Fig. 1
Embryology of the vertebral arteries. The vertebral arteries develop from longitudinal plexiform anastomoses of the first six cervical intersegmental arteries. The seventh cervical intersegmental arteries develop into the subclavian arteries
Prior to the development of the mature vertebrobasilar system, temporary anastomoses between the cranial portions of the dorsal longitudinal neural arteries and the primitive internal carotid arteries exist [1]. These carotid-vertebrobasilar anastomoses, as well as the cervical intersegmental arteries, eventually regress (with the exception of the seventh cervical intersegmental arteries which develop into the subclavian arteries). If these anastomoses fail to regress, communication between the vertebrobasilar system and the internal carotid artery persists into adulthood. Four major carotid-vertebrobasilar variants have been described in the literature and are named according to the cranial nerves that they parallel (Fig. 2): the persistent trigeminal artery, the persistent otic artery, the persistent hypoglossal artery, and the proatlantal intersegmental artery [1–3]. In general, these variants are considered incidental findings; however, an association with an increased incidence of aneurysms has been described [3].
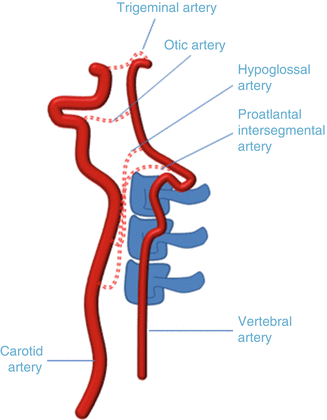
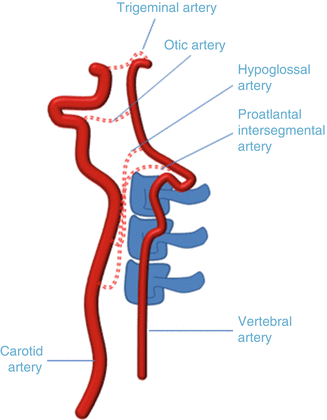
Fig. 2
Carotid-vertebrobasilar variants. The persistent trigeminal artery is the most common variant, connecting the cavernous internal carotid artery with the distal basilar artery. The persistent otic artery connects the petrous internal carotid artery with the proximal basilar artery via the internal acoustic meatus. The persistent hypoglossal artery connects the distal cervical internal carotid artery with the proximal basilar artery via the hypoglossal canal. The proatlantal intersegmental artery connects the distal cervical internal carotid artery with the proximal basilar artery via the foramen magnum
The persistent trigeminal artery is the most common carotid-vertebrobasilar variant, present in 0.1–1.0 % of cerebral angiograms and autopsies [1, 3]. It arises from the cavernous internal carotid artery and runs across the dorsum sellae to connect with the distal basilar artery (Fig. 3); the basilar artery proximal to the aberrant connection is often hypoplastic. On sagittal imaging, the persistent trigeminal artery forms a characteristic “tau” or “trident” sign (Fig. 4) [5]. The persistent trigeminal artery can occasionally be mistaken for an aneurysm on cross-sectional imaging; 3D CTA or DSA can be helpful in these difficult cases (Figs. 5 and 6). Patients are typically asymptomatic but can uncommonly present with trigeminal neuralgia if there is significant mass effect on the trigeminal nerve.
