Fig. 1
Intracranial atherosclerotic stenoses diagnosed by transcranial Doppler imaging (TCDI) and digital subtraction angiography (DSA). (a) DSA (center), 58 % stenosis of the M1 segment of the middle cerebral artery (MCA); TCDI, normal mean flow velocity (MFV) in the MCA at a depth of 60 mm (insert on the left) and high MFV in the mid-MCA at a depth of 49 mm (insert on the right), indicating a ≥50 % MCA stenosis. (SPR in the figure indicates stenotic-to-prestenotic ratio). (b) DSA (center), 71 % mid-basilar artery (BA) stenosis; TCDI, normal MFV in the BA at a depth of 85 mm (insert on the left) and high MFV in the BA at a depth of 94 mm (insert on the right), indicating a high-grade (≥70 %) mid-BA stenosis. (c) DSA (center), 84 % proximal BA stenosis; TCDI, delayed systolic upstroke in both proximal and mid-BA with MFVs of 20 and 23 cm/s, indicating a most severe or elongated stenosis (Adapted from Zhao L, Barlinn K, Sharma VK, Tsivgoulis G, Cava LF, Vasdekis SN, Teoh HL, Triantafyllou N, Chan BP, Sharma A, Voumvourakis K, Stamboulis E, Saqqur M, Harrigan MR, Albright KC, Alexandrov AV (2011) Velocity criteria for intracranial stenosis revisited: an international multicenter study of transcranial Doppler and digital subtraction angiography. Stroke 42: 3429–3434. doi: 10.1161/strokeaha.111.621235; with permission)
Besides the most common application of TCDI in grading luminal stenosis of ICAS, it could also be used in the following manners. Quantified vasomotor reactivity by TCDI could represent the status of cerebral autoregulation, which probably is globally impaired in patients with symptomatic ICAS, and also a risk factor for stroke [16]. MES revealed by TCDI supports the theory that artery-to-artery embolization may be a potential mechanism of ICAS-related ischemic stroke, and it has been found to be related to the worsening of neurological deficits in acute ischemic stroke and also independently correlated to recurrent events in ischemic stroke due to ICAS [16]. In addition, recent studies suggested that the steal phenomenon termed “reversed Robin Hood syndrome” revealed by TCDI, referring to arterial blood flow stolen from ischemic to non-affected brain tissues, could be a possible mechanism for an early deterioration of acute ischemic stroke and correlated with the risk of stroke recurrence independent of stroke subtypes [19].
In recent years, TCCD (Fig. 2) has become a standard diagnostic technique to evaluate intracranial arteries in ischemic stroke patients at some centers and has been increasingly used in guiding thrombolysis of acute stroke [20]. The combination of flow and anatomic information of intracranial arteries and adjacent structures enhanced the diagnostic accuracy of the severity of luminal stenosis in ICAS, as compared with routine TCDI. Moreover, the use of contrast in TCCD yields higher diagnostic value than TCDI in patients with insufficient temporal acoustic window [20].
