Fig. 1
Longitudinal color (a, b) and power (c) Doppler ultrasound image demonstrating a hypoechoic thickened wall, a finding consistent with a mural hematoma (a) narrowing and displacing the true lumen (b) of the ICA with enlargement of its external diameter to 7 mm (normal 5 mm). An abnormal high-resistance spectral Doppler US waveform is demonstrated in the dissected lumen (d)
Spectral Doppler US waveforms in CAD can be normal or may demonstrate nonspecific features also occurring in high-grade stenosis and occlusions (damped spectral waveform with lower amplitude and biphasic pattern, high-resistance spectral waveform, or absence of flow with no spectral Doppler waveform) [54].
The diagnostic sensitivity of US decreased if CAD results in a low-grade stenosis [54]. Sturzenegger et al. showed high accuracy in detecting a dissection with US in case of ICA occlusion or high-grade stenosis but a 20 % sensitivity in low-grade stenosis [49]. Another limitation is that US is less reliable for CAD outside the “window” of the US examination such as dissections located in the subpetrous segment and the carotid canal or in case of small mural hematoma [54, 55].
Cross-Sectional Imaging: CT or MRI?
MRI, including MR angiography (MRA) and high-resolution MRI (HR-MRI), and CT angiography (CTA) are the main noninvasive cross-sectional imaging tools used for assessing CAD. The use of MRI and CTA is twofold: (1) demonstration of a mural hematoma and (2) evaluation of the consequence of the mural hematoma on the lumen patency. MRI is currently the recommended imaging technique [5], thanks to its higher ability to demonstrate arterial wall abnormalities and its better specificity as compared with CTA. CTA can be performed as a first-line screening tool, particularly in case of trauma.
Magnetic Resonance Imaging