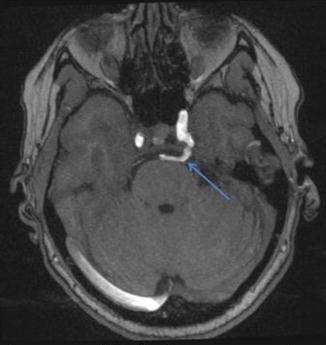
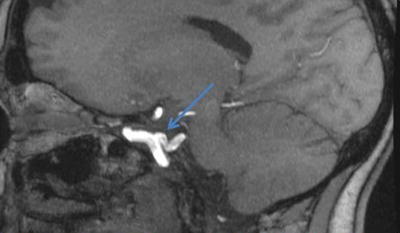
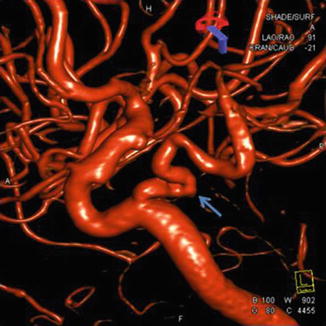
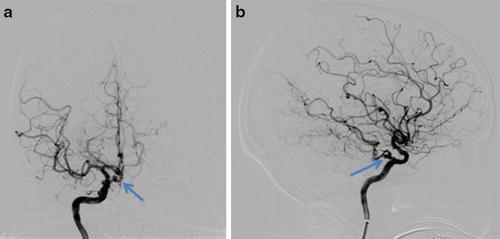
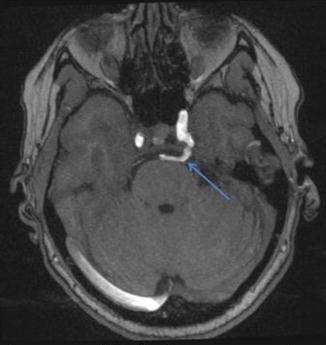
Fig. 3
MRA in a 45-year-old female demonstrating a persistent trigeminal artery (arrow) which connects the cavernous internal carotid artery with the distal basilar artery
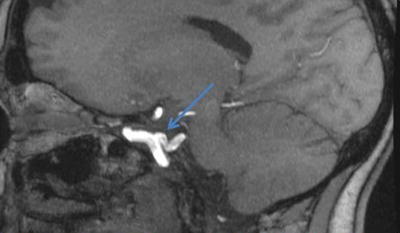
Fig. 4
“Tau” sign (arrow) on MRA sagittal reformat in a patient with a persistent trigeminal artery. The persistent trigeminal artery is the most common carotid-vertebrobasilar variant
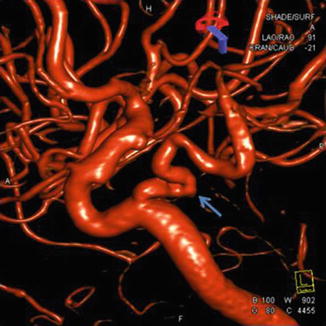
Fig. 5
3D carotid angiography nicely demonstrates a persistent trigeminal artery (arrow) which was mistaken for an aneurysm on prior MRA
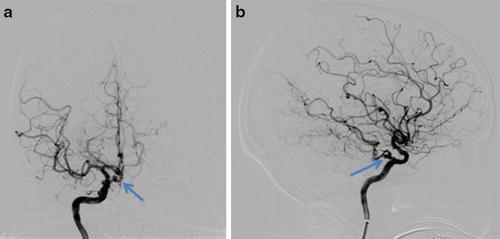
Fig. 6
Right carotid DSA in the same patient as in Fig. 5 with frontal (a) and lateral (b) projections, again demonstrating a persistent trigeminal artery (arrow)
The persistent hypoglossal artery is the second most common carotid-vertebrobasilar variant, present in 0.1–0.25 % of cerebral angiograms and autopsies [1, 3]. It arises from the distal cervical internal carotid artery (usually between the C1 and C3 vertebral bodies), passes through an enlarged hypoglossal canal, and joins the proximal basilar artery (Figs. 7 and 8). Patients are typically asymptomatic but can uncommonly present with glossopharyngeal neuralgia and hypoglossal paralysis if there is significant mass effect on the hypoglossal nerve.
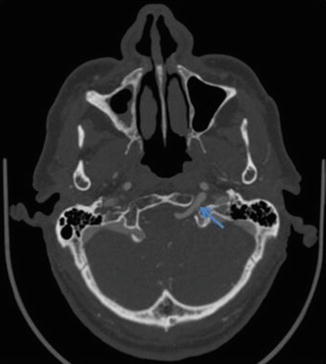
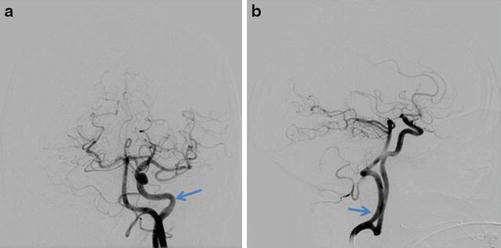
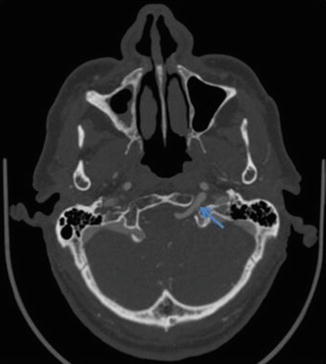
Fig. 7
CTA demonstrating a persistent hypoglossal artery (arrow) traversing through the hypoglossal canal
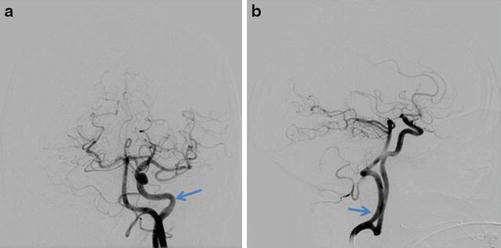
Fig. 8
Left carotid DSA in the same patient as in Fig. 7 with frontal (a) and lateral (b) projections demonstrating a persistent hypoglossal artery (arrow)
The persistent otic artery is exceedingly rare, having only been described in a handful of case reports [1, 3]. It arises from the petrous internal carotid artery, exits via the internal acoustic meatus, and joins the proximal basilar artery.
A fourth variant, the proatlantal intersegmental artery, is similar to the persistent hypoglossal artery in that it arises from the distal cervical internal carotid artery and joins the proximal basilar artery; however, instead of traveling through the hypoglossal canal, the proatlantal intersegmental artery travels through the foramen magnum [1, 5].
