Fig. 1
(a–d) Hypothetical outcome of hyperfractionated (HF) as compared to conventional radiotherapy (CF), e.g., in head and neck squamous cell carcinoma. Actuarial curves displaying the primary study endpoint, e.g., overall survival and development of late toxicity. Hyperfractionated radiotherapy results in a therapeutic gain and increased rate of uncomplicated cure, i.e., survival without serious side effects
In the context of reirradiation, the issue of normal tissue toxicity is of utmost importance. While acute toxicity largely is comparable to that of first-line treatment, late toxicity resulting from high cumulative radiation doses has often been observed (Simmonds et al. 1989; Stewart 1999). Chronically progressive fibrosis, stenosis, and perfusion deficits resulting in tissue necrosis have been described. Their impact on quality of life and organ function might be severe. With regard to palliative reirradiation, late toxicity might not become clinically apparent during the limited life span of most patients. However, the situation might be different in patients treated with curative intent (Nieder et al. 2000). It is therefore necessary to assess the biologically effective dose (BED) and late effects of the initial course of radiotherapy and to exclude patients who tolerated their previous treatment poorly. In addition, efforts must be made to limit the volume of reirradiated normal tissues. Today’s improved target volume imaging and definition approaches as well as image-guided high precision techniques contribute to volume reduction and improved dose distribution. Proton radiotherapy may in the future add to current options for high conformity reirradiation techniques. Harnessing high precision technology might even allow for hypofractionated short-course reirradiation as demonstrated, e.g., in palliative treatment of head and neck cancer (Heron et al. 2011), recurrent high-grade gliomas (Grosu et al. 2005; Nieder et al. 2008; Fogh et al. 2010), stereotactic irradiation of lung (Peulen et al. 2011; Meijneke et al. 2013; Kilburn et al. 2014) and stereotactic radiosurgery of vestibular schwannomas (Yomo et al. 2009), spinal metastases (Choi et al. 2010), or brain metastases (Maranzano et al. 2012). Single doses were also given with intraoperative approaches, e.g., in rectal cancer (Haddock et al. 2001). In addition, several brachytherapy regimens have been used, e.g., in prostate and head and neck cancer (Burri et al. 2010). Intraoperative radiotherapy and single fraction radiosurgery are not the focus of this chapter. The disease-specific chapters provide details on such approaches. In a Canadian survey on reirradiation, many respondents recommended brachytherapy or highly conformal external irradiation techniques (Joseph et al. 2008). Hyperfractionated schedules were suggested as a means of limiting retreatment toxicity. Obviously, hyperfractionation was among the few strategies available in the past and also utilized by one of the authors of this chapter in the 1990s (Nieder et al. 1999) before positron emission tomography (PET), intensity-modulated radiotherapy (IMRT), proton beams, and other tools became a part of our armamentarium.
Reirradiation is not a new idea, some clinical studies were published already more than 40 years ago and some of these included patients treated as early as 1940 (Shehata et al. 1974; Fu et al. 1975; Hunter and Stewart 1977; Laramore et al. 1978; Dritschilo et al. 1981). The same holds true for accompanying experimental studies (Brown and Probert 1975). The vast majority of published reirradiation series consist of retrospective data or small prospective studies. In addition, the typical patient populations are more heterogeneous than in first-line radiotherapy studies. For example, patients with local relapse, regional relapse, or second primary tumors might be included. Therefore, the level of evidence is not comparable to that of first-line radiotherapy, where many treatment recommendations and guidelines are based on large and well-designed prospective randomized trials or meta-analyses of several trials. However, it appears that reirradiation not only palliates cancer-related symptoms but, under certain circumstances, might contribute to improved survival, especially in diseases where local control determines survival (Jereczek-Fossa et al. 2008). One example is a study of 108 children with relapsed ependymoma where 66 % received radiotherapy at relapse and 50 % of older children were reirradiated, and where reirradiation was associated with better outcome (Messahel et al. 2009). In a smaller study, which included 25 patients with previously irradiated recurrent medulloblastoma, a trend towards better event-free survival was seen in patients who received additional radiotherapy as part of their retrieval therapy (Dunkel et al. 2010). Limited evidence is also available from reirradiation of benign tumors such as pituitary adenoma (Schoenthaler et al. 1992). In a randomized trial, 130 patients with head and neck cancer were treated with salvage surgery and randomly assigned to full-dose reirradiation combined with chemotherapy or to observation. Full-dose reirradiation combined with chemotherapy after salvage surgery significantly improved disease-free survival, but had no significant impact on overall survival (Janot et al. 2008).