Dissections of the craniocervical arteries are best assessed with cross-sectional fat-suppressed T1 sequence with cranial and caudal saturation pulses and with MRA techniques. The former can provide direct visualization of the mural hematoma, which is the pathological hallmark of a dissection, and the latter allows noninvasive visualization of arteries.
Lumen Imaging: MR Angiography
Contrast-enhanced MRA (CE-MRA) is the technique of choice, thanks to recent improvements in MR gradients and software, which allow rapid acquisitions with centric k-space filling and automatic bolus triggering. Like DSA, CE-MRA can demonstrate luminal irregularity; long narrowing of the vessel, the so-called string sign; or occlusion and pseudoaneurysm formation (Fig. 2). CE-MRA also has the advantage of being easily preceded with fat-suppressed T1-WI axial images through narrowed segments to confirm or refute a suspected dissection. The combination of cross-sectional MRI and MRA is not only a powerful diagnostic modality but can also be used effectively for noninvasive follow-up of CAD to monitor lumen patency evolution, resolution of a mural hematoma, or development of complications (Figs. 3, 4, and 5).
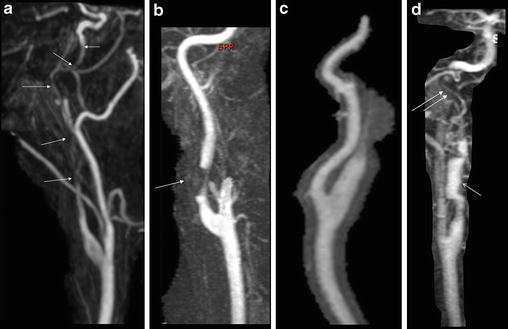
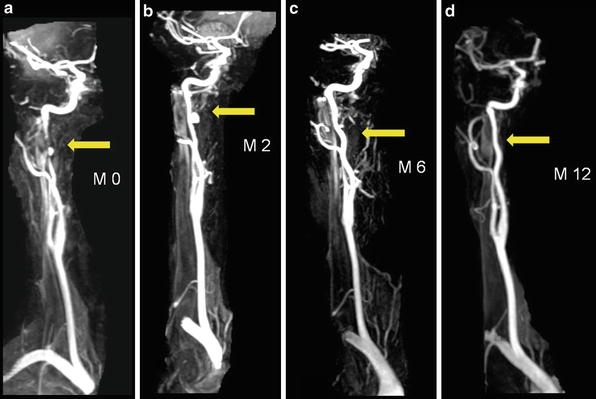
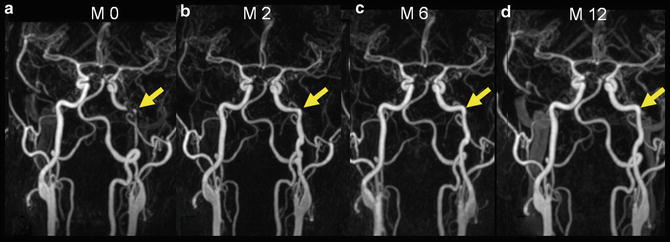
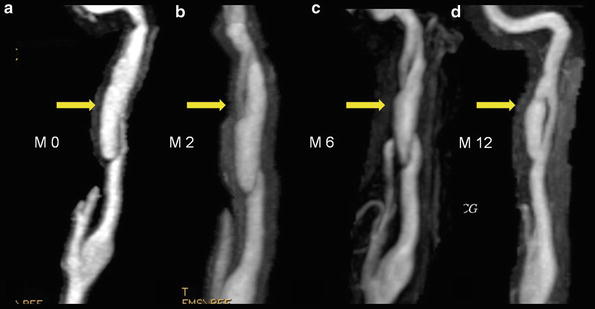
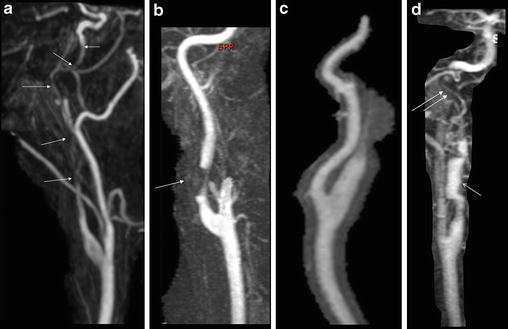
Fig. 2
Luminal patterns of carotid artery dissection on contrast-enhanced MR angiography (CE-MRA). (a, b) Stenotic type of CAD. In (a) CE-MRA demonstrates a long, tapered, eccentric, and irregular stenosis (arrows) that begins beyond the ICA bulb and that extends to the skull base, the “string” sign. In (b) focal eccentric stenosis (arrow) that begins beyond the ICA bulb, with a distal dilatation, the “string and pearl” sign. (c) Occlusive pattern of CAD. Flame-shaped tapering followed by an occlusion of the lumen that spares the carotid bulb. (d) Pseudoaneurysmal pattern of CAD (arrow) associated with a distal long-tapered stenosis (double arrows)