Vertebral artery fenestrations occur in 0.2–2.0 % of cerebral angiograms and autopsies [1]. Extracranial fenestrations are thought to arise due to the persistence of the cervical intersegmental arteries while intracranial fenestrations are thought to arise due to the persistence of basivertebral anastomoses. On imaging, vertebral artery fenestrations are characterized by a “gap” in the middle of a vertebral artery; this can be confusing on axial imaging, with visualization of two apparent lumens, but is nicely demonstrated on reformats (Fig. 9). Vertebral artery fenestrations are associated with other vascular anomalies and predispose to vertebral artery aneurysms [1–3]. Basilar artery fenestration is more common than vertebral artery fenestration, occurring in up to 5 % of cerebral angiograms and autopsies, and also predisposes to aneurysms (Fig. 10) [1].
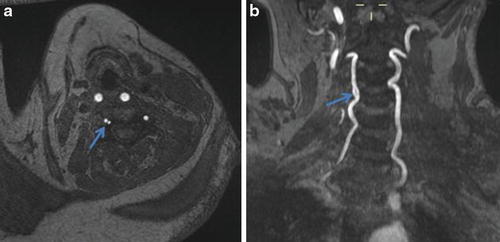
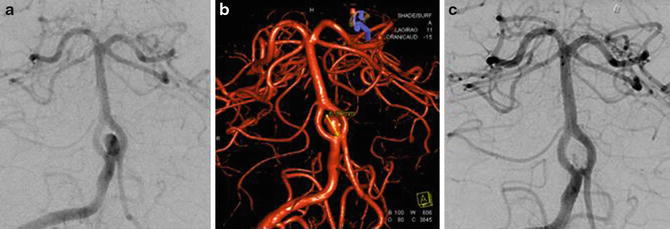
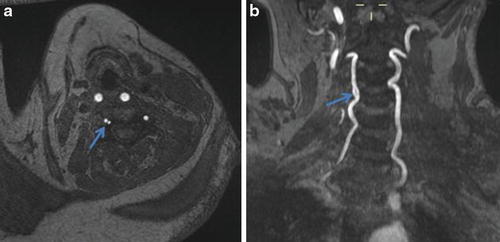
Fig. 9
(a) Axial time-of-flight MRA demonstrates two apparent lumens (arrow) of the right vertebral artery. (b) Coronal time-of-flight MRA maximum-intensity projection reveals that this represents a focal fenestration (arrow) of the V2 segment
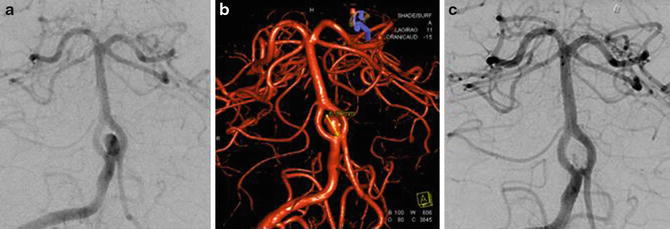
Fig. 10
Right vertebral artery DSA (a) and 3D angiogram (b) demonstrating a fenestrated proximal basilar artery with an associated saccular aneurysm. (c) Right vertebral artery DSA after coil embolization demonstrates no significant filling of the aneurysm sac
Duplication of the vertebral artery is very rare. It is thought to be due to failure of fusion of the cervical intersegmental arteries during development [1].
Anatomy
The vertebral arteries usually arise from the proximal subclavian arteries. However, in approximately 5 % of patients, the left vertebral artery arises directly from the aortic arch (Fig. 11) [5]. The vertebral arteries are typically asymmetric in size, most commonly with the left vertebral artery being dominant. When the vertebral arteries are approximately equal in size, they are considered to be codominant. The normal luminal diameter of the extracranial vertebral artery is approximately 3–5 mm.
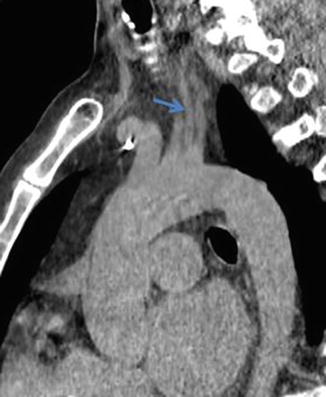
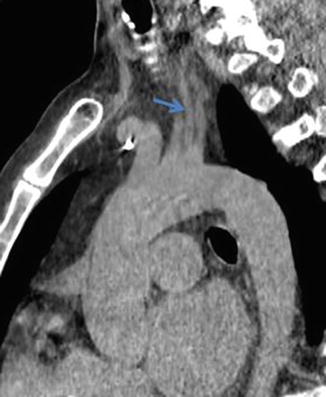
Fig. 11
Oblique sagittal reformat NECT demonstrating the left vertebral artery arising directly from the aorta (arrow)
After arising from the subclavian arteries, the vertebral arteries ascend in the neck and are categorized into four segments, abbreviated V1, V2, V3, and V4 (Fig. 12).