2 Hyperfractionation in First-Line Radiotherapy
The clinical evaluation of hyperfractionated radiotherapy in patients with advanced squamous cell carcinoma of the head and neck started in the 1970s (Meoz et al. 1984). EORTC protocol 22791 compared once-daily fractionation of 70 Gy in 35–40 fractions in 7–8 weeks, to pure hyperfractionation of 80.5 Gy in 70 fractions in 7 weeks using 2 fractions of 1.15 Gy per day (i.e., same conventional overall treatment time), in T2-T3 oropharyngeal carcinoma (excluding base of tongue), node negative, or N1 of less than 3 cm (Horiot et al. 1992). From 1980 to 1987, 356 patients were randomized. As published in 1992, the local control was significantly higher (p = 0.02) after hyperfractionation. The multivariate Cox model confirmed that the treatment regimen was an independent significant prognostic factor for locoregional control (p = 0.007). This improvement of locoregional control was responsible for a trend to an improved survival (p = 0.08). There was no difference in late normal tissue damage between the two treatment modalities, although some controversy exists about the certainty to which differences in normal tissue damage may be excluded by this and other trials (Baumann et al. 1998; Baumann and Beck-Bornholdt 1999; Bentzen et al. 1999). Several trials of unconventional fractionated radiotherapy in head and neck squamous cell carcinoma followed, e.g., by the RTOG (Fu et al. 2000), but the effect of such treatment on survival remained unclear. A meta-analysis of updated individual patient data was performed (Bourhis et al. 2006). Trials were grouped in three prespecified categories: hyperfractionated, accelerated, and accelerated with total dose reduction. Tumor sites were mostly oropharynx and larynx; 74 % of patients had stage III-IV disease. There was a significant survival benefit with altered fractionated radiotherapy, corresponding to an absolute benefit of 3.4 % at 5 years (hazard ratio 0.92, 95 % CI 0.86–0.97; p = 0.003). The benefit was significantly higher with hyperfractionated radiotherapy (8 % at 5 years) than with accelerated radiotherapy (2 % with accelerated fractionation without total dose reduction and 1.7 % with total dose reduction at 5 years, p = 0.02). There was a benefit on locoregional control in favor of altered fractionation versus conventional radiotherapy (6.4 % at 5 years; p < 0.0001), which was particularly efficient in reducing local failure, whereas the benefit on nodal control was less pronounced.
Certain side effects such as development of radiation retinopathy might also be less likely in patients with head and neck cancer treated with hyperfractionated radiotherapy. In a study by Monroe et al. (Monroe et al. 2005), 186 patients received a significant dose to the retina as part of curative radiotherapy. Primary sites included nasopharynx, paranasal sinus, nasal cavity, and palate. Hyperfractionated radiation was delivered to 42 % of the patients in the study, typically at 1.1–1.2 Gy per fraction. The remainder were treated once daily. Thirty-one eyes in 30 patients developed radiation retinopathy, resulting in monocular blindness in 25, bilateral blindness in one, and decreased visual acuity in four. The actuarial incidence of developing radiation retinopathy was 20 % at both 5 and 10 years. Higher retinal doses resulted in a steady increase in the incidence of retinopathy, with 25 of the 30 cases occurring after 60 Gy or more. Of the patients receiving more than 50 Gy to the retina, hyperfractionation was associated with a significantly lower incidence of radiation retinopathy (37 % vs. 13 %; p = 0.0037). On multivariate analysis, retinal dose (p < 0.0001) and fractionation schedule (p = 0.0003) were significant predictors of radiation retinopathy.
Patients with localized unresectable non-small cell carcinoma of the lung were also treated with hyperfractionated regimens, e.g. 1.2 Gy twice daily (Seydel et al. 1985). Of 120 eligible patients, 10 received a dose of 50.4 Gy, 20 received 60.0 Gy, 79 received 69.6 Gy, and 11 patients received 74.4 Gy. Complete regression occurred in 19 % of T1-T3, N0-N2 patients. There were six cases of severe and two of life-threatening toxicity, but there were no fatalities attributable to the treatment. Toxicity consisted mainly of pneumonitis and pulmonary fibrosis as well as esophagitis. Median survival of the entire group was 7.2 months, which was consistent with previous experience at that time. It was later found that all five of the 5-year survivors came from the 79 patients assigned to receive 69.6 Gy (Cox et al. 1991). Combined stage II and III 5-year survival rates were 8 % for 69.6 Gy compared to 6 % for standard once-a-day irradiation in concurrent RTOG trials. A randomized phase III trial shed more light on the issue of hyperfractionation (Sause et al. 1995). Three arms were evaluated (1) standard radiation therapy, (2) induction chemotherapy followed by standard radiation therapy, and (3) twice-daily radiation therapy. Patients were required to have a Karnofsky performance status of 70 or more and weight loss less than 5 % for 3 months prior to entry into the trial. Of the 490 patients registered in the trial, 452 were eligible. The disease in 95 % of the patients was stage IIIA or IIIB. Patients were randomly assigned to receive either 60 Gy of radiation therapy delivered at 2 Gy per fraction, 5 days a week, over a 6-week period (standard radiation therapy), induction chemotherapy consisting of cisplatin and vinblastine followed by standard radiation therapy starting on day 50, or 69.6 Gy delivered at 1.2 Gy per fraction twice daily. One-year survival (%) and median survival (months) were as follows: standard radiation therapy – 46 %, 11.4 months; chemotherapy plus radiotherapy – 60 %, 13.8 months; and hyperfractionated radiation therapy – 51 %, 12.3 months. The chemotherapy plus radiotherapy arm was statistically superior to the other two treatment arms (p = 0.03). This was confirmed in a later analysis of the trial (Sause et al. 2000). Other groups explored hyperfractionation in non-randomized studies of early stage non-small cell lung cancer (Jeremić and Milicić 2008, Jeremic et al. 1997). However, other developments (accelerated radiotherapy (Mauguen et al. 2012), concomitant chemoradiation (Aupérin et al. 2010, stereotactic body radiotherapy (Zhang et al. 2014)) outperformed classical hyperfractionation, which has not evolved into a standard of care in non-small cell lung cancer.