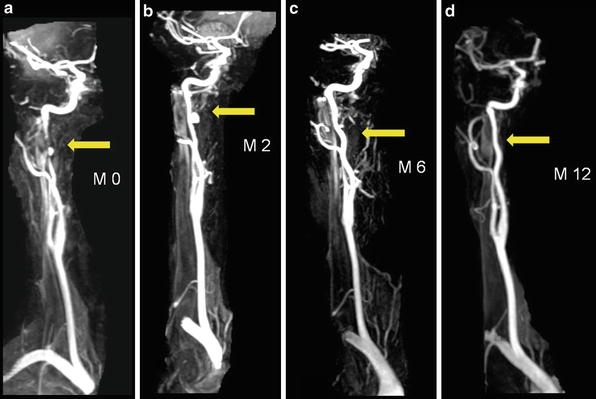
Fig. 3
Evolution of a pseudoaneurysm type of carotid artery dissection. The pseudoaneurysm (arrow) was initially associated with a focal-tapered stenosis (a). Resolution of the stenosis associated with a progression of the pseudoaneurysm 2 months after onset of the CAD (b). Progressive normalization seen at 6 and 12 months (c, d)
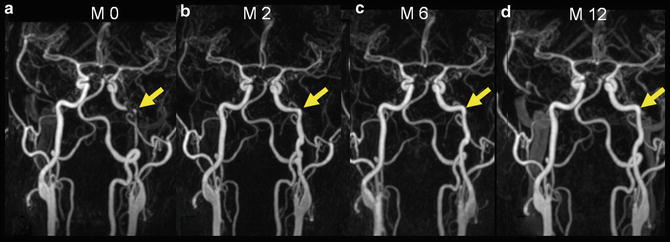
Fig. 4
Evolution of a stenotic type of carotid artery dissection. The long, irregular stenosis (arrow) improves with progressive normalization seen at 12 months
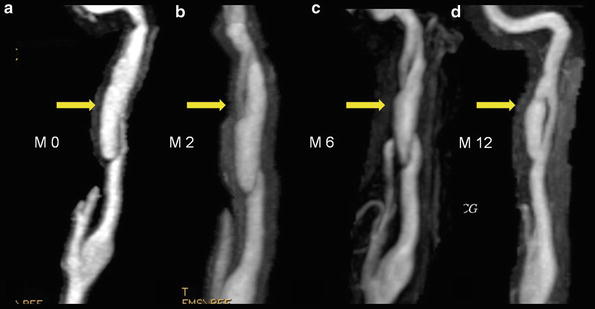
Fig. 5
Twelve-month evolution of a pseudoaneurysm type of carotid artery dissection with true and false lumen remaining patent
Mural Hematoma Imaging
Carotid artery dissection is characterized, on fat-suppressed T1 sequence, by a narrowed eccentric flow void, which is surrounded by a crescent-shaped, hyperintense area expanding the vessel diameter (Fig. 6) [53]. MR signal of the mural hematoma has a similar temporal evolution than intracerebral counterpart. [56] An acute mural hematoma can be hypointense on T2- and T1-WI images and therefore be difficult to distinguish from an area of flow void. The mural hematoma may therefore be missed on MRI within the first 48 h after CAD [53]. Thereafter, the hematoma becomes of intermediate signal intensity on T1-WI images and hyperintense on proton density and T2-WI images (Fig. 7). After a few days, the hematoma remains hyperintense on T1-WI images for approximately 2 months (Figs. 7 and 8). Subsequent loss of signal intensity can mask the hematoma after 6 months, as it becomes isointense with the surrounding soft tissue. These patterns are, however, not specific for a dissection as fresh thrombus due to an atheromatous or embolic occlusion that may exhibit a similar evolution of MR signal intensity. It may, indeed, be impossible to distinguish an intraluminal clot from an occlusive mural hematoma. In such cases, a crescentic thickening with eccentric signal void proximal to complete occlusion should suggest dissection rather than atheromatous occlusion (Fig. 9).
