and mid arterial (b) phases from a left ICA injection shows a 2 cm left insular bAVM supplied by the middle cerebral artery (MCA) (white arrowheads). Venous drainage is into the vein of Labbe (white arrows). Repeat lateral angiogram following surgical resection of the lesion in the mid arterial phase (c) and late arterial phase (d) shows no nidal opacification or early venous opacification and the lesion was thought to be cured. At the age of 25, the patient sustained a sudden onset of severe headache and loss of consciousness. Noncontrast head CT showed a large parenchymal, subarachnoid, and intraventricular hemorrhage (e). Repeat angiogram (f) (left internal carotid injection in the lateral projection in the early arterial phase) demonstrated a 3.4 cm left insular bAVM. Venous drainage (g) was via Labbe (white arrows) as well and numerous smaller frontal cortical veins (black arrowheads) into the superior sagittal sinus. This was an unusual case of a bAVM that was thought to be cured following microsurgical resection, but which recurred and ruptured 9 years later. Cases such as this one stress the need for interval imaging follow-up in younger patients even in cases that are thought to be cured
Imaging
Whether a bAVM is identified incidentally or due to clinical sequelae such as seizure or focal neurological deficit resulting from hemorrhage or venous congestion, imaging studies establish the diagnosis. Imaging studies in their totality are critical to define simple bAVM characteristics such as location and size as well as establishing more complex characteristics such as drainage pattern, feeding arteries, and the presence of complicating factors such as associated aneurysms. Therefore, an understanding of the concepts already presented and applied to various imaging studies allows the radiologist to provide critical information in regard to the characteristics of a bAVM, thus providing support for clinical decision making as well as therapeutic planning. Every opportunity should be taken to provide more than a simple diagnosis of bAVM and help better understand individual bAVM physiology and procedural risk.
Computed Tomography
Noncontrast computed tomography (CT) is not well suited to assessing the detailed angioarchitectural components critical in making treatment decisions for bAVMs, however, as it is often the first neuroimaging patients have for practical reasons, and therefore every radiologist should be aware of the CT imaging features. In particular, patients who initially present with hemorrhage secondary to bAVM will most likely undergo CT as their first diagnostic procedure, and radiologists should always be cognizant to the possibility of bAVM as the cause of hemorrhage. When intracerebral hemorrhage is encountered in young patients (<45 years old), has a lobar distribution, or is associated with parenchymal calcifications or obvious hyperdense serpiginous structures, bAVM must be considered as a possible etiology [88]. In the case of unruptured bAVMs, slightly hyperdense serpiginous structures may be seen and generally represent draining veins which at their largest are typically quite a bit larger than the largest feeding arteries. Parenchymal calcification can be seen in up to 20 % of bAVM either relating to