Hyperfractionated radiotherapy was studied in a large variety of other cancer types, but no general benefit was found. One of the unsuccessful examples is high-grade gliomas (Nieder et al. 2004). None of the glioma studies reviewed in 2004 reported a significant improvement in survival by altered fractionation in comparison to either institutional historical controls or their respective randomized control arm.
3 Hyperfractionated Reirradiation
In contrast to first-line radiotherapy where systematic efforts were undertaken to compare different fractionation regimens, no such randomized comparisons are available. The retrospective series reported by Bauman et al. (Bauman et al. 1996) included 17 patients with primary CNS tumors who received hyperfractionated reirradiation and 17 patients treated with once-daily fractionation (Table 1). Some children, e.g., with medulloblastoma were included in this heterogeneous population. Median overall survival was 8.3 months in all 34 patients. The actuarial risk of necrosis was 22 % at 1 year following retreatment. Fractionation had no statistically significant influence on overall survival, progression-free survival, or increased complications in this study with limited statistical power. A retrospective comparison in patients with rectal cancer was reported in 1997 (Lingareddy et al. 1997). The study included 52 patients, 22 of whom opted for hyperfractionated radiotherapy, while the others preferred conventional once-daily treatment, mainly for logistical reasons. Ninety percent received concomitant 5-FU. Fractionation had no statistically significant influence on overall survival, but late toxicity was significantly reduced in patients who had received hyperfractionated treatment (relative risk 3.9, 95 % confidence limit 1.1–14.4). No such correlation was found in the retrospective study of reirradiation for rectal cancer patients who underwent resection after reirradiation with concurrent 5-FU (Mohiuddin et al. 1997). Another study in rectal cancer where all patients received hyperfractionated reirradiation reported relatively moderate rates of late toxicity (necessitating surgery in only one patient, Table 1), but 15 % serious postoperative complications in those patients who eventually underwent tumor resection after chemoradiation (Valentini et al. 2006). The other studies summarized in Table 1 were not designed to evaluate the impact of hyperfractionation on outcome. In patients reirradiated for head and neck cancer and analyzed for development of carotid blowout syndrome (CBOS), the rate of CBOS was 1.3 % in those treated in a continuous course with 1.8–2 Gy daily fractions or 1.2 Gy twice-daily fractions (McDonald et al. 2012). However, the rate was 4.5 % in patients treated with 1.5 Gy twice daily in alternating weeks or with delayed accelerated hyperfractionation (p = 0.002). Another retrospective study on reirradiation of head and neck cancer patients treated with hyperfractionated radiotherapy with 1.2 Gy up to 66 Gy showed that 56 % of the surviving patients will develop grade 3 or higher toxicity (Lohaus et al. 2013). Thus, there is very limited evidence supporting the hypothesis that hyperfractionated reirradiation, which is challenging with regard to logistics and resource utilization, would improve the therapeutic index by reducing late toxicity. On the other hand, the regimens evaluated so far were often administered with treatment planning approaches and technology that are no longer in use, resulting in unnecessary large volumes of irradiated normal tissues. This leads us to conclude that a randomized trial of conventional versus hyperfractionated reirradiation utilizing, e.g., image-guided IMRT or IMPT would be of interest.
