1. Coma (excluding spinal cord-mediated reflexes)
2. Absence of brain stem reflexes
(a) Pupils mid-position or greater and absent pupillary light reflex (fixed dilated pupils)
(b) Corneal
(c) Gag/pharyngeal
(d) Cough/tracheal
(e) Vestibulo-ocular (“cold caloric”)
(f) Loss of central drive to breathe, assessed with apnea test
To confirm brain death according to clinical criteria, prerequisites should be fulfilled prior to the application of the diagnostic tests – see Table 2. Most countries mandate that the clinical diagnosis be confirmed by more than one physician, either two or three.
Table 2
Preconditions: patient-related prerequisites that should be fulfilled prior to application of diagnostic tests
1. Established etiology and/or structural lesion capable of causing death by neurological criteriaa |
2. Reduced consciousness (as measured by GCS 3–5) |
3. Evidence for progressing loss of brain stem function |
The Role of Imaging Tests in Determination of Brain Death
There are known circumstances during which a clinical examination to confirm brain death may become unreliable or cannot be completely performed. These confounding conditions that may impede or invalidate clinical diagnosis of brain death are listed in Table 3.
Table 3
Confounding conditions that may impede or invalidate the clinical diagnosis of brain death
1. Trauma to the eyes |
2. Trauma to the middle and/or inner ears |
3. Nerve or muscle dysfunction or neuromuscular blockade potentially accounting for unresponsiveness |
4. Presence of atypical responses |
5. Unresuscitated shock |
6. Acquired or therapeutic hypothermia |
7. Severe metabolic and endocrine abnormality that is difficult to reverse (e.g., glucose, acid-base, electrolytes) |
8. Clinically significant drug intoxications (e.g., alcohol, barbiturates, sedatives, hypnotics) |
9. Inability to perform apnea test |
10. High cervical spine injury |
11. Primary infratentorial brain injury |
12. Young children |
In situations in which clinical testing cannot be performed or when uncertainty exists about the reliability of its parts due to confounding conditions, ancillary tests (i.e., imaging studies) may be useful. Ancillary tests are applied as complementary to clinical testing that otherwise, for any reason, cannot be conducted or is unreliable. Furthermore, the usage of ancillary tests can reduce the time taken to confirm the diagnosis and increase its confidence in all cases of suspected brain death regardless of the presence of confounding conditions. Early determination of brain death may allow for avoidance of protracted stays in the intensive care unit (ICU) and potentially expedite organ donation before tissue viability becomes a concern. In some protocols, ancillary tests are used to shorten the duration of the observation period.
The objective of ancillary tests in the diagnosis of brain death is to demonstrate the absence of cerebral electrical activity (EEG and evoked potentials) or cerebral circulatory arrest. Currently, only catheter cerebral angiography and perfusion scintigraphy are widely accepted as ancillary tests in the determination of cerebral circulatory arrest. However, in clinical practice transcranial Doppler sonography (TCD), CT angiography (CTA), and MR angiography (MRA) are used as well. Other methods, like perfusion CT (PCT), xenon CT (XeCT), MR spectroscopy (MRS), diffusion-weighted MRI (DWI), and functional MRI (fMRI), are being studied as potentially useful in the diagnosis of brain death.
Pathophysiological Mechanism of Cerebral Circulatory Arrest
Cerebral blood flow (CBF) is continuously regulated by cerebral perfusion pressure (CPP) and cerebrovascular resistance (CVR). CPP is measured as a difference between the entry pressure, mean arterial pressure (MAP), and the exit pressure – intracranial pressure (ICP). According to the Poiseuille equation:
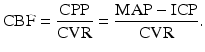
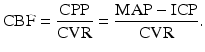
The normal CBF at rest is maintained within a range of 45–60 ml/100 g of brain tissue/min. A brain injury is accompanied by cerebral edema. Because the skull bones limit the intracranial volume, this “mass effect” leads to the intracranial hypertension. The process spreads from the region of primary injury gradually involving the whole brain, and the intracranial vessels become compressed but preserve their patency. Initially, CVR is kept almost constant by the activated autoregulation mechanisms releasing blood vessel tone. However, this phenomenon is capable to preserve a sufficient blood supply as long as CPP exceeds approx. 50 mmHg – see Fig. 1.