previous hemorrhage or venous thrombosis (Fig. 11) [80]. Gliosis as a consequence of vascular shunting or recurrent microhemorrhage may be directly identifiable or alternatively may be demonstrated by volume loss and asymmetry. The latter may be obvious or, alternatively, may come to the radiologist’s attention by appreciating asymmetry in the CSF spaces such as the sulci or Sylvian fissure. Ex-vacuo dilatation of ventricular structures may give a clue to the presence of regional parenchymal volume loss. Hydrocephalus may also be present due to mass effect from the nidus itself, or more commonly a prominent draining vein.
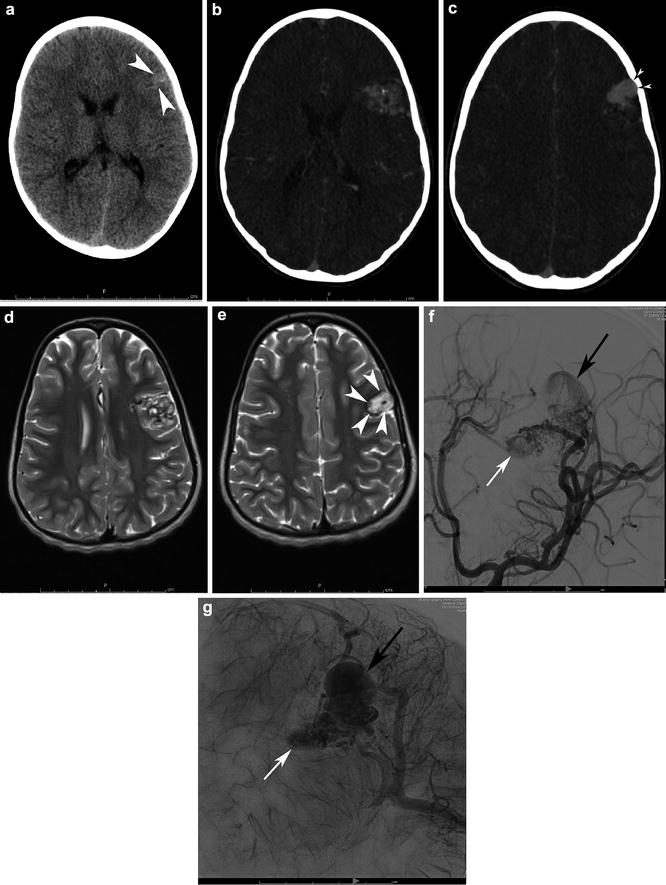
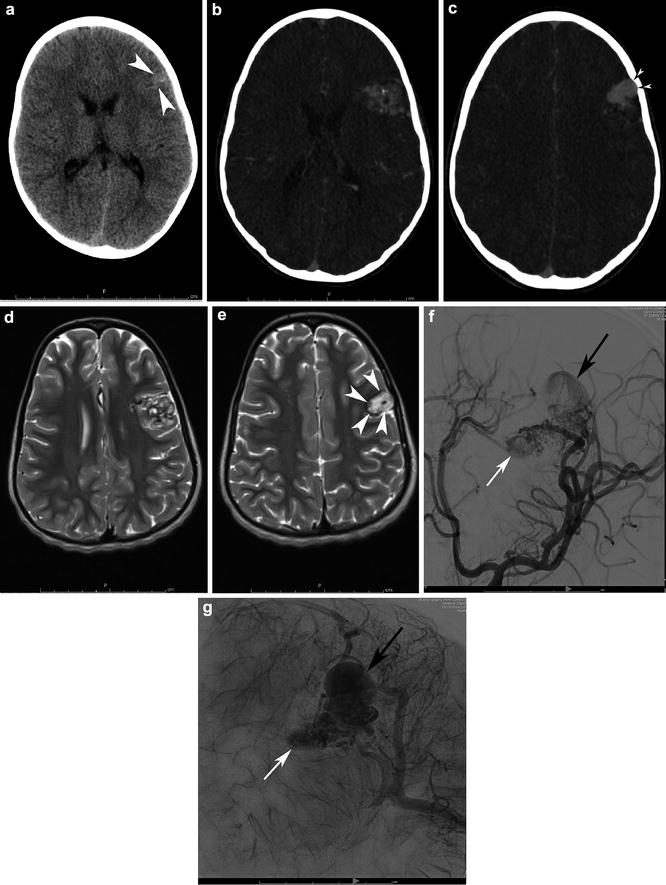
Fig. 11
A 6-year-old girl had a noncontrast head CT for first seizure (a). Note the calcified, slightly hyperdense pyramidal shape structured within the anterior left frontal lobe cortex and subcortical white matter (white arrowheads). Transaxial CTA confirms the diagnosis (b), with a pyramidal complex mesh-like network of the nidus as well as associated features such as abnormal, enlarged draining veins (c). Note the scalloped inner table abutting the venous varix owing to chronicity (black arrowheads with white center). Transaxial T2-WI MRI (d, e) demonstrates the classic flow voids associated with the nidus. Note, however, the high signal within the large venous varix (e) attributable to turbulent and slower flow (white arrowheads). MRI signal characteristics must be interpreted in the context of the flow physiology. Left ICA LAO angiograms in the early arterial and capillary blush phases (f and g) clarify the detailed angioarchitecture of the bAVM. There is direct supply from a trunk of the prefrontal division of left MCA with additional en passant supply from a second trunk. Drainage is entirely superficial, with the deep aspect of the AVM and draining superiorly into the superior sagittal sinus via cortical veins and the superficial aspect draining via cortical veins into the superficial Sylvian territory and out of via the vein of Labbe. There is a 1.5 cm venous varix (small white arrow) associated with the deep aspect of the AVM and a 2.5 cm venous varix (small black arrow) associated with the superficial aspect
CT angiography (CTA) will often establish the diagnosis of bAVM by demonstrating the complex mesh-like network of the nidus and, if flow is sufficient, enlarged arterial feeders and abnormal draining veins. However, as traditional CTA lacks temporal resolution, the key diagnostic feature of bAVMs, namely, arteriovenous shunting, cannot be identified. In the absence of temporal resolution, other intracranial vascular abnormalities must be necessarily considered in the differential diagnosis, specifically developmental venous anomalies (DVAs) (Fig. 12) and capillary telangiectasia. DVAs and capillary telangiectasias have features that may be mistaken for a nidus, and DVAs demonstrate a prominent vein, which may lead to further confusion. Modern CT angiography (CTA) provides excellent spatial resolution allowing characterization of nidal characteristics including size and to a lesser extent intranidal aneurysms, though the spatial resolution remains a fraction of that of conventional angiography (Figs. 1 and 13) [89].