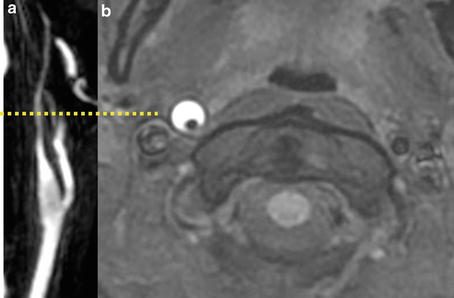
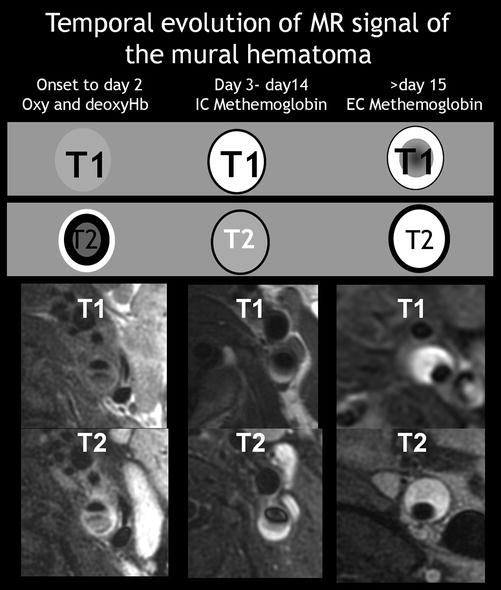
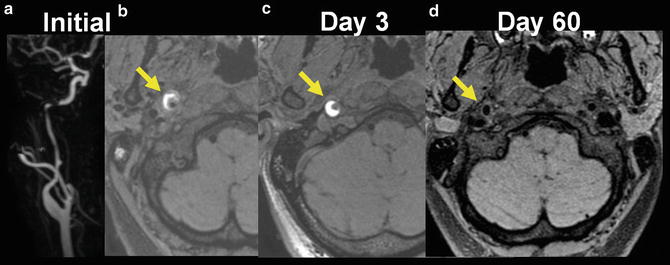
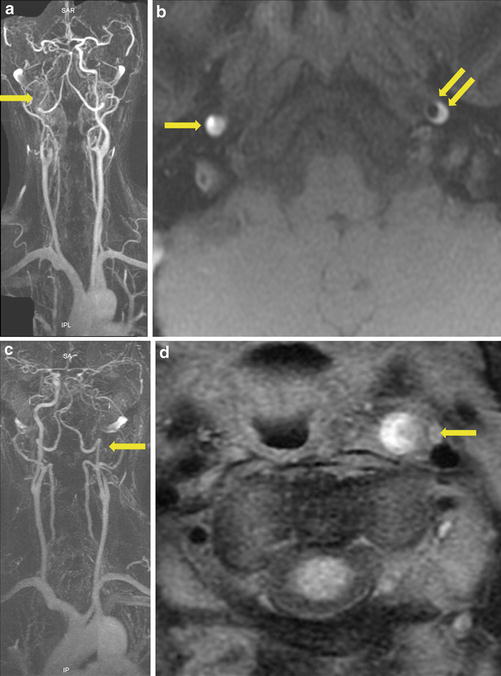
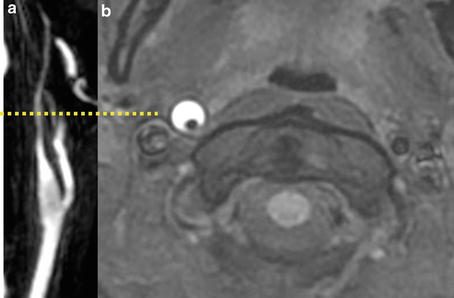
Fig. 6
Right carotid artery dissection on contrast-enhanced MR angiography (CE-MRA) (a) and axial fat-suppressed T1-WI image (b). Carotid artery dissection is characterized, on fat-suppressed T1-WI by a narrowed eccentric flow void, which is surrounded by a crescent-shaped, hyperintense mural hematoma expanding the outer vessel diameter
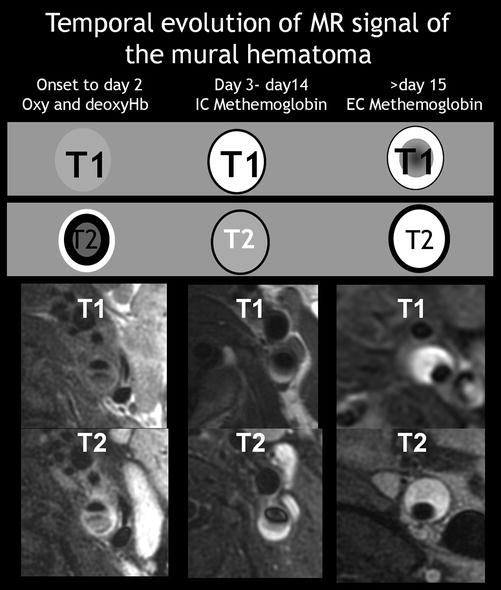
Fig. 7
Temporal evolution of MR signal of the mural hematoma on T1 and T2 sequences. An acute mural hematoma, e.g., within 2 days after onset, is isointense on T2- and T1-WI images. The hematoma becomes of intermediate signal intensity on T1-WI images and hyperintense on T2-WI images after day 2. The hematoma remains hyperintense on both T2- and T1-WI images for approximately 2 months. Subsequent loss of signal intensity can mask the hematoma after 6 months, as it becomes isointense with the surrounding soft tissue (not shown)
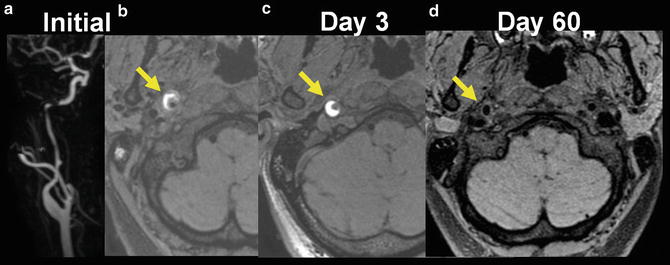
Fig. 8
Temporal evolution of MR signal of the mural hematoma on T1 sequence. Contrast-enhanced MR angiography (a) demonstrated a long irregular stenosis sparing the right ICA bulb. The mural hematoma was initially isointense to the surrounding tissue with bright heterogeneous signal of its peripheral part (b). It becomes homogeneously hyperintense at day 3 (c) and was no longer visible on fat-suppressed T1-WI image at day 60 (d)
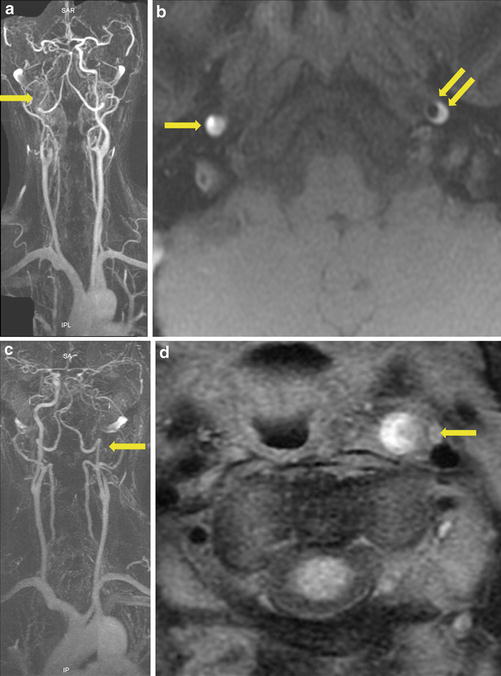
Fig. 9