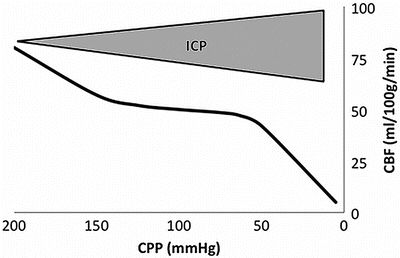
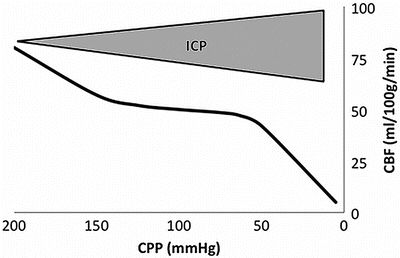
Fig. 1
Relationship between cerebral blood flow (CBF) and cerebral perfusion pressure (CPP). CPP is measured as a difference between the entry pressure, mean arterial pressure (MAP), and the exit pressure, intracranial pressure (ICP). In increasing ICP the cerebral autoregulation is capable to preserve almost constant CBF as long as CPP exceeds approx. 50 mmHg. Further elevation of ICP results in deterioration of cerebral blood flow leading to cerebral circulatory arrest
Further elevation of ICP results in an increase of CVR. The capillaries as the most susceptible vessels are primarily affected; then the process spreads proximally progressively involving bigger arteries. As ICP reaches the diastolic pressure, the end-diastolic cerebral flow completely ceases. At this stage CBF is deeply compromised, although still sufficient to preserve a viability of the brain. When ICP exceeds the diastolic pressure approaching MAP, the circulatory arrest occurs in the compressed cerebral capillaries and venules. At this time larger arteries stay patent, and their elastic walls are distended by the blood pushed forward by the heartbeat in systolic phase (antegrade flow). In diastole a similar blood volume is pressed out of the collapsed arteries (retrograde flow). Such an oscillating, to-and-fro movement of the blood provides the zero-net CBF. This is an early phase of cerebral circulatory arrest leading to necrosis of neurons within 10–15 min. With further ICP elevation, the oscillations of blood column in the proximal arteries become weaker. If ICP exceeds the level of systolic pressure, a blood movement in the cerebral vessels ceases completely, while oscillation can be still observed in extracranial segments of the internal carotid arteries (ICAs) and vertebral arteries (VAs).
Imaging of Structural Changes in Brain Death
CT
Non-contrast-enhanced CT (NECT) of the head is useful for assessing the severity of brain injury in the suspicion of brain death. The study may reveal the features of cerebral edema including a generalized hypodensity of brain parenchyma, decreased differentiation between the gray and white matter (GM:WM ratio), sulcal effacement, absence of the basal cisterns, and signs of brain herniation – see Fig. 2. It was shown that a midline shift >10 mm, absence of the ambient cistern, and GM:WM ratio <1.18 predict progression to brain death. Although the study is ubiquitous, noninvasive, non-time-consuming, and non-cost consuming, it lacks sufficient diagnostic value as an ancillary test.
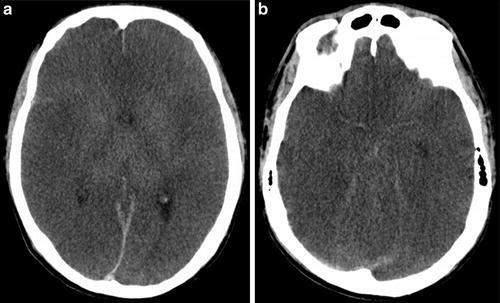
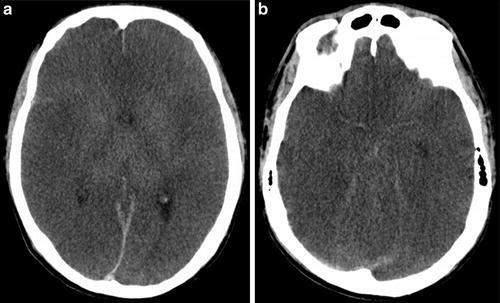
Fig. 2
CT findings in a 23-year-old man after cardiac arrest who presented with clinical signs of brain death: (a, b) 5 mm MPRs in axial plane show diffuse edema, sulcal effacement, ventricular narrowing, low GM/WM differentiation, and diminution of the basal cisterns
MRI
The advent of magnetic resonance imaging has improved diagnostic possibilities in brain death, due to several sophisticated techniques offered by MRI.
In the early 1990s, first reports demonstrated the potential role of MRI in the diagnosis of brain death, including MR spectroscopy [2, 3].
Common MRI findings in echo-planar scans are variable edema, including diffuse gyral swelling, most prominent in the acute phase, diffuse cerebral white matter injury, diffuse cortical high signal intensity, and tonsillar herniation [4–6]. Some authors observed also transtentorial herniation [4, 7, 8]. Less frequent finding is focal edema in the upper spinal cord [5].
Poor gray/white matter differentiation after the diagnosis of brain death is not commonly observed. Matsumura et al. [8] found significant increasing of the ratio of signal intensities between white and gray matters on PD- and T2-WI images but decreased on T1-WI images. These phenomena may be related to different vulnerabilities of white and gray matter to anoxic injury – see Fig. 3a, c.