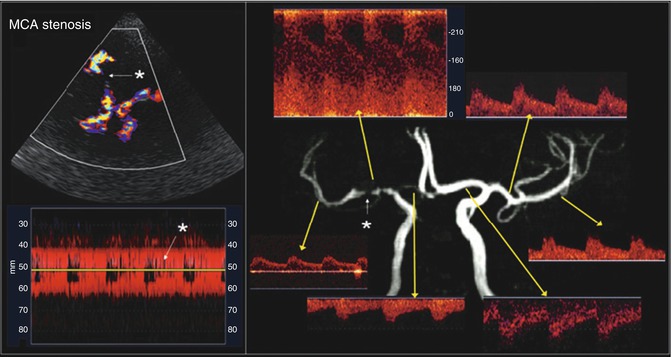
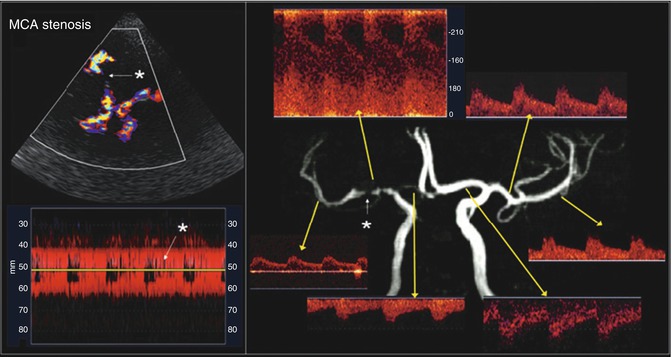
Fig. 2
Appearance of a critical right middle cerebral artery (MCA) stem stenosis (white star) on transcranial color-coded duplex (TCCD) (upper left) and power motion-mode (PMD) transcranial Doppler imaging (TCDI) (lower left). Flow disturbance is easily discernible on both TCCD and PMD. TCDI waveforms of the diseased right MCA and other cerebral arteries are displayed with a corresponding magnetic resonance angiography image (right). There is significantly increased mean flow velocity (MFV, 220 cm/s) at the stenosis region and decreased MFV (40 cm/s) and delayed upstroke (0.25 s) at the post-stenotic region (Adapted from Topcuoglu MA (2012) Transcranial Doppler ultrasound in neurovascular diseases: diagnostic and therapeutic aspects. J Neurochem 123 Suppl 2: 39–51. doi: 10.1111/j.1471-4159.2012.07942.x; with permission)
Real-time flow monitoring by TCDI or TCCD yields an economic and effective method for detecting the recanalization of occluded arteries in acute ischemic stroke (Fig. 3) and is possibly related to stroke severity and mortality [16]. In the past few years, TCDI and TCCD have also been used to enhance the thrombolytic activity of intravenous tissue plasminogen activator (tPA), which was found to be efficient and safe. As compared with tPA alone, tPA plus high-frequency TCDI/TCCD may correlate with higher rates of complete recanalization, without increasing the risk of symptomatic intracranial hemorrhage [21].
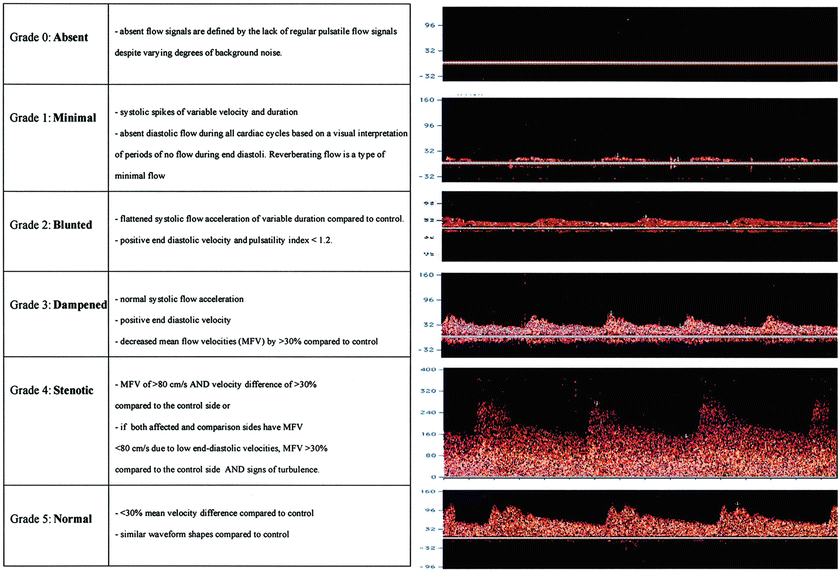
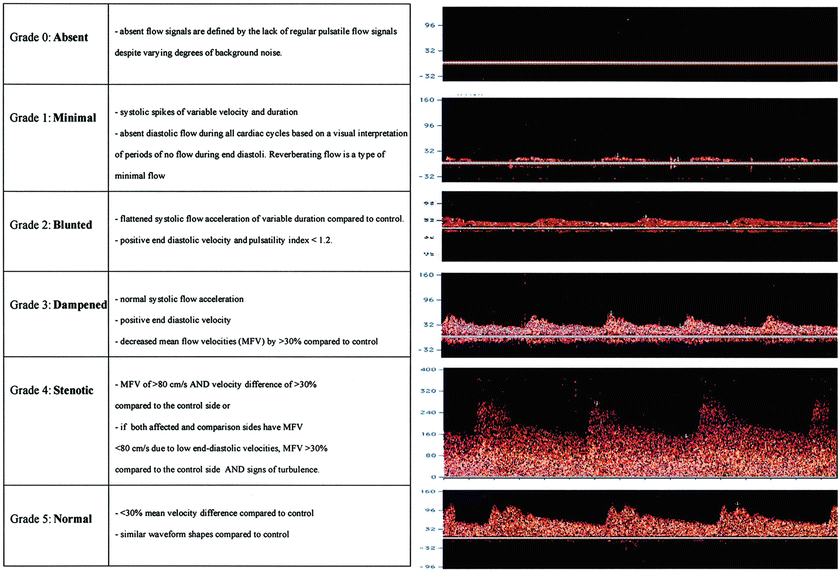
Fig. 3
Description and illustration of the thrombolysis in brain ischemia (TIBI) transcranial Doppler imaging (TCDI) flow grades (Grades 0–5) to classify residual flow through an occluded intracranial artery. Emergent TCDI TIBI classification was found to be correlated with initial stroke severity, clinical recovery, and mortality in stroke patients treated with intravenously administered tissue