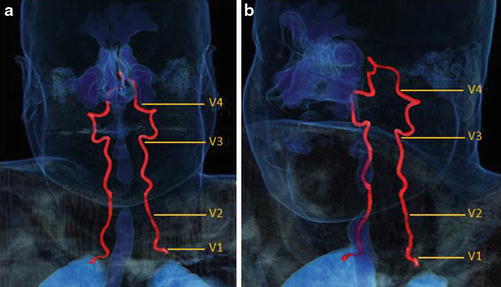
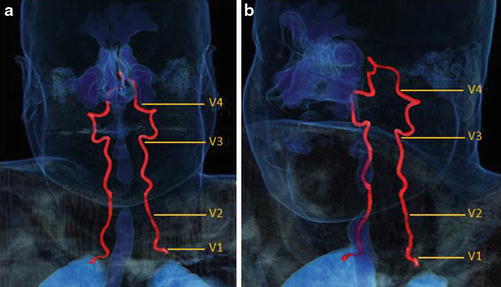
Fig. 12
3D vessel analysis in a normal patient with frontal (a) and oblique (b) projections demonstrating the four standard vertebral artery segments, V1–V4
V1 Segment
The V1 segment is the first segment of the vertebral artery. The V1 segment typically arises from the subclavian artery, courses posteriorly and superiorly in the superior mediastinum, and then enters the C6 transverse foramen to become the V2 segment.
V2 Segment
The V2 segment courses superiorly through the C3–C6 transverse foramina, turns superolaterally through the “inverted L-shaped” C2 transverse foramen, and then exits superiorly through the C2 transverse foramen to become the V3 segment. The V2 segment resides inside the vertebral column (Fig. 13) and is vulnerable to vertebral fractures, especially fractures which violate the transverse foramina.
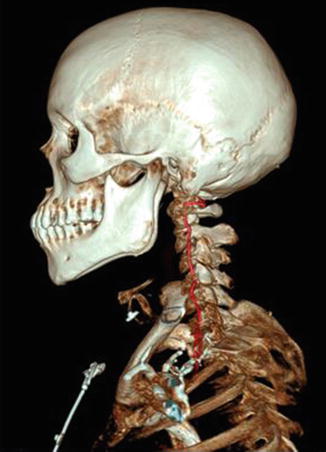
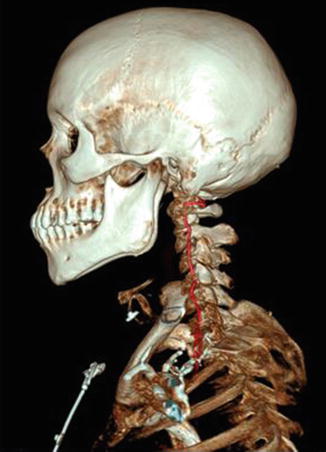
Fig. 13
3D vessel analysis in a normal patient demonstrating the relationship of V2 with the bony cervical vertebral column
V3 Segment
The V3 segment courses posteriorly and medially around the atlantooccipital joint (forming a groove along the posterior ring of the atlas) and then sharply turns anteriorly and superiorly to pierce the dura to become the V4 segment. The V3 segment resides outside the vertebral column and is vulnerable to rotational forces which can injure the artery at its dural insertion.
V4 Segment
The V4 segment courses superiorly, anteriorly, and medially through the foramen magnum and then superomedially behind the clivus. The V4 segments unite to form the basilar artery near the pontomedullary junction.
Along its course, the vertebral artery gives rise to numerous arterial branches: cervical branches, meningeal branches, and intracranial branches. These arteries are described below.
Cervical Branches
The cervical branches originate from the extracranial vertebral artery as it courses up the neck. The cervical branches are categorized into the spinal branches (which supply the spinal cord and vertebral bodies) and the muscular branches (which supply the deep cervical musculature). The muscular branches provide an important collateral pathway in the vertebrobasilar circulation, communicating with the occipital branch of the external carotid artery.
Meningeal Branches
The meningeal branches originate from the distal extracranial vertebral artery. The meningeal branches are categorized into the anterior meningeal artery and the posterior meningeal artery. The anterior meningeal artery arises from V2 and supplies the dura around the foramen magnum. The posterior meningeal artery arises from V3 and supplies the falx cerebellum.
Intracranial Branches
The intracranial branches originate from the intracranial vertebral artery (i.e., the V4 segment). The intracranial branches are categorized into the anterior spinal arteries, the posterior spinal arteries, the posterior inferior cerebellar arteries, and the perforating arteries. The anterior spinal arteries unite in 50 % of cases and course inferiorly in the anteromedian sulcus of the spinal cord to supply the anterior spinal cord. The posterior spinal arteries course inferiorly along the posterolateral spinal cord to supply the posterior spinal cord. The posterior inferior cerebellar arteries course around the medullary tonsils to supply portions of the medulla, inferior cerebellum, and choroid plexus of the fourth ventricle. The perforating arteries arise off the intracranial vertebral artery to supply portions of the medulla, inferior cerebellar peduncles, and olivary bodies.