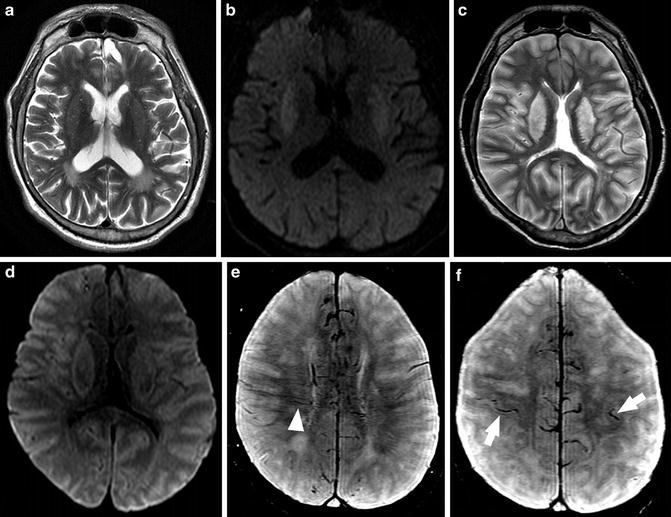
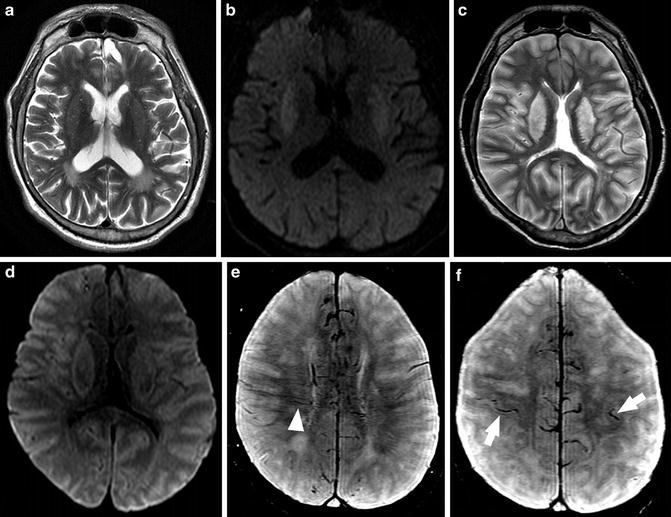
Fig. 3
MR findings in a 42-year-old man after cardiac arrest who presented with clinical signs of brain death. First examination performed several hours after resuscitation: (a) 5 mm axial T2-WI image (TR = 3,713 ms, TE = 104 ms) shows moderate increase in signal of lentiform nuclei consistent with edema; (b) DWI (TR = 5,000 ms, TE = 86 ms) shows restricted diffusion in lentiform nuclei. Second examination performed the next day: (c) 5 mm axial T2-WI image (TR = 3,713 ms, TE = 104 ms) shows massive edema involving the basal ganglia and cortex with narrowing of ventricles; (d) DWI (TR = 5,000 ms, TE = 86 ms) shows restricted diffusion in lentiform nuclei and cortex. Cortical and deep GM are more frequently affected because neurons are more vulnerable to hypoxia than oligodendroglia or astrocytes; (e, f) SWI (TR = 73 ms, TE = 47 ms) shows multiple and branching low signal intensities extending through the cerebral hemisphere parallel or perpendicular to the outer wall of both lateral ventricles (arrowhead, transcerebral vein sign) and abnormal low signal intensities in both cerebral hemisphere cortical areas (arrows, bilateral cortical vein sign)
They also showed the absence of normal flow voids in MR echo-planar images. Moreover, with serial MR before and after the diagnosis of brain death, they demonstrated disappearance of flow voids in cavernous portions of the ICAs after the diagnosis. The disappearance of flow voids in the ICA correlates with nonfilling of the ICA revealed with intra-arterial digital subtraction arteriography [8]. Sohn et al. [9] using 3-T MRI demonstrated that tonsillar herniation and loss of intra-arterial flow signal voids on T2-WI are highly sensitive and specific findings in patients with brain death.
Additional signs include the bilateral transcerebral vein sign, defined as branching structures extending through cerebral hemispheres parallel or perpendicular to the outer wall of a lateral ventricle, and the bilateral cortical vein sign, defined as visualization of cortical veins of cerebral hemispheres. Both signs can be easily demonstrated with T2*WI gradient-recalled echo (GRE) and susceptibility-weighted images (SWI) [9] – see Fig. 3e, f.
Diffusion-Weighted Imaging
Lövblad et al. first demonstrated the usefulness of diffusion-weighted images (DWI) in the diagnosis of brain death. They found a significant drop in the apparent diffusion coefficient (ADC) values compared with those of normal controls, and these observations have been later confirmed by other authors [7, 10, 11]. With DWI and ADC mapping, it is possible to identify areas corresponding to cytotoxic edema and ischemic damage – see Fig. 3b, d.