plasminogen activator (Adapted from Demchuk AM, Burgin WS, Christou I, Felberg RA, Barber PA, Hill MD, Alexandrov AV (2001) Thrombolysis in Brain Ischemia (TIBI) transcranial Doppler flow grades predict clinical severity, early recovery, and mortality in patients treated with intravenous tissue plasminogen activator. Stroke 32: 89–93. doi: 10.1161/01.STR.32.1.89; with permission)
TCDI, as a noninvasive and relatively inexpensive imaging method, is widely available throughout the world. With its unique advantages as mentioned above, TCDI could be a useful tool to evaluate hemodynamic characteristics of symptomatic ICAS, and some of the characteristics could be potential predictors for stroke severity and the risk of recurrence in patients with symptomatic ICAS. However, TCDI is a highly operator-dependent examination; inconsistency of experience and skills of different operators may impact on its diagnostic accuracy. Also, insufficiency of temporal windows, especially in elder females, leads to underestimation of diseased intracranial arteries [16].
MRA
Several different techniques or sequences of MRA, a noninvasive imaging modality, have been applied in clinical practice to evaluate the cervicocerebral vasculature during the past decades [22]. Among all these techniques, time-of-flight MRA (TOF-MRA) is the most commonly used MRA technique in clinical investigation of intracranial arteries. In addition, phase-contrast MRA (PC-MRA), contrast-enhanced MRA (CE-MRA), and quantitative MRA (QMRA) could also be used to reveal anatomic severity of luminal stenosis as well as flow information in the case of ICAS. Besides depicting vessel structures, most of these MRA techniques bear flow information as well, which is a particular advantage of this imaging modality over others. However, in the case of symptomatic ICAS, MRA techniques may not be available in acute settings, at least at primary or secondary medical centers, which limit its values in acute evaluation of such lesions.