Table 1
Hyperfractionated reirradiation: study overview
Reference | Study type | Disease site | Number of patients | Interval between series | Reirradiation | Side effects | Median survival |
---|---|---|---|---|---|---|---|
Lingareddy et al. (1997) | Retrospective | Locally recurrent rectal cancer | 22 out of 52 in the study | Minimum 3 months, median 24 monthsa | 30 Gy with or without additional boost, varying total doses, 1.2 Gy b.i.d., typically with concurrent 5-FU | Acute grade III toxicity in 31 %a. Late grade III-IV toxicity in 33%a | 12 monthsa |
Mohiuddin et al. (1997) | Retrospective | Locally recurrent rectal cancer | 21 out of 39 in the study | Minimum 3 months, median 18 monthsa | 30 Gy with or without additional boost, varying total doses, 1.2 Gy b.i.d., typically with concurrent 5-FU, resection 8–12 weeks later | Treatment break or termination in 18 %a. Delayed wound healing in 7 %a. Severe late toxicity in 28 %a. | 45 monthsa |
Valentini et al. (2006) | Multicenter phase II | Locally recurrent rectal cancer | 59 | Minimum 9 months, median 27 months | 30 Gy + 10.8 Gy boost, 1.2 Gy b.i.d., concurrent 5-FU, resection 6–8 weeks later, then chemotherapy | 12 % of patients developed late toxicity | 42 months |
Karam et al. (2015) | Retrospective | Recurrent nasopharyngeal carcinoma | 32 out of 42 in the study | Minimum not reported, median 4.6 years | Total dose 40–60 Gy, 1.1–1.4 Gy b.i.d., typically with chemotherapy, IMRT in 27 patients | Late grade III-IV toxicity rate 37 % at 3 yearsa | 3-year survival 49%a |
Popovtzer et al. (2009) | Retrospective | Recurrent squamous cell head and neck cancer | 31 out of 66 in the study | Minimum 6 months, median 37 monthsa | Total dose 70 Gy at 1.25 Gy b.i.d. with concurrent cisplatin and 5-FU | Acute grade III-V in 10 %. Late grade III-V in 29 % | Approximately 19 months (estimated from the published graph)a |
Benchalal et al. (1995) | Pilot study | Head and neck cancer | 19 | Minimum 9 months, median 30 months | Total dose 60 Gy at 1.2 Gy b.i.d. | Acute grade III toxicity in 47 %. Late grade III toxicity in 11 % | Approximately 18 months (estimated from the published graph) |
Bauman et al. (1996) | Retrospective | Primary central nervous system tumors | 17 | Minimum 3.7 months, median 17.5 months | Total dose 30–44 Gy, in some cases over 60 Gy at 1.0 Gy b.i.d. | 1 case of necrosis, 1 of cognitive decline, 1 of increased tumor cyst | Not reported |
Lohaus et al. (2013) | Retrospective | Recurrent squamous cell head and neck cancer | 34 out of 40 in the study | Minimum 6 months, median 12 months | Total dose 60–66 Gy at 1.2 Gy b.i.d. with concurrent cisplatin and 5-FU | Late grade III-IV toxicity in 56 % | 18 months |
Table 2 summarizes the results of hyperfractionated-accelerated reirradiation. In these studies, the typical dose per fraction was 1.5 Gy. If one intends to administer a total dose of 45 Gy in a hyperfractionated accelerated fashion, i.e., 1.5 Gy b.i.d., 15 treatment days are needed. This compares to 25 treatment days when once-daily treatment with 1.8 Gy per fraction is chosen. As with hyperfractionated reirradition, no randomized trials have been published. Given the design and size of the available studies, no definitive conclusions can be drawn. However, comparison with studies where conventional fractionation was employed in the same cancer types does not provide strong indications for improved efficacy or therapeutic ratio. This should be considered in clinical decision making, as illustrated in the example provided in Fig. 2. Figure 3 elaborates on the usefulness of isoeffect calculations in reirradiation scenarios. The BED obtained with different fractionation regimens is calculated in Table 3A and 3B.