Selcuk et al. [11] observed greater decrease in ADC values in white matter than in gray matter for both the cerebral and cerebellar hemispheres. The sensitivity and specificity as well as positive and negative predictive cutoff values of ADC to distinguish patients with brain death from controls were 100 % in their study, but they recommend to determine ADC cutoff values for different MRI scanners and DWI protocols. They believe that a 100 % sensitivity and specificity in their results indicates a potential role of ADC maps as confirmatory test in diagnosis of brain death.
However, Luchtmann et al. evaluating the reliability of DWI in the diagnosis of brain death concluded that effects of pseudonormalization of ADC values (after 5–28 days after brain ischemic damage) and possible susceptibility artifacts due to microhemorrhages preclude a leading role of DWI in the diagnosis of brain death [10].
MR Spectroscopy
Other sophisticated technique offered by MRI is MR spectroscopy (MRS), allowing to study metabolic changes in brain tissue. In previously used method of 31P MRS, the most striking feature was a complete absence of adenosine triphosphate (ATP) and domination of intense inorganic phosphate signal [2, 3]. Kato et al. [3] observed also an absence of phosphocreatine and stated that MRS may be helpful in diagnostics of brain death particularly in children, when other examinations are not sufficient to establish defined diagnosis.
Falini et al. [12] performing sequential MR imaging and proton (1H) MRS in a patient with severe hypoxic-ischemic brain injury found that observed structural and biochemical changes reflected the known evolution of brain degeneration after asphyxial injury, particularly the massive decrease of cortical N-acetylaspartate in the acute phase, suggesting the severity of the neuronal damage, and the subsequent progressive increase of choline related to the delayed degeneration of white matter.
To our best knowledge, no other study on application of MRS in human brain death is available.
Functional Imaging of Brain Death
The future perspectives of imaging of the brain in comatose patients may be linked to functional MRI (fMRI) with blood oxygen level-dependent (BOLD) signal. In 2009 Boly et al. [13] first reported that fMRI showed no significant functional connectivity in a brain death patient in contrary to a vegetative state patient with preserved cortico-cortical connectivity. In 2010 Monti et al. [14] successfully elaborated new methodology, which can be applied in fMRI in a patient without conscience. With imagery tasks they were able to establish functional and interactive communication with a minority of patients who met the behavioral criteria for a vegetative state after serious brain injury, detecting residual cognitive function in them. For this reason fMRI probably will provide a method for detecting minimal cognitive ability in patients without a behavioral response and could be considered a complementary tool for existing diagnostic methods. Hence, it can be believed that fMRI will increase significantly our understanding of conscience disorders in patients with severe brain damage and differentiate with the highest accuracy brain death from a vegetative state or chronic coma.
From the theoretical point of view, MRI might seem an ideal confirmatory test in brain-dead patients because it is able to prove the cessation of cerebral blood flow (MRA) and to demonstrate the irreversible loss of cerebral bioelectrical activity (fMRI). However, practical application of MRI in these patients is limited because transportation and monitoring of ventilated patients to an MRI scanner can be challenging, and the procedure is time-consuming. On the other hand, in chosen cases MRI may add certainty to the diagnosis of brain death in addition to clinical findings. Further extensive studies are needed to establish a potential role of MRI techniques, including MRA, DWI, MRS, and fMRI, in the diagnostic algorithm for brain death.
Imaging of Cerebral Circulatory Arrest
Prerequisites for Blood Flow Studies in the Diagnosis of Brain Death
It is recommended that blood flow studies be performed when it is impossible to complete the clinical criteria defined in Table 1. Before these tests are performed, at least two particular clinical criteria must be met:
1.
An established etiology capable of causing neurological death in the absence of reversible conditions capable of mimicking neurological death
2.
Deep unresponsive coma
Prior to the testing, unresuscitated shock and hypothermia must be corrected.
The blood flow studies are not confounded by drugs or metabolic disorders, so they are reliable in cases of barbiturate overdose.
Catheter Cerebral Angiography
Catheter cerebral angiography is considered as a reference method in the diagnosis of majority of cerebrovascular pathologies including cerebral circulatory arrest. For the diagnosis of brain death, it was used as the first imaging modality in 1953.
The study involves a follow-up of iodinated contrast dynamics after its intravascular injection on time-resolved images made with the usage of the digital subtraction technique (DSA).