TOF-MRA (Fig. 4) is an angiographic method without using any contrast agents, which could be widely used in patients with contraindications to contrast agents, such as renal failure and relevant allergic reactions. According to the SONIA trial, the positive and negative predictive values for TOF-MRA to diagnose a 50–99 % intracranial stenosis were 59 % (95 % CI: 54–65 %) and 91 % (95 % CI: 89–93 %), with DSA being the reference standard [14]. Thus, TOF-MRA could be a reliable tool to rule out an arterial stenosis in ICAS, but positive diagnosis of luminal stenosis by TOF-MRA needs to be confirmed by other imaging methods, which is similar to that of TCDI as mentioned above. This could be due to the signal contrast mechanism of TOF-MRA, flow-related enhancement, which may lead to an overestimation of the severity of luminal stenosis in lesions with slow or minimal flow. Although the flow-dependent nature of TOF-MRA may hinder its ability to scale the luminal stenosis in the case of ICAS, it could to a certain extent reveal the hemodynamic impact of an ICAS lesion. So the inherent flow information yields a unique advantage of this imaging method over some of the others, which is further discussed below [15].
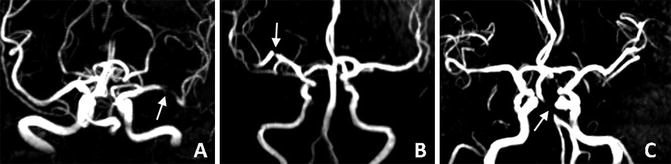
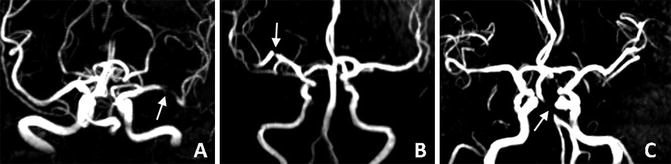
Fig. 4
Intracranial atherosclerotic lesions at the left middle cerebral artery (a, arrow), right middle cerebral artery (b, arrow), and basilar artery (c, arrow), as revealed by time-of-flight magnetic resonance angiography
Like TOF-MRA, PC-MRA does not need contrast agents either, though the latter technique is not as commonly used as the former one in clinical practice to evaluate intracranial atherosclerotic disease. By using PC-MRA, not only the arterial structure could be revealed, flow velocities and cerebral blood flow could also be quantified, based on phase shifts of flowing protons within the vessel lumen. By using TOF-MRA to facilitate three-dimensional vessel localization, PC-MRA-based QMRA could significantly improve the accuracy of PC-MRA to quantify cerebral blood flow, which is detailed below [23].
In addition to the contrast agent-independent MRA techniques as above, CE-MRA is also frequently used in clinical practice to simultaneously visualize the entire supra-aortic vasculature, including extracranial and intracranial arteries. With contrast injection, CE-MRA could better delineate vessel structures as compared with TOF-MRA, but the diagnostic accuracy of arterial stenoses by CE-MRA was lower for intracranial arteries than that for extracranial arteries. Further, CE-MRA does not bear flow information in the evaluation of ICAS, unlike TOF- and PC-MRA.
CTA
Routine single-phase CTA has been increasingly used in the clinical evaluation of ICAS during the past few years (Fig. 5). As a minimally invasive imaging modality, routine CTA could yield a useful tool for scaling the severity of luminal stenosis in ICAS. By using intravenous contrast, CTA could better depict the vessel geometry as compared with MRA, but delineation of some segments of the intracranial vasculature, such as the petrous and cavernous portions of internal carotid artery, and the distal vertebral artery, may be affected by surrounding bones, though the associated artifacts could be improved by proper post-processing techniques.