Occlusive cases of carotid artery dissection. In the first patient (a, b), CE-MRA demonstrated right ICA occlusion and long stenosis of the left ICA. On fat-suppressed T1-WI image (b), it was difficult in the right ICA lumen to distinguish an intraluminal clot from an occlusive mural hematoma (arrow). CAD diagnosis was done, thanks to left ICA mural hematoma (double arrows). In the second patient (c, d), CE-MRA demonstrated left ICA occlusion (arrow). On fat-suppressed T1-WI image (d), it was also difficult distinguishing an intraluminal clot from an occlusive mural hematoma. In such a case, the crescentic hyperintense thickening associated with increase outer external diameter of the occluded ICA suggested dissection rather than atheromatous occlusion
At least four causes of false-positive MRI or MRA findings have been reported [57].
Atheromatous plaques causing subtotal occlusion can be hyperintense on T2-WI images and mimic a CAD [57]. This rim is, however, typically isointense on T1-WI images, whereas the periarterial rim in subacute CAD is usually hyperintense on T1-WI images [56].
Structures adjacent to arteries can simulate a periarterial rim of hyperintense signal which can mimic a mural hematoma or a pseudoaneurysm. For instance, carotid arteries are surrounded by fat. Systematic fat-saturation imaging is the best solution.
Turbulent or slow flow on MRI and MRA can be another confounding factor for CAD by producing hyperintense signal in the periphery of the arterial flow void, along the surface of the arterial lumen. For example, marked narrowing of the extracranial segment of the internal carotid artery can produce hyperintense slow flow distally, which can be manifested by loss of part or all of the intracranial flow void. This signal can be mistaken for mural hematoma. Inflow phenomena generally produce signal abnormalities that are located centrally within the flow void rather than peripherally. Homogeneity of the hyperintense signal on all slices associated with vessel expansion supports dissection rather than slow flow.