Catheter Cerebral Angiography Findings in Intracranial Hypertension and Brain Death
At raising ICP the cerebral arteries and veins fill with contrast with increasing delay in comparison to extracranial vessels of the head. At the early stage of cerebral circulatory arrest, nonfilling of the cortical arterial branches and the deep cerebral veins (the internal cerebral vein, ICV; the great cerebral vein, GCV; and the straight sinus) occurs. At this stage an oscillation of contrast column in the proximal intracranial arteries may be detected. The pendulum movement of the contrast is accompanied by its slow antegrade propagation and intracranial stasis. The contrast is gradually eliminated from the intracranial vessels through the arteriovenous shunts and the interrupted blood-brain barrier. Noteworthy the superior sagittal sinus and the transverse sinuses may fill from the extracranial vessels through the emissary veins. With further increasing ICP, the contrast column is stopped in more proximal arterial segments. A total intracranial nonfilling occurs when the ICP reaches systolic blood pressure.
Technique of Catheter Cerebral Angiography in the Diagnosis of Brain Death
Catheter cerebral angiography for the determination of brain death is performed with an injection of iodinated contrast material to reach both anterior and posterior cerebral circulation. The propagation of contrast is assessed in a filming sequence acquired with the usage of DSA technique. The duration of the acquisition varies among national guidelines, but it should not be shorter than 15 s to confirm cerebral circulatory arrest (personal experience).
The national guidelines permit two different techniques of contrast injection:
1.
Aortocervical arteriography – injection from the aortic arch
2.
Selective 4-vessel cerebral angiography, performed with a selective catheterization of both ICAs or the common carotid arteries (CCAs) and VAs
While performing the selective angiography, one must take into account that the contrast injected under high pressure into the ICA or VA may be pushed forward to intracranial arteries resulting in an artifactual intracranial filling. This can be avoided if the injection from the aortic arch is applied. Moreover, the selective technique requires higher manual skills and experience than aortic arch angiography. Finally, the risk of procedure-related complications is higher for selective technique. Because both techniques are characterized by equally high accuracy, the aortic arch angiography is a preferred option.
Intravenous angiography can be applied in the determination of cerebral circulatory arrest as well [15]. However, when performing the exam with i.v. injection, one must consider the jugular and dural venous sinus reflux of contrast. Such finding is not indicative of preserved cerebral circulation [15]. Another disadvantage of this technique is a low concentration of contrast achieved in the head and neck vessels due to the effect of dilution. This could make the detection of possible intracranial opacification equivocal. Therefore arterial injection is preferred.
Criteria for the Diagnosis of Brain Death in Catheter Cerebral Angiography
Cerebral circulatory arrest can be documented by catheter cerebral angiography if all of the following conditions are met:
1.
Filling of external carotid arteries confirming a proper contrast delivery
2.
No filling of ICAs beyond the level of the anterior clinoid process
3.
No filling of VAs beyond their dural penetration
4.
No filling of the internal cerebral veins [16] (see Fig. 4)
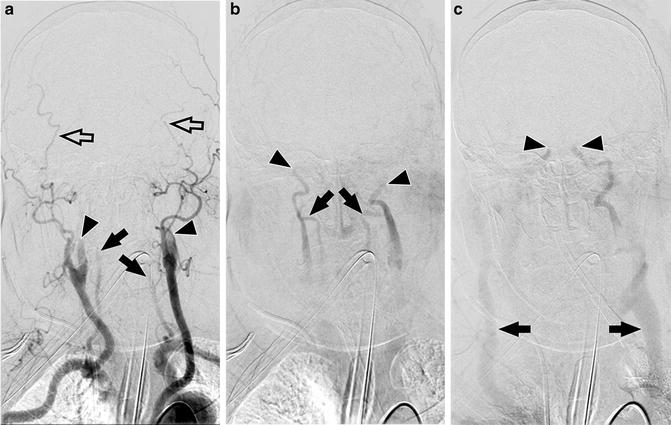
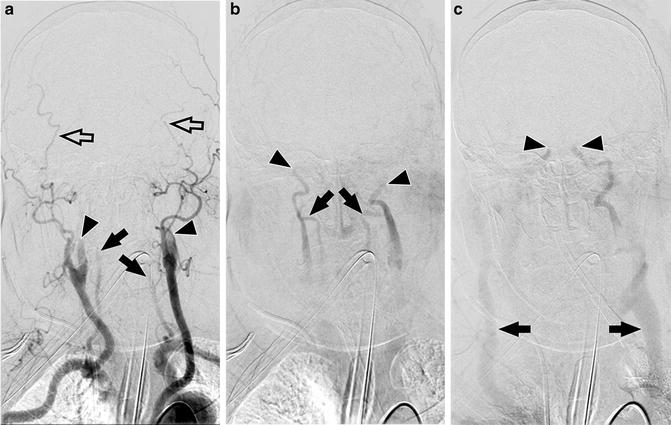
Fig. 4
Catheter angiography findings in a 74-year-old woman after traumatic brain injury who presented with clinical signs of brain death. Aortic arch injection of 30 ml of contrast at flow rate of 15 ml/s. (a) 3 s after injection branches of the external carotid arteries are filled (empty arrows), while filling of the internal carotid arteries (arrowheads) and the vertebral arteries (arrows) is limited to their proximal extracranial parts; (b) 10 s after injection slow propagation of contrast is visible in extracranial parts of the ICAs (arrowheads) and the VAs (arrows); (c) 15 s after injection contrast reaches cavernous segments of the ICAs (arrowheads). Venous outflow through the external jugular veins is observed (arrows). No intracranial filling is noted. These findings are consistent with the diagnosis of brain death
However, delayed opacification of proximal segments of the cerebral arteries with contrast stasis and without deep venous filling does not preclude the diagnosis of brain death – see Fig. 5.