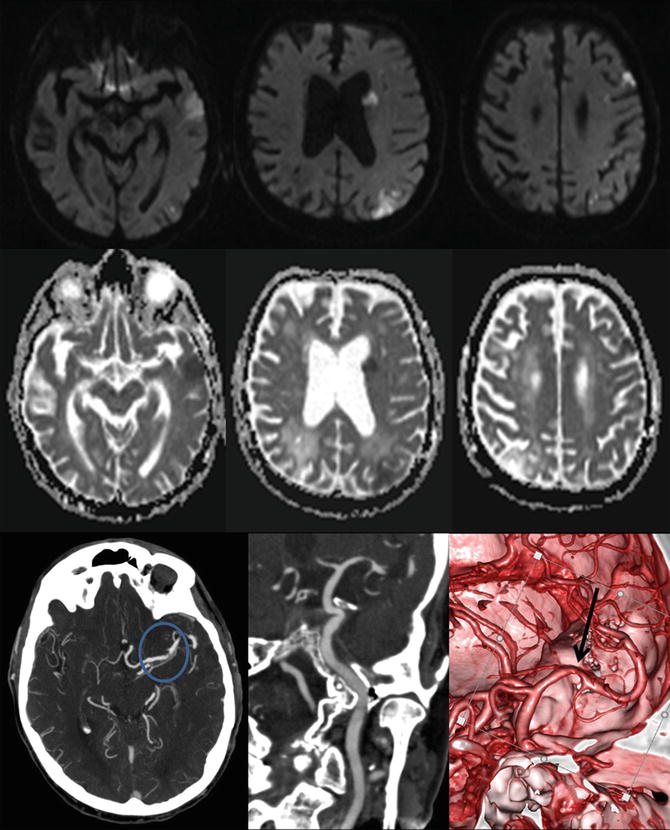
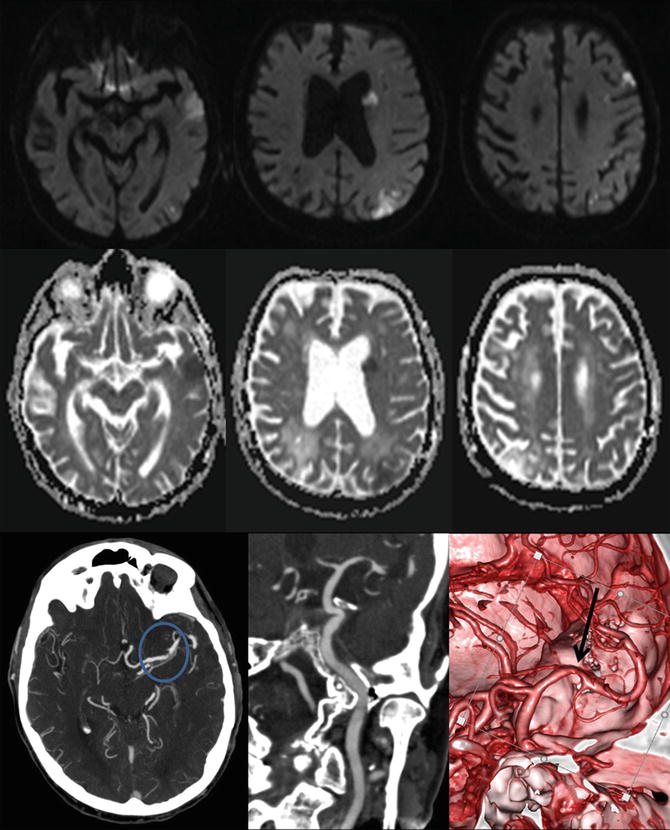
Fig. 5
Intracranial atherosclerosis on computed tomography angiography (CTA). This patient presented with multiple acute ischemic lesions in the left hemisphere as shown in the diffusion-weighted imaging (DWI) and apparent diffusion coefficient (ADC) maps. Notice the old right parietal infarct. The CTA demonstrates a tight stenosis at the left M1 segment of the middle cerebral artery. The 3D reconstruction better delineates the finding and the vessel morphology
Regarding the diagnostic ability of CTA for defining the severity of luminal stenosis in ICAS, there have been no large prospective studies. According to some small studies, CTA probably has higher diagnostic accuracy than MRA to define an ICAS lesion of 50–99 % luminal stenosis. However, based on results from the SONIA trial, the positive predictive value of CTA to define a 50–99 % intracranial stenosis was low, almost comparable to that of MRA and TCDI [24]. The SONIA trial suggested that, like MRA or TCDI, CTA was also a useful tool to rule out the presence of arterial stenosis due to ICAS but not as much reliable in scaling the severity of luminal stenosis, with DSA as the reference standard, while the results may be biased by the fact that only a small part of subjects analyzed in the SONIA trial underwent CTA examinations. Thus, further prospective large studies should be performed to validate the diagnostic accuracy of CTA for detecting arterial stenosis by ICAS.