Variant anatomy of the posterior inferior cerebellar artery is common. Anomalous origin of the posterior inferior cerebellar arteries from V3 occurs in up to 10 % of cases. Duplication of the posterior inferior cerebellar arteries is less common, occurring in approximately 2 % of cases [4]. Occasionally, the posterior inferior cerebellar arteries arise from a single common trunk. Occasionally, the vertebral artery terminates in the posterior inferior cerebellar artery; in this situation, the contralateral vertebral artery supplies the majority of the posterior circulation blood flow [4]. Familiarity with these common variants helps avoid confusion when interpreting the intracranial branches of the vertebral arteries.
Vascular Territories
The vertebrobasilar system supplies the posterior circulation of the brain. As such, vertebrobasilar insufficiency results in neurologic deficits that are significantly different than those incurred by carotid artery insufficiency. Typical symptoms of vertebrobasilar insufficiency include bilateral motor and sensory deficits, nausea, vomiting, vertigo, ataxia, diplopia, dysarthria, and dysmetria [4, 5]. The precise deficits can be predicted based on the vascular territory of the arterial insult.
Cerebellum
The cerebellum is predominantly supplied by three paired arteries: the superior cerebellar arteries, the posterior inferior cerebellar arteries, and the anterior inferior cerebellar arteries. The superior cerebellar arteries supply the superior surface of the cerebellum and the upper vermis. The posterior inferior cerebellar arteries supply the posterior and inferior surfaces of the cerebellum and inferior vermis. The anterior inferior cerebellar arteries supply the petrosal surface of the cerebellum. The central portions of the vermis and cerebellar hemispheres represent a watershed area with variable contributions from all three vessels.
Brainstem
The brainstem is predominantly supplied by the basilar artery and its perforating branches. However, the superior cerebellar arteries supply portions of the superior pons while the distal vertebral arteries and the posterior inferior cerebellar arteries supply portions of the medulla. Occlusion of one of the posterior inferior cerebellar arteries can cause infarction of the lateral medulla, resulting in Wallenberg syndrome. This syndrome is clinically characterized by contralateral sensory deficits affecting the body and extremities, as well as ipsilateral sensory deficits affecting the face.
Imaging
The vertebral arteries can be evaluated with a variety of imaging modalities, including ultrasound, MRA, CTA, and angiography. Ultrasound with pulsed Doppler using a linear 5.0–7.5 MHz probe is a relatively inexpensive examination but is significantly limited by osseous structures, calcified atherosclerosis, patient body habitus, and operator experience. Thus, cross-sectional imaging is typically performed for full evaluation of the vertebral arteries.
Two-dimensional time-of-flight MRA typically provides satisfactory visualization of the vertebral arteries. A superior saturation band is commonly applied to eliminate jugular venous flow. However, it should be noted that a superior saturation band can potentially hide reversed vertebral artery flow. Thus, in cases of suspected subclavian steal syndrome or bow hunter’s syndrome, a phase contrast sequence can be performed to unmask reversal of flow. The general limitations of time-of-flight MRA include overestimation of stenosis in the setting of vessel calcification, vessel tortuosity, or slow flow. Dynamic contrast-enhanced MRA is able to overcome many of these limitations but is still prone to poor spatial resolution, patient motion, and metal artifacts.
An alternative to MRA is CTA, which provides excellent visualization of the vertebral arteries with superior spatial resolution and shorter imaging times. In addition, CTA is generally offered day and night at most facilities. The scanning range typically includes the aortic arch to the circle of Willis in order to visualize the whole length of the vertebral arteries. Automatic triggering or test bolus techniques can be used to time the contrast injection. An adjunct non-contrast and post-contrast head CT is often performed with the CTA. One weakness of CTA is that it tends to overestimate arterial stenosis in the setting of heavy vessel calcification. In addition, the direction of arterial flow cannot be determined with conventional CT imaging.
Angiography has historically been considered the “gold standard” in vertebral artery imaging but is now rarely performed as the initial imaging examination. In the modern era, angiography is typically reserved for resolving diagnostic uncertainties, planning interventions, or performing therapeutic procedures. Vertebral artery angiography is typically performed with rapid sequences during subclavian artery injections. Selective imaging can also be obtained, usually in the AP, lateral views, and oblique views. Angiography carries a small inherent risk of vascular injury or distal embolization. The risk of TIA and stroke is approximately 3 % and <1 % respectively with a cerebral angiogram examination [4].
The ideal imaging modality for the evaluation of the vertebral arteries depends on the clinical context. In cases of suspected vertebral artery occlusion or dissection, CTA of the head and neck is a reasonable initial examination, providing rapid visualization of the vertebral arteries with excellent spatial resolution, thus potentially minimizing the time to revascularization. However, in cases of subclavian steal syndrome or bow hunter’s syndrome, dynamic imaging with contrast-enhanced MRA may be preferred. Finally, in patients with tenuous renal function, time-of-flight MRA may be selected in order to avoid contrast agents and the risk of nephrogenic systemic fibrosis.