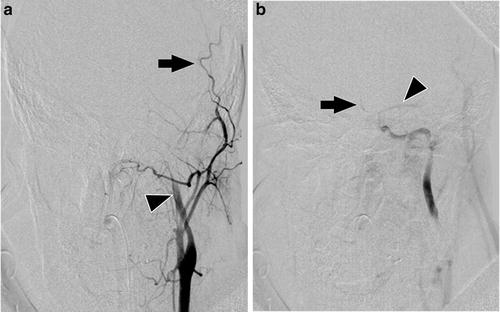
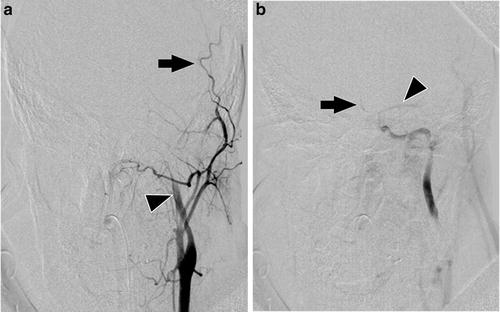
Fig. 5
Catheter angiography findings in an 18-year-old man after traumatic brain injury who presented with clinical signs of brain death. Selective injection of 8 ml of contrast at flow rate of 6 ml/s to the left common carotid artery. (a) 3 s after injection branches of the external carotid artery are filled (arrow), while filling of the ICA (arrowhead) is limited to its proximal extracranial part; (b) 12 s after injection stasis of contrast is visible in the M1 segment of the middle cerebral artery (arrowhead) and A1 and A2 segments of the anterior cerebral artery (arrow). No intracranial venous outflow is noted. These findings are consistent with the diagnosis of brain death
Usefulness of Catheter Cerebral Angiography in the Diagnosis of Brain Death
Catheter angiography is widely accepted as a reference test in the diagnosis of brain death. Despite this several disadvantages limit its application. The catheterization of aortic arch, especially ICAs and VAs, requires high expertise. The exam must be performed in the angiographic lab; thus, transportation of a critically ill patient is necessary. The procedure is invasive, accompanied by the risk of serious complications, such as an injury of a catheterized vessel or vasospasm. Besides catheter angiography is the most costly procedure among the blood flow tests. Potential risk of a damage of transplantable organs caused by iodinated contrast, although postulated, has not been confirmed yet. Moreover, it was revealed that contrast medium administration to donors does not affect kidney graft function after transplant [17]. No false-positive results of catheter angiography in the diagnosis of brain death have been reported (i.e., angiography revealed cerebral circulatory arrest, yet the patient survived), which means the 100 % specificity. The sensitivity is close to 100 % as well. There are casuistic reports of false-negative results in cases in which residual preservation or restoration of intracranial blood flow was observed after ICP was relieved through some decompressive mechanism, e.g., craniectomy or skull fractures (see “Limitations” section) [15]. There are several reports of preserved blood flow in the posterior fossa with cerebral circulatory arrest in the anterior circulation [15, 18]. This points to the protective function of the cerebellar tentorium, which causes an uneven increase in ICP. In all false-negative exams, patients met all clinical criteria of brain death, and in all cases intracranial circulation was markedly slowed.
CT Angiography
CTA for the determination of brain death was proposed in 1998 [19]. Despite the fact that technical protocol or evaluation criteria have not been standardized yet, CTA is considered the most promising ancillary test.
CT Angiography Findings in Brain Death
Unfortunately CTA findings were not studied in non-brain-dead patients with intracranial hypertension. The earliest sign of cerebral circulatory arrest in CTA is a lack of opacification of deep veins – the ICVs and the GCV. The sensitivity of this finding in the diagnosis of brain death in CTA is 98–100 % [19–23]. A lack of opacification of cortical branches of the middle cerebral arteries (MCA-M4) is slightly less sensitive indicator of brain death with the sensitivity of 86–100 % [19, 21, 22]. The basilar artery (BA) and cortical branches of the posterior cerebral arteries (PCA-P2) are more frequently opacified in brain-dead patients than MCA-M4 – see Fig. 6. Their sensitivity is 85–94 % and 79 %, respectively [19, 21]. The least sensitive finding of brain death is a lack of opacification of cortical branches of the anterior cerebral arteries (ACA-A3, the pericallosal arteries) with the sensitivity of 64 % [21] – see Fig. 7. Such a sequence is explained by the highest susceptibility of cortical branches of the MCA to intracranial hypertension and the lowest CPP in these arteries. In the course of cerebral circulatory arrest, proximal segments of the cerebral arteries frequently show delayed opacification for some time. These vessels appear as thin and faint. The intracranial stasis of contrast is observed with its extremely slow elimination through the arteriovenous shunts and extravasation due to the interrupted blood-brain barrier. The latest sign of cerebral circulatory arrest is intracranial nonfilling – see Fig. 8.