Additionally, CTA could also be used to evaluate the thrombus burden in intracranial atherosclerotic disease, for instance, the clot burden score (CBS), which has been found to be able to predict functional outcome, final infarct size, and the parenchymal hematoma risk [25]. Further, CTA source images (CTA-SI) are very useful in the assessment of tissue status in acute ischemic stroke. Ischemic brain tissues with low cerebral blood flow are revealed as regions without the enhancement on CTA-SI, which could be differentiated from brain tissues with normal cerebral blood flow. By using the Alberta Stroke Program Early CT Score (ASPECTS) on CTA-SI, acute ischemic stroke within the middle cerebral arterial territory could be more reliably identified, and the final infarct volume and clinical outcomes could be more accurately predicted, as compared with non-contrast CT [26].
Moreover, CTA could be used to evaluate primary and secondary collaterals (Fig. 6). For instance, there have been several methods to evaluate leptomeningeal collaterals by CTA-SI or reconstructed CTA, the interobserver agreement of which methods ranged from moderate to excellent [18]. Multiple studies have demonstrated the protective role of good collaterals as revealed by CTA in clinical outcomes of stroke patients [26]. However, till now, there have been no commonly accepted methods using CTA to evaluate collateral circulation in the case of symptomatic ICAS that has been validated in large prospective studies. The inconsistency of these methods used for evaluating collaterals may hinder the generalized use of this minimally invasive imaging modality in multicenter studies to fully appreciate the impact of collateral flow in the case of symptomatic ICAS.
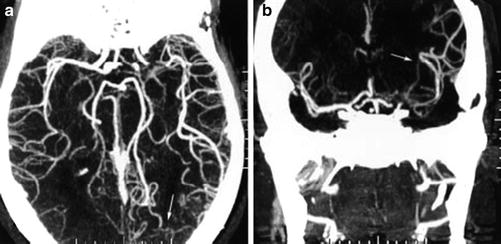
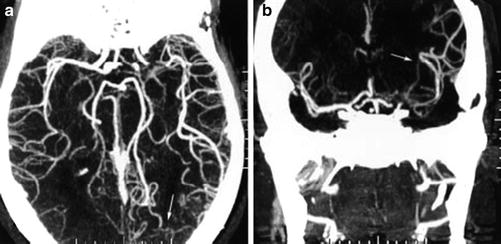
Fig. 6
Axial (a) and coronal (b) maximum intensity projections of computed tomography angiography demonstrating leptomeningeal collateral blood flow from the posterior cerebral artery (arrows) to distal segments of an occluded middle cerebral artery (From Liebeskind DS (2003) Collateral circulation. Stroke 34: 2279–2284. doi: 10.1161/01.STR.0000086465.41263.06)
Although CTA has multiple applications in the evaluation of ICAS, it tends to eliminate any temporal flow information through the lesions, which by itself is not a good tool for evaluating hemodynamics of ICAS lesions. But it may be a good source for the reconstruction of blood flow models of ICAS by using the computational fluid dynamics (CFD) technique, which is discussed below [15]. Another limitation of this imaging method is that it is contrast agent dependent, which is contraindicated in patients with renal failure or allergic reactions to the contrast agent.