Flow-related enhancement in arteries and veins can simulate mural hematoma. Bright signal in arteries at the extreme of an imaging volume due to entry of unsaturated spins (i.e., the so-called entry slice phenomenon) has long been recognized as a cause of abnormal arterial signal on selected images. This phenomenon can simulate mural abnormal signal or complete absence of the expected flow void within an artery and be mistaken for dissection. The recognition that the image is obtained at the extreme of the imaging volume (i.e., first or second image in a stack of images) and correlation with the signal of the arteries on other imaging sequences resolve the issue.
A false-negative diagnosis of dissection on MR is most likely at the very early stage, when the mural hematoma is not yet hyperintense. Indeed, at this stage, the blood products within the hematoma have not yet progressed to the stage of methemoglobin. In this case, the signal intensity of the hematoma does not differ from that of background fat-suppressed tissue. Other signs, such as the presence of a pseudoaneurysm, or an increase in external diameter may help in these difficult cases.
In addition, whenever a CAD produces arterial occlusion (as opposed to stenosis), the mural hematoma may not be conspicuous against the background of the hyperintense signal abnormality of an occluded arterial lumen on fat-suppressed T1-WI images. In such cases, although the arterial occlusion is recognized, the cause may not be discernible.
The mural hematoma frequently extends cranially to the petrous carotid segment in the case of carotid artery dissection. This location is within the limits of the field of view of standard brain MRI. Consequently, apart from dedicated cervical fat-suppressed T1 sequences, brain MRI may be useful to demonstrate CAD. More than three-quarters of such acute CAD can be diagnosed using stroke brain MRI protocol only, including DWI, FLAIR, T2* sequences, and time-of-flight MRA (Fig. 10) [29]. This result might have clinical implications for patients who are not initially suspected of having CAD based on available history at the time of protocol and at institutions where cervical CE-MRA is not coupled with MR brain imaging. The interpretation of direct signs of CAD on brain MRI is a learnable skill and requires little specialized training and can also be achieved in regular clinical practice.
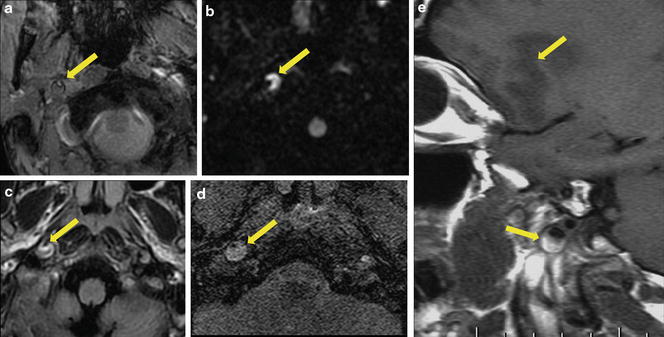
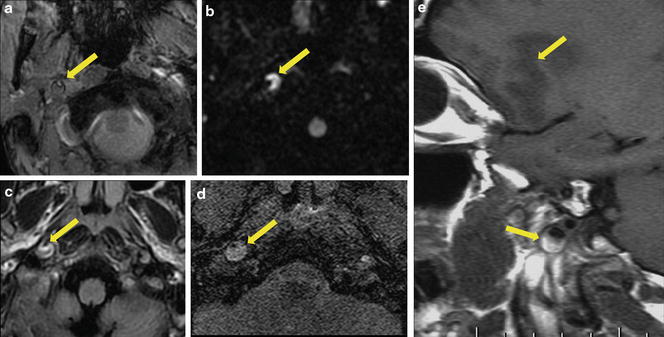
Fig. 10
Illustration of carotid artery dissection on brain MRI sequences in five patients. Axial gradient-recalled echo T2 (a), diffusion-weighted imaging (b), FLAIR (c), and native slices of 3D time-of-flight angiography of the circle of Willis (d). Sagittal T1-WI imaging (e). Increased external diameter, crescentic mural thickening (arrows), and eccentric lumen (arrows) were clearly seen in all patients
High-Resolution MRI
High-resolution magnetic resonance imaging (HR-MRI) is an attractive tool for the assessment of carotid artery diseases. Using dedicated surface radiofrequency coils at 1.5-T or standard coils at 3-T, it provides high-resolution images of the arterial wall in vivo. Optimal image quality relies on correct patient’s preparation, on adequate coil positioning, and on the use of pulse sequences with signal suppression of the flowing blood. HR-MRI has initially been applied for the in vivo analysis of carotid atherosclerosis with now solid evidence that HR-MRI can identify the major components of atherosclerotic plaque, i.e., lipid core, hemorrhage, calcifications, as well as the fibrous cap [58]. Beyond atherosclerosis, HR-MRI can be used in case of suspicion of cervical artery dissection, providing an excellent visualization of the cervical arterial mural hematoma on both carotid and vertebral arteries [59].