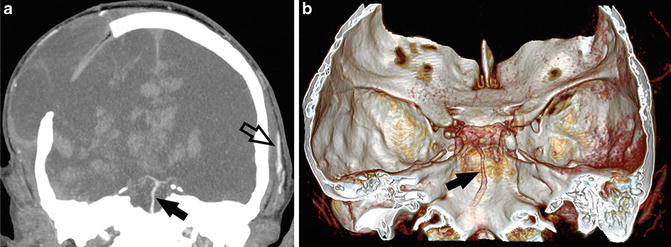
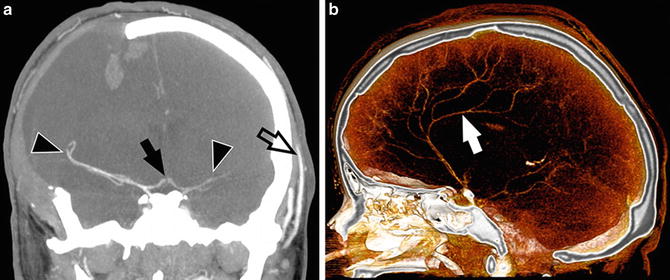
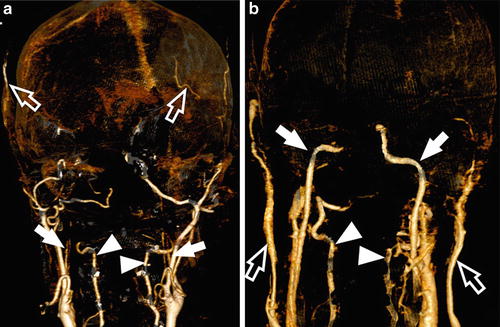
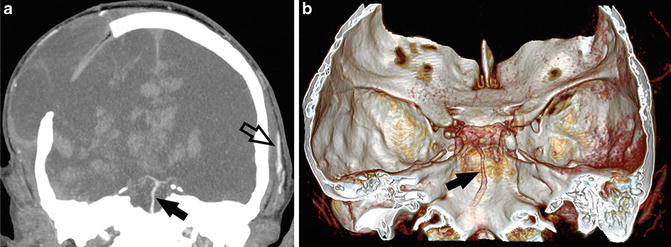
Fig. 6
CTA findings in a 50-year-old man with traumatic brain injury and right-sided craniectomy presented with signs of brain death: 10 mm MIP in coronal plane (a) and VRT (b) with a delay of 60 s after injection show opacification of the basilar artery (arrows). No filling of the anterior cerebral circulation or deep venous outflow is noted. Opacification of the superficial temporal artery (empty arrow) indicates that the contrast was injected properly. In some cases of brain death, the stasis of contrast in the posterior circulation may be observed due to the protective function of cerebellar tentorium
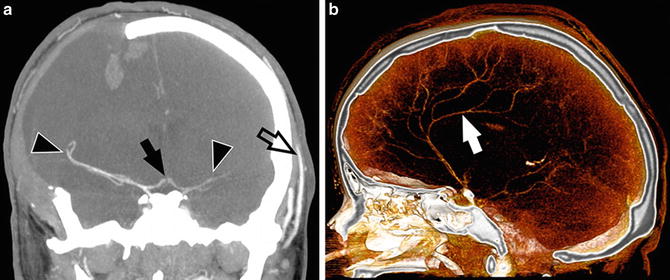
Fig. 7
CTA findings in a 22-year-old woman with a brain stem ischemic stroke and a right-sided craniectomy who presented with signs of brain death on clinical examination. (a) 10 mm MIP in coronal plane with a delay of 60 s after injection shows opacification of M1 and M2 segments of the middle cerebral arteries (arrowheads) and A1 segments of the anterior cerebral arteries (arrow); (b) sagittal VRT shows opacification of the right pericallosal artery (arrow). No filling of the basilar artery or deep venous outflow is noted. In some cases of brain death, the stasis of contrast in the pericallosal arteries may be observed because of higher CPP in these arteries in comparison to cortical branches of the middle cerebral arteries
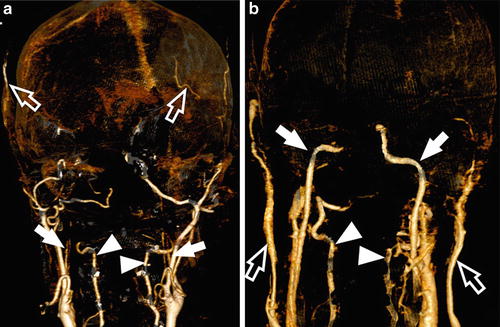
Fig. 8
CTA findings consistent with the diagnosis of brain death: (a) VRT 20 s after contrast injection shows filling of the ICAs (arrows) and the VAs (arrowheads) limited to their proximal extracranial parts. Opacification of extracranial arteries (empty arrows) indicates that the contrast was injected properly; (b) VRT 60 s after contrast injection shows slow propagation of contrast to the level of petrous segment of the right ICA and cavernous segment of the left ICA (arrows). Vertebral arteries are opacified to the level of foramen magnum, before their dural penetration (arrowheads). Venous outflow through external jugular veins is observed (empty arrows). No intracranial filling was noted
Technique of CT Angiography in the Diagnosis of Brain Death
The technique of CTA involves a rapid, intravenous administration of iodinated contrast in the bolus at a flow rate of 3–5 ml/s. Volume of contrast medium used for CTA for the diagnosis of cerebral circulatory arrest is 60–120 ml. The contrast can be followed by the infusion of 30–40 ml of normal saline at the same rate (i.e., saline flush) to push the contrast medium forward and to optimize contrast enhancement.
For the diagnosis of brain death, at least three acquisitions should be performed:
1.
Non-enhanced scanning as a reference for assessing vascular opacification.
2.