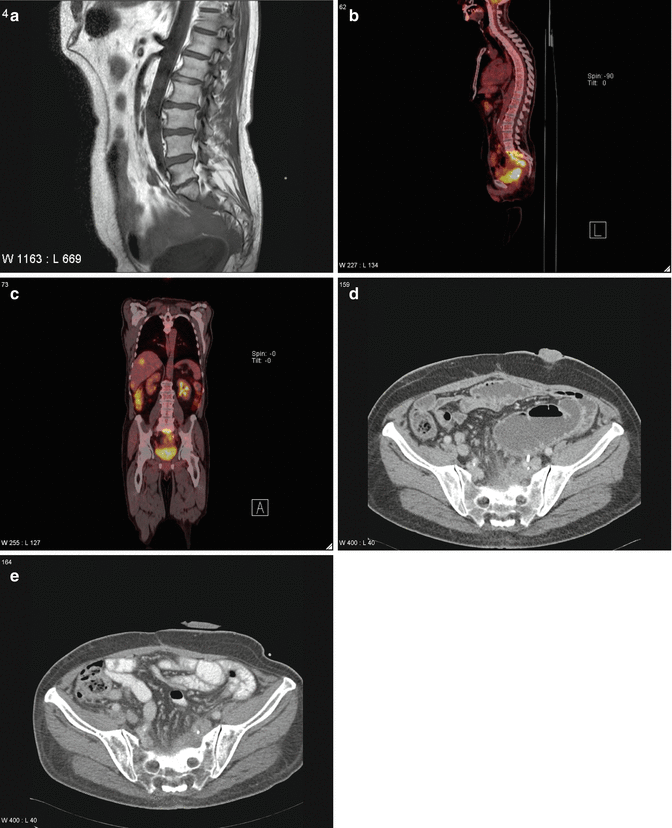
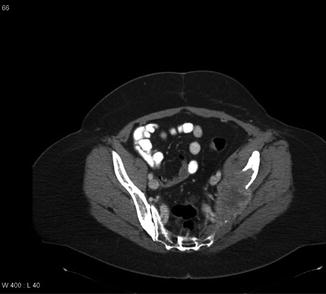
Table 2
Hyperfractionated-accelerated reirradiation: study overview (not all head and neck cancer studies are shown)
Reference | Study type | Disease site | Number of patients | Interval between series | Reirradiation | Side effects | Median survival |
---|---|---|---|---|---|---|---|
Abdel-Wahab et al. (1997) | Pilot study | Relapsed brain metastases | 15 | Minimum 8 weeks, median 10 months | Limited brain fields after previous WBRT; 1.5 Gy b.i.d., median 30 Gy | No serious toxicity | 3.2 months |
Nieder et al. (1999) | Retrospective | Relapsed high-grade gliomas | 32 | Minimum 8 weeks, median 20 months | Limited brain fields; 1.3 Gy b.i.d., total dose 45.5 Gy (during the later part of the study 1.5 Gy b.i.d., total dose 45 Gy) | 16 % late toxicity including 2 patients with radionecrosis | 8.5 months |
Spencer et al. (2008) | Multi-institutional prospective trial RTOG 9610 | Unresectable recurrent squamous cell carcinoma of the head and neck | 79 | Minimum 0.6 years, median 2.5 years | 4 weekly cycles of chemoradiotherapy separated by 1 week of rest; 1.5 Gy b.i.d., total dose 60 Gy | 6 deaths in acute period, acute grade IV in 18 %, late grade III and IV in 22 %, feeding tube at last follow-up in 70 % | 8.5 months |
Watkins et al. (2009) | Retrospective | Locoregionally recurrent head and neck tumors | 39 | Minimum 0.5 years, median 2.3 years | 4 weekly cycles of chemoradiotherapy separated by 1 week of rest; 1.5 Gy b.i.d., total dose 60 Gy | 4 deaths in acute period, acute grade IV in 10 %, late grade III-V in 56 % | 19 months |
Haque et al. (2009) | Retrospective | Gastrointestinal tumors (pancreas, bile duct, colon etc.) | 13 | Minimum 5 months, median 26 months | Typically 30–39 Gy total dose, 1.5 Gy b.i.d. | Late grade III toxicity: 0, late grade IV toxicity: 1 (8 %) | 14 months |
Das et al. (2010) | Retrospective | Rectal cancer | 50 | Minimum 0.4 years, median 2.3 years | If interval <1 year: 30 Gy If interval ≥1 year: 39 Gy 1.5 Gy b.i.d. Typically with concurrent capecitabine | Late grade III or IV toxicity: 13 (26 %) | 26 months |
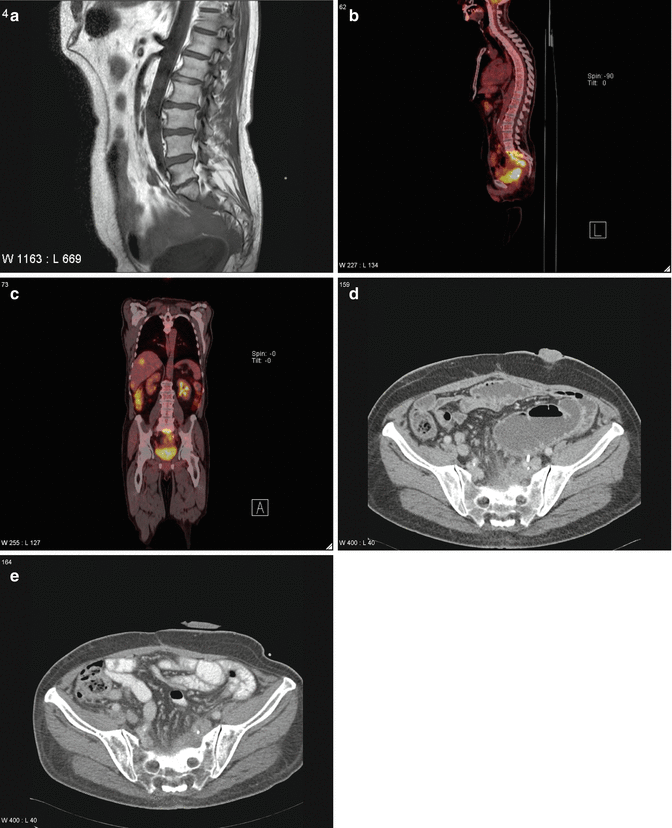