Atherosclerotic Disease
Epidemiology and Clinical Findings
The most common nontraumatic condition affecting the extracranial vertebral arteries is atherosclerotic disease [6]. Atherosclerosis is a systemic arterial disease, prevalent in industrialized nations. It is responsible for the majority of ischemic strokes, resulting in healthcare-associated costs over $30 billion each year [7]. There are multiple well-known risk factors for atherosclerosis, both modifiable and nonmodifiable. Common nonmodifiable risk factors include family history, age, and male gender. Common modifiable risk factors include age, hypertension, hyperlipidemia, diabetes, smoking, and renal failure (Table 1) [8].
Table 1
Risk factors for atherosclerotic disease
Nonmodifiable | Family history |
Age | |
Male gender | |
Modifiable | Hypertension |
Hyperlipidemia | |
Diabetes | |
Smoking | |
Renal failure |
There is a strong association between vertebral artery atherosclerosis and carotid artery atherosclerosis; however, the vertebral arteries tend to be less severely and less diffusely affected than the carotid arteries [6].
Most patients with vertebral artery atherosclerosis are asymptomatic; however, if the degree of vertebral artery stenosis is significant, patients can present with vertebrobasilar insufficiency which can manifest as vertigo, ataxia, imbalance, syncope, and cranial nerve deficits [8]. Patients can also present with a myriad of non-neurologic symptoms related to systemic atherosclerosis, including angina from coronary artery disease and claudication from peripheral vascular disease.
Pathophysiology
Atherosclerosis is a complex pathologic process with endothelial injury playing a central role in its pathogenesis [8, 9]. Endothelial injury results in increased permeability of the arterial wall to lipids, which deposit in the intima resulting in the fibrofatty plaque. Microscopically, the fibrofatty plaque is composed of lipids, foam cells, and connective tissue. A fibrotic cap, composed of smooth muscle and collagen, forms over the surface of the fibrofatty plaque. Disruption of the fibrotic cap results in exposure of the thrombogenic fibrofatty plaque to the bloodstream, which can result in acute thrombosis and/or thromboembolus [9]. In addition, as the fibrofatty plaque enlarges, it progressively narrows the arterial lumen. In general, a 50 % decrease in luminal diameter is required before hemodynamic consequences occur, as compensatory physiologic vasodilation mitigates the effects of mild atherosclerotic disease [9]. It should be noted that tandem stenoses can be additive in their hemodynamic effects, much like serial resistances in a circuit [8]. Ultimately, clinical symptoms occur when the arterial demand outstrips the arterial supply, resulting in a demand-supply mismatch and end-organ ischemia. If the mismatch is transient and infarction has not occurred, then the clinical manifestations are transient and the episode is referred to as a transient ischemic attack. However, if the mismatch is prolonged and infarction has occurred, then the clinical manifestations are prolonged and the episode is referred to as a cerebrovascular accident or stroke. Patients with prior transient ischemic attacks have a ten times increased risk of stroke [9].
Imaging Findings
Vertebral artery stenosis severity is categorized per the North American Symptomatic Carotid Endarterectomy Trial (NASCET) method [10]. The NASCET method defines the percent stenosis as the ratio of the difference between the normal and diseased luminal diameter to the normal luminal diameter (i.e., percent stenosis = (normal lumen − minimal diseased lumen) / (normal lumen) × 100%). Flow-limiting atherosclerotic disease is graded by the percent stenosis, with mild narrowing <50 %, moderate narrowing 50–70 %, and severe narrowing >70 % (Table 2). Irregular atheromatous plaques are at an increased risk of thromboembolism and are considered an independent risk factor for stroke [4].
Table 2
Grading of atherosclerotic disease
Mild | <50 % |
Moderate | 50–69 % |
Severe | ≥70 % |
Ultrasound with pulsed Doppler has a very limited role in evaluating vertebral artery atherosclerosis, largely due to its inability to visualize the vertebral artery in its entirety. As with other blood vessels, arterial stenosis is characterized on ultrasound by increased velocities, turbulence, high-resistance waveforms, and elevated resistive indices [6, 8, 11]. The post-stenotic segment is characterized by decreased velocities, loss of pulsatility, “parvus-tardus” waveforms, and decreased resistive indices. Severe stenosis in one vertebral artery can result in overestimation in stenosis of the contralateral vertebral artery due to compensatory increased blood flow [9].
CTA or MRA is often the initial diagnostic examination for vertebral artery atherosclerosis, with >90 % sensitivity and >90 % specificity [5, 8, 9]. Both imaging modalities offer complete visualization of the vertebral arteries and allow for routine NASCET characterization of atherosclerotic disease. In general, atherosclerosis is characterized by smooth or irregular plaques resulting in luminal narrowing, often with concomitant arterial wall calcification [8]. Atherosclerotic disease is typically most severe at the ostia of the vertebral arteries (Fig. 14). Multiplanar reformats and/or 3D vessel analysis can be useful in accurately quantifying the degree of arterial stenosis, especially in vessel segments which do not run orthogonal to the axial plane.