High-Resolution MRI Using Surface Coils at 1.5-T
The aim of MRI of the arterial wall of cervical arteries is to obtain in plane, submillimetric voxels. The loss of signal to noise induced by reducing the voxel size (500 × 500 μm × 2–3 mm) is compensated for by the use of phased-array surface coils, which increases the signal-to-noise ratio by 40 %. The so-called HR-MRI corresponds to images with submillimetric voxels that imply the use of dedicated surface coils. These coils are commercially available and compatible with most MR manufacturers. They consist of a pair of multichannel coils. The coils, made of flexible material, are placed bilaterally around the neck. The carotids, being superficial structures, are well suited for surface coil imaging.
To explore the patient with suspected CAD, the radiologist should ensure that the coil is at the level of the carotid-presumed lesion. A multicentric study reports a rate of 90 % of interpretable MRI exams performed for the purpose of carotid bifurcation analysis. For a given magnetic field when using the same surface coil, image quality seems stable across different platforms [60].
The MR protocol comprises multiple sequences in the axial plane, covering about 3 cm in height. Consequently, the slices must be focused on the index lesion or artery.
As in atherosclerotic plaque exploration, it is currently recommended to combine multiple contrasts to fully characterize potential mural hematoma. Pulse sequences designed for vascular imaging are classed as “bright blood” or “black blood,” depending upon the flowing blood signal. Both of these sequences provide a good contrast between the lumen and the arterial wall. Bright blood sequence corresponds to 3D TOF gradient echo sequences used for MR angiography. On this sequence, the flowing blood signal is enhanced, and the lumen is brighter than the vessel’s wall. The lack of 180° refocusing pulse creates T2*-sensitive signal. The aim of black blood (BB) sequences is to cancel the signal of the flowing blood so as to increase the contrast between the lumen (in black) and the wall. For that reason, the acquisition rate is synchronized to the heartbeat and is preceded by a double-inversion-recovery (IR) magnetization preparation pulse. This process maximizes flow suppression due to outflow and minimizes artifacts due to vessel motion. The time of inversion (TI) for the double-IR preparatory pulse is fixed as close to the null point of the blood signal. The double-IR preparation and rapid SE acquisition are typically repeated using 2×RR cardiac gating until all the rapid SE shots are acquired. Black blood sequence can be T2 or proton density (PD) weighted (2×RR) or T1-WI (1×RR). By applying the double-IR preparation just after the cardiac trigger, the slice-selective re-inversion pulse is placed in the “end-diastolic” part of the cardiac cycle. Because the TI is usually close to 650 ms, the rapid SE acquisition is also played out during diastole. A third inversion pulse can be added for fat suppression.
Several principles should be followed when designing an MR protocol for imaging cervical arterial wall in CAD: (1) the coil positioning is of importance and should be guided by lumen abnormalities seen on CE-MRA or ultrasonographic examination. The surface coils should be positioned anteriorly and laterally, facing the mandibular bone for the petrous portion of internal carotid artery, whereas it should be placed posterior and under the mastoid for the V3 segment; (2) acquire an oblique scout view (3D phase contrast, 2D time of flight, 1 min) of the entire index artery so as to visualize the plaque distribution and the level of the carotid lesion; (3) acquire HR images (voxel <500 × 500 μm in plane); (4) use multicontrasts, by combining black and bright blood sequences, to identify the mural hematoma presence and age; (5) keep the total acquisition time to less than 30 min. Indicative parameters at 1.5-T are detailed in Table 1 [58].