Fig. 2
An illustrative case from one of the authors’ institutions (Nordland Hospital Bodø, Norway). A 56-year-old male patient was diagnosed with anal cancer stage T3 N0 M0 in 1997. He received radiotherapy (40 Gy plus 10 Gy boost in 2-Gy fractions) with concomitant 5-FU and mitomycin-C. In 2001, the patient developed local relapse and was salvaged surgically. In 2007, both local relapse in the presacral region and liver metastases were detected. Palliative chemotherapy was initiated, first cisplatin and 5-FU, then 5-FU and mitomycin-C. Because of increasing pelvic pain, the patient was referred for palliative reirradiation in December 2008. The liver metastases were stable. No obvious late toxicity from the initial course of radiotherapy was present. The patients Karnofsky performance status (KPS) at that time was 70. We considered the following key questions. Is the patient’s performance status at a level that justifies initiation of radiation therapy? Yes, the KPS was 70. Do laboratory tests point to advanced hepatic disease and/or poor tolerability/efficacy of the planned therapy? No, the only abnormal finding was slight anemia and elevated alkaline phosphatase. Are other disease sites absent or controlled and if so, does one expect continued disease control? The liver metastases were stable. Will systemic treatment be offered or are there no more options left? Taxane-based chemotherapy was an option. Will local control impact on the survival of the patient or is treatment focused on palliation of symptoms? The aim was palliation of pelvic pain. Might the cumulative radiation dose to critical normal tissue structures result in serious toxicity in patients with expect prolonged survival? The probability of bowel or bladder toxicity, insufficiency fracture, or nerve damage was considered low and the same holds true for the probability of long-term survival. How did the tumor respond to initial radiotherapy and how long is the interval? Complete remission was obtained in 1997 and the interval of 11 years did permit reirradiation. The magnetic resonance, positron emission tomography (PET), and computed tomography (CT) images above show the presacral tumor mass, its