Early post-contrast scanning for assessing intra- and, more important, extracranial vascular opacification. This phase is started approx. 20 s after the beginning of injection. Opacification of branches of external carotid arteries – the superficial temporal or the facial arteries – indicates that the contrast was injected correctly, and there are no hemodynamic abnormalities causing a delay of contrast delivery to the vessels of head and neck. To ensure scanning is performed during the phase of optimal contrast enhancement, several strategies can be applied. A fixed delay is the easiest but least favorable option since it does not adjust for individual variations in contrast dynamics. Two more advanced strategies, test bolus injection and bolus tracking technique, can be applied to tailor the contrast injection to the individual patient.
3.
Late post-contrast scanning for assessing intracranial vascular opacification. This phase is started 60 s after the beginning of injection, with a delay of 40 s to the early phase. The necessity of performing the late phase of CTA in the diagnosis of cerebral circulatory arrest is motivated by a possible delayed intracranial opacification in increased ICP. Termination of the study on the early phase may result in a failure to recognize delayed intracranial filling producing a false-positive result.
Intermediate post-contrast scans may be added between the early and the late phase for a better follow-up of the contrast dynamics.
Practical Tips
The superficial temporal artery (STA) is routinely used for assessing sufficient contrast delivery. But in some cases, both STAs may be surgically ligated during bilateral temporal craniectomy. In such cases the facial artery can be used as a good alternative.
In patients with suspected brain death, CT images often show subarachnoid hemorrhage (SAH) or high-attenuation areas along the basal cisterns and cortical sulci, mimicking SAH. The latter is described as pseudo-SAH phenomenon, which is a synergistic result of distention of congested superficial veins due to elevated intracranial pressure and severe brain edema. Both true SAH and pseudo-SAH impede the assessment of intracranial filling considering that cerebral vessels in intracranial hypertension are usually abnormally thin and faintly opacified. Therefore cerebral vessels should be assessed on pre- and post-contrast scans.
Another potential difficulty associated with CTA assessment is a consequence of frequently found midline shift and marked ventricular displacement. This may cause problems with identification of the dislocated deep cerebral veins on routinely used axial images. These vessels can be far easier localized on sagittal maximum intensity projections (MIPs).
Criteria for the Diagnosis of Brain Death in CT Angiography
There are no widely accepted criteria of CTA in the diagnosis of brain death. Four methods of evaluation have been proposed so far; they are summarized in Table 4 and Fig. 9.
Table 4
Criteria for the diagnosis of brain death in CT angiography
Criteria | Lack of opacification of | Sensitivity (%) |
---|---|---|
ICA beyond the level of the anterior clinoid process | 86–92a | |
VA beyond their dural penetration | ||
ICV, GCV, and the straight sinus | ||
10 point [26] | BA | 54–70 |
Right and left PCA-P2 | ||
Right and left ACA-A3 (pericallosal artery) | ||
Right and left MCA-M4 | ||
Right and left ICV | ||
GCV | ||
![]() Stay updated, free articles. Join our Telegram channel![]() Full access? Get Clinical Tree![]() ![]() ![]() |