Table 1
Indicative imaging parameters for 1.5-T high-resolution plaque MR imaging using dedicated surface coils [60]
Parameters | 3D TOF | T1 BB | DP BB | T2 BB |
---|---|---|---|---|
TR (ms) | 30 | 1 RR | 2 RR | 2 RR |
TE (ms) | 6.9 | 9 | 16–20 | 50 |
FOV (mm) | 130 | 130 | 130 | 130 |
Slice thickness (mm) | 2.2 | 3 | 3 | 3 |
Matrix | 288 × 224 | 352 × 256 | 256 × 512 | 256 × 512 |
NEX | 2 | 3 | 2 | 2 |
Synchronization | – | Cardiac | Cardiac | Cardiac |
Number of slices | 20 | 8 | 8 | 8 |
Resolution (μm)a | 254 × 254 | 254 × 254 | 254 × 254 | 254 × 254 |
Scan time (min)b | 4 | 4–5b | 4–6b | 4–6b |
High-Resolution MRI at 3-T
Although most of the HR-MRI studies have been performed on 1.5-T MR units, 3-T units are now more widely available. Surface coils for carotid imaging are commercially available for 3-T MR units. These are slightly different from those used on 1.5-T units (tuned to a different frequency and adapted to the 3-T connectors and/or interfacing). They provide significant improvement in signal-to-noise and contrast-to-noise ratios [61], allowing reduction in both the acquisition time and the in-plane resolution. For a given acquisition time, thinner slices or larger anatomic coverage in the z-axis can be obtained. At 3-T, longer repetition times are advised for PD- and T2-WI images. Double-IR blood suppression techniques are available at 3-T, but they require an adjustment of the TI for the blood T1 at 3-T, i.e., slightly longer TI for 3-T compared to that used for 1.5-T [61]. Demonstration of strong agreement between 3 and 1.5-T HR-MRI of the carotid plaques, particularly in the identification of atherosclerotic plaque composition, including hemorrhage, supports the translation of histologically validated 1.5-T criteria to 3-T carotid images [62]. However, there are differences in the measured size of arterial wall hemorrhage measures, larger at 1.5-T than at 3-T, suggesting that patients enrolled in serial prospective trials of hematoma measurement in CAD should be imaged at the same field strength in order to minimize interstudy errors during analysis [62].
High-Resolution MRI Findings in CAD
Using standard MRI, an early and reliable identification of acute CAD might be impaired by the limited spatial resolution, the tortuous course of the arteries, the great variability in normal vessel caliber, and the presence of the thick bony covering and adjacent veins. This is the case for dissection of the upper portions of the vertebral arteries (V2 and V3 segments), outside the field of this review, and for dissection of petrous ICA. In some cases, the diagnosis of CAD remains presumptive, further confirmed by sequential follow-up MR showing signs of healing or progression in response to treatment.
1.5-T HR-MRI has demonstrated its usefulness for the assessment of CAD [59] and permits an excellent depiction of the structural characteristics of the vessel wall and the vessel lumen in acute CAD. The eccentric vessel lumen (occlusion, stenosis, luminal thrombus) and the vessel wall (crescent mural hematoma, pseudoaneurysm, double lumen, intimal tear) of the dissected artery can then be exquisitely analyzed using HR-MRI (Fig. 11) [63–65]. The mural hematoma is well defined between intimal and adventitial wall boundary. The signal intensity of mural hematoma varies with the time elapsed since the onset of symptoms [56]. More specifically, the age of the mural hematoma may be classified into acute, early subacute, late subacute, and chronic hematomas according to its signal intensities on the T1- and T2-WI sequences. One study suggested that the MR signal changes of mural hematoma had only moderate agreement with the dynamics seen in cerebral hematomas, even if it can be difficult to determine the exact delay between the occurrence of CAD and the HR-MRI scan. Initially, the mural hematoma is isointense to the sternocleidomastoid muscle on T1-WI and hypointense on T2 and TOF imaging. At a mean delay of 5,8 days, mural hematoma may appear hyperintense on all sequences (Figs. 7 and 8). Progressively, the signal drops on all pulse sequences [56].