bony extension, two liver metastases, and a ureteral stent. Tables 1 and 2 provide an overview on rectal cancer reirradiation studies, which might guide decision making. When deciding between hyperfractionated, conventional, and hypofractionated three-dimensional conformal radiotherapy in this case, the following facts were considered. The planning target volume resulting from the PET-CT-defined gross tumor volume did not include large volumes of bowel or bladder. The interval from initial treatment was very long. No concomitant chemotherapy would be added because the patient had previous heavy exposure to the standard drugs. Because of a lack of clinical data in the reirradiation setting, taxanes or oxaliplatin were not considered. Thus, we decided that hypofractionated reirradiation appeared feasible in this palliative setting. The patient received 12 fractions of 3 Gy in January 2009. He developed urinary infection and received antibiotics and a new stent. No other acute complications or toxicity was registered. Pelvic pain improved, though not completely. No additional systemic treatment was given. At the last follow-up in February 2010, the presacral tumor was stable (CT image panel e), and the patient was without obvious late toxicity
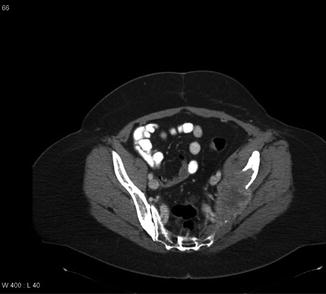
Fig. 3
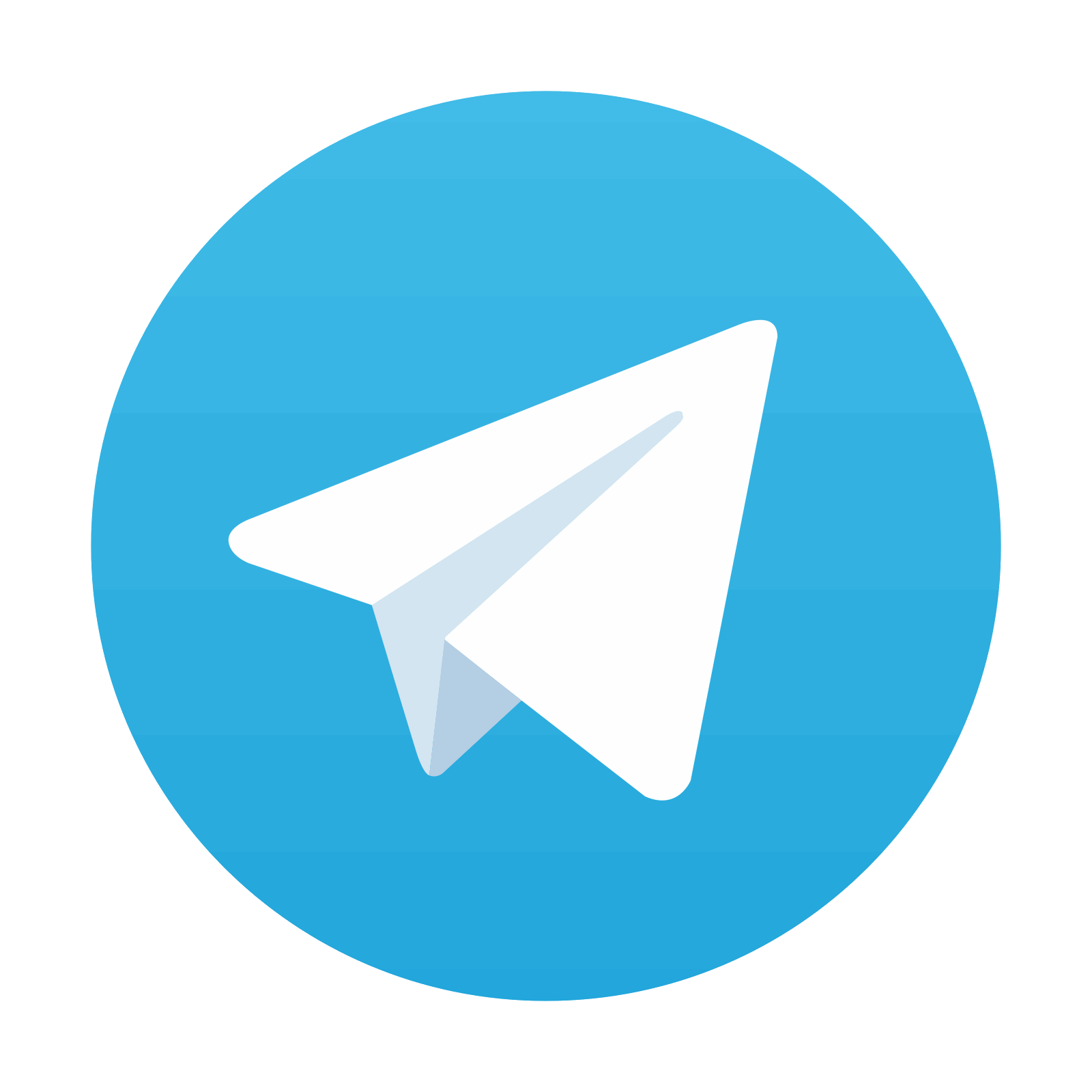
An illustrative case from one of the authors’ institutions (Nordland Hospital Bodø, Norway). A 49-year-old female patient was diagnosed with metastasized high-grade leiomyosarcoma of the uterus with multiple lung metastases and two bone metastases in 2005. After hysterectomy, she received three cycles of adriamycin without objective response. Palliative radiotherapy to a large painful pelvic bone metastasis (computed tomography (CT) image below) was given in May 2006, and the total dose was 39 Gy in 13 fractions of 3 Gy. Two anterior-posterior opposing fields were used. The lesion diminished in size (<50 % reduction) and then remained stable until February 2008 when the patient presented with increasing pain and bone destruction and was referred for reirradiation. She had not received second-line chemotherapy. With regard to potential