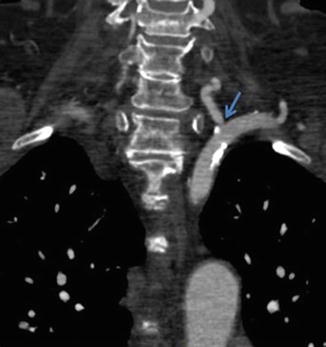
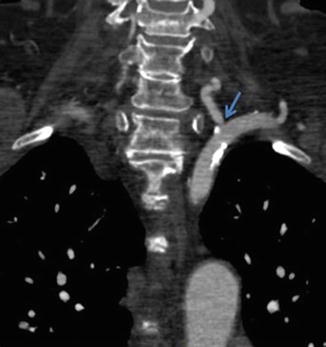
Fig. 14
CTA demonstrating moderate stenosis of the left vertebral artery origin (arrow) due to ostial calcified atherosclerotic disease
Advanced atherosclerotic disease can manifest as near-occlusion or occlusion of the vertebral artery (Figs. 15 and 16 respectively). However, if the clinical suspicion for significant atherosclerosis is low (e.g., young patient without atherosclerotic risk factors), other causes of vertebral artery stenosis should be considered, such as vertebral artery dissection, radiation arteritis, fibromuscular dysplasia, or vasculitis [8]. In these cases, MRA may provide additional information by directly visualizing fibrofatty atherosclerotic plaque and excluding other causes of luminal narrowing (Fig. 17). Lastly, the role of DSA in evaluating atherosclerotic disease is generally reserved for planning for endovascular interventions (Fig. 18).
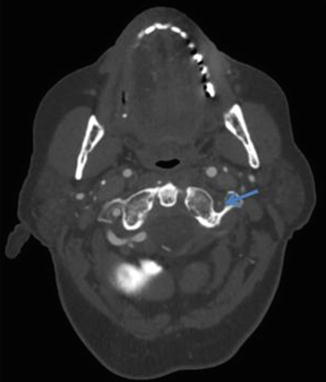
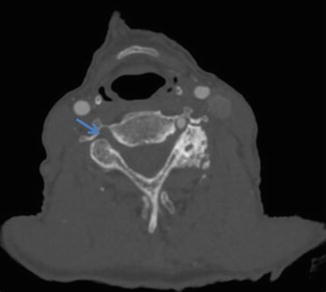
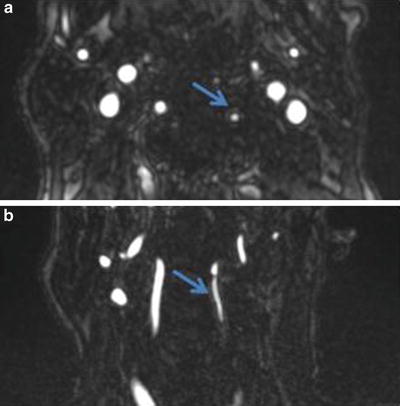
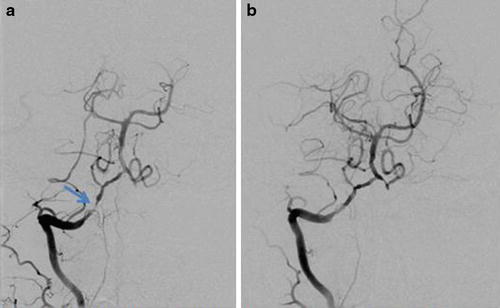
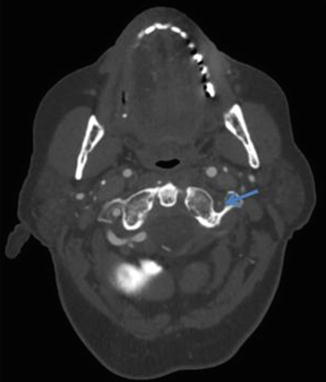
Fig. 15
CTA demonstrating severe stenosis of the left extracranial vertebral artery (arrow) due to atherosclerotic disease
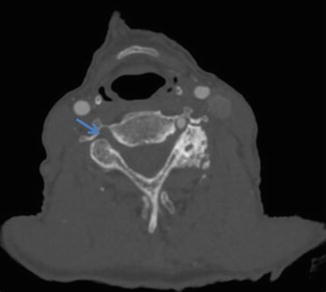
Fig. 16
CTA demonstrating complete occlusion of the right vertebral artery (arrow)
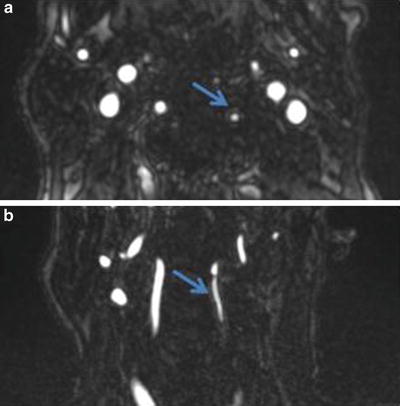
Fig. 17
Axial (a) and coronal (b) MRA of the neck demonstrating a fibrofatty atherosclerotic plaque (arrows) of the left vertebral artery
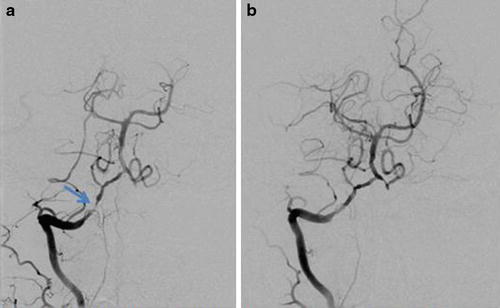
Fig. 18
(a) Right vertebral artery DSA demonstrates a high-grade stenosis of V4. (b) Right vertebral artery DSA after angioplasty and placement of a 2 × 8 mm chromium cobalt stent demonstrates an excellent angiographic result
Work-Up and Management
The management of vertebral artery atherosclerotic disease has historically been with medical therapy targeting atherosclerotic risk factors such as hypertension or hypercholesterolemia. Surgery, while frequently performed for carotid artery atherosclerosis, is rarely performed for vertebral artery atherosclerosis [12]. With the advent of minimally invasive endovascular techniques, angioplasty and stenting now have a role in patients who fail maximum medical