DSA
DSA (Fig. 7), with its superb spatial resolution, is regarded as the gold standard to diagnose the severity of luminal stenosis, flow direction, and collaterals in ICAS. It has been used in enormous studies as the reference standard to validate the diagnostic accuracies of other imaging modalities to assess the intracranial vasculature, for instance, in the SONIA trial [14]. It has also been used in large clinical trials to determine the severity of luminal stenosis by ICAS, for the sake of patient selection for the investigation of certain treatment strategies, for example, the WASID and the SAMMPRIS trials [9, 10]. The WASID criteria for measuring the percentage of luminal stenosis in ICAS are now widely used in clinical practice and relevant research areas. Further, although there are still controversies in applying interventional therapy in symptomatic ICAS, when employed, the severity of luminal stenosis defined by DSA is usually used to guide clinical decisions or to guide patient selection in the setting of clinical trials [27].
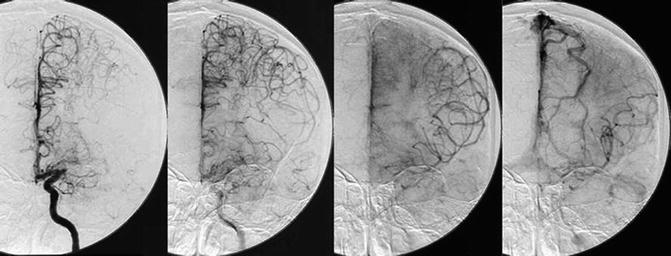
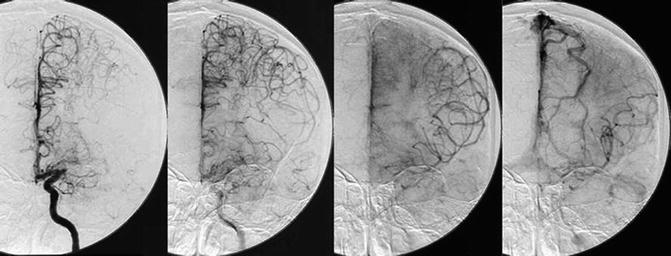
Fig. 7
Serial angiographic images from a selective left internal carotid artery injection in a patient who has middle cerebral artery occlusion, illustrating retrograde collateral flow. (© David S. Liebeskind, MD.)
Moreover, DSA may be used to evaluate and grade collateral status in symptomatic ICAS. There have been multiple methods using DSA to evaluate the collateral circulation, including collaterals of the Circle of Willis and leptomeningeal collaterals. For instance, the American Society of Interventional and Therapeutic Neuroradiology/Society of Interventional Radiology (ASITN/SIR) Collateral Flow Grading System (Table 1) is one of the most commonly used grading methods to gauge the function of collateral circulation. However, there were few studies investigating the interobserver reproducibility of methods utilizing DSA to grade collateral circulation in the case of ICAS. According to the limited existing data, several of these methods were of good and very good interobserver agreement [18]. Collateral status graded by DSA has been found to be able to alter the risk of stroke recurrence in ischemic stroke or TIA due to ICAS, in the WASID cohort and other studies as well [15]. With the collateral status identified as an independent predictor for recurrent stroke, probably surpassing the role of percent stenosis, in patients with symptomatic ICAS [28], there is a great need for the establishment and validation of generalizable grading methods, so that the role of collateral circulation could be further explored in larger, multicenter clinical studies.
Table 1
The ASITN/SIR collateral flow grading system
Grade 0 | No collaterals visible to the ischemic site |
Grade 1 | Slow collaterals to the periphery of the ischemic site with persistence of some of the defect |
Grade 2 | Rapid collaterals to the periphery of ischemic site with persistence of some of the defect and to only a portion of the ischemic territory |
Grade 3 | Collaterals with slow but complete angiographic blood flow of the ischemic bed by the late venous phase |
Grade 4 | Complete and rapid collateral blood flow to the vascular bed in the entire ischemic territory by retrograde perfusion |
DSA may differentiate antegrade and retrograde flow by directly revealing the flow directions. However, with its invasive nature, DSA could result in periprocedural complications, including transient or even permanent neurological deficits in rare cases, risk of which may be operator dependent. Also, the DSA procedure is relatively more expensive than other imaging methods. Thus, DSA could