side effects and fractionation of reirradiation, the following considerations are important. On a planning CT scan with empty bladder, the amount of reirradiated bladder was judged to be minimal. Bony structures had to be reirradiated to full dose as they were part of the clinical target volume. The same holds true for certain muscles and soft tissue. The skin could be spared to a large degree with three-dimensional treatment planning. The major organs at risk were small and large bowel and the sacral and presacral nerve roots and nerves. When calculating the biologically effective dose (BED) of the first irradiation course, one has to keep in mind that two opposing fields were used resulting in a maximum dose to parts of the bowel of 105 % of the prescribed dose, which was 3 Gy. Thus, these parts had received 13 fractions of 3.15 Gy. We will use an alpha/beta value of 3 Gy in this example. The resulting BED is 13 × 3.15 × (1 + 3.15÷3), i.e., 84 Gy3, according to the formula n × d × (1+ d÷alpha/beta value) where n is the number of fractions and d the dose per fraction. This dose is actually equivalent to 50 Gy in 25 fractions of 2 Gy (BED 83 Gy3). If one wishes to limit the reirradiation BED to the equivalent of 40 Gy in 20 fractions of 2 Gy (BED 67 Gy3), the following alternatives result: 40 Gy in 20 fractions of 2 Gy, 35 Gy in 14 fractions of 2.5 Gy (BED 64 Gy3), 30 Gy in 10 fractions of 3 Gy (BED 60 Gy3), 28 Gy in 7 fractions of 4 Gy (BED 65 Gy3) and, of course, lower doses such as 20 Gy in 5 fractions of 4 Gy. For an alpha/beta value of 4 Gy, the same reirradiation schedules would be feasible. The previous regimen of 39 Gy in 13 fractions had resulted in a quite long palliative benefit and no obvious late toxicity. The choice will now depend on the patient’s life expectation and willingness to accept the possible consequences of bowel, soft tissue, and nerve toxicity. Other factors such as travel distance might also impact on the patient’s preference
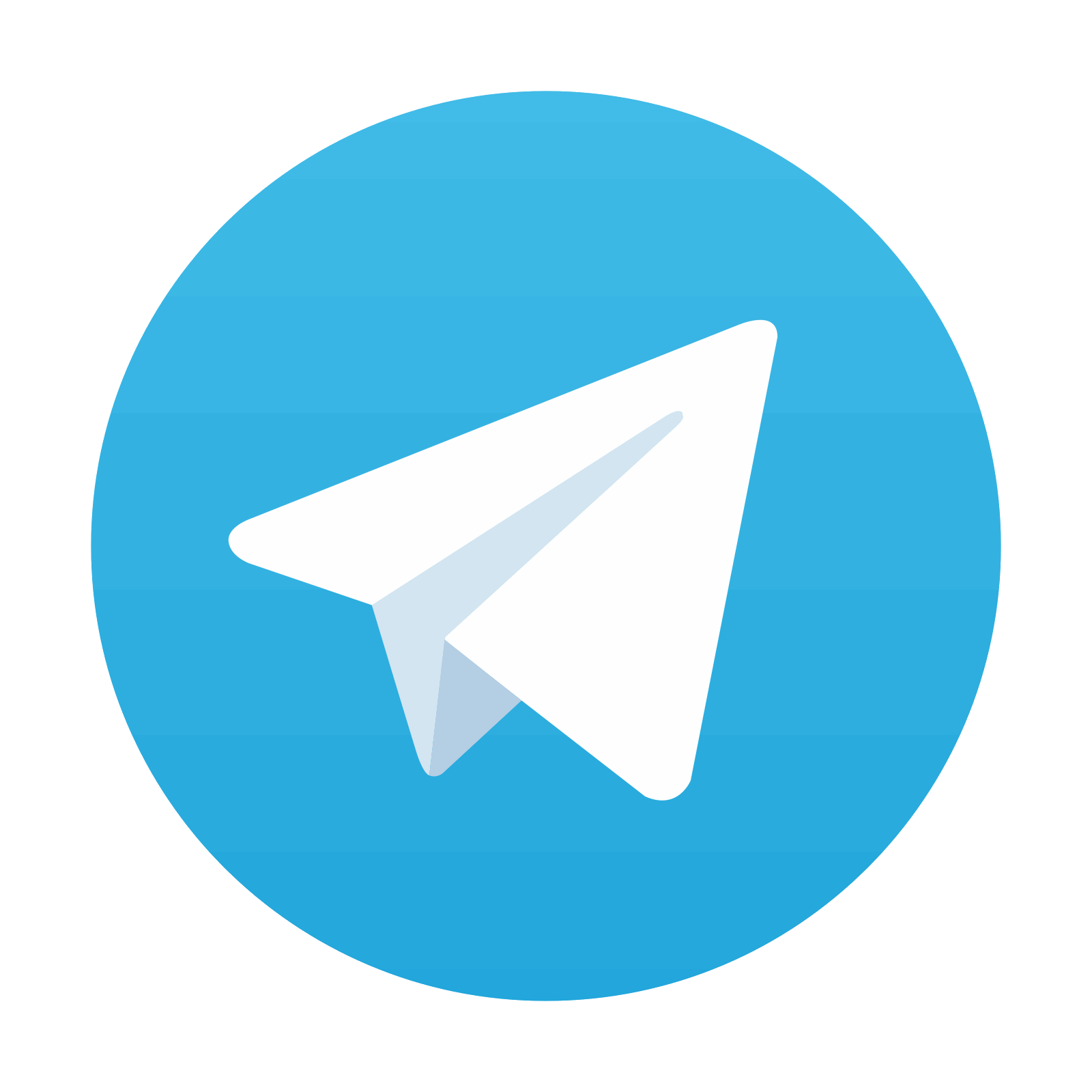
Stay updated, free articles. Join our Telegram channel

Full access? Get Clinical Tree
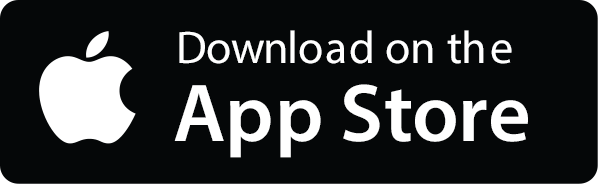
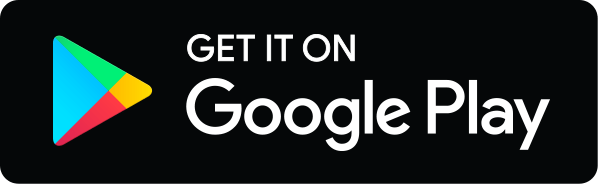