therapy. Endovascular treatment involves aggressive procedural anticoagulation and deployment of a self-expanding stent across the stenotic lesion. The angiographic success rate of endovascular techniques has been reported to be up to 95 %, with a stroke rate of <2 % [9]. However, endovascular stenting suffers from restenosis and neointimal hyperplasia which often occurs at the margin of previously placed stents (Fig. 19) [5]. Drug-eluting stents and other methods of reducing restenosis and neointimal hyperplasia are currently being developed and studied.
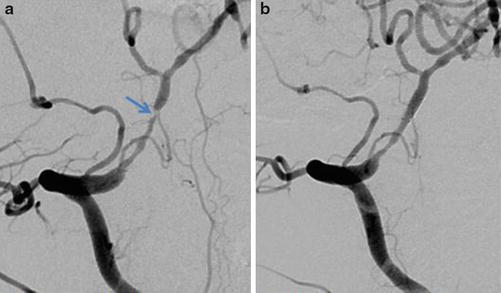
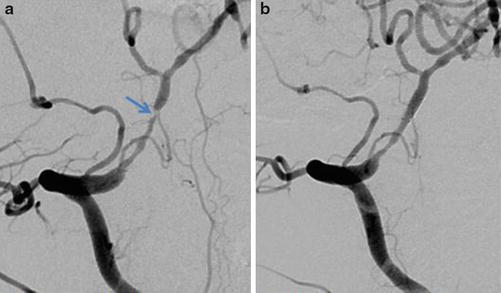
Fig. 19
(a) Right vertebral artery DSA 2 years later in the same patient as in Fig. 18 demonstrates a >90 % stenosis at the distal aspect of the previously placed stent. (b) Right vertebral artery DSA after angioplasty and placement of a second 2 × 8 mm chromium cobalt stent demonstrates an excellent angiographic result
Vertebral Artery Dissection
Epidemiology and Clinical Findings
Vertebral artery dissection has an annual incidence of 1 per 100,000 patients and is one of the most common causes of stroke in young adult patients [4]. Vertebral artery dissection constitutes approximately 27 % of all craniocervical arterial dissections and causes up to 40 % of all posterior fossa ischemic strokes [4, 13]. The mean age at presentation is approximately 45 years; there is a slight male predominance [4, 14, 15]. Patients can present with dizziness/vertigo, headache, neck pain, ataxia, visual symptoms, nausea/vomiting, nystagmus, Horner syndrome, sensory deficits, cranial nerve palsies, dysphagia, and tinnitus (Table 3) [13, 16]. In a meta-analysis of nearly 2,000 patients, Gottesman et al. found that the most common symptom was dizziness/vertigo (58 %), followed by headache (51 %) and neck pain (48 %) [16]. However, it should be noted that nearly one in four patients with proven vertebral artery dissection have no headache or neck pain at the time of diagnosis; thus, the absence of these symptoms do not exclude the diagnosis.
Dizziness/vertigo | 58 % |
Headache | 51 % |
Neck pain | 46 % |
Ataxia | 38 % |
Visual symptoms | 36 % |
Nausea/vomiting | 35 % |
Nystagmus
![]() Stay updated, free articles. Join our Telegram channel![]() Full access? Get Clinical Tree![]() ![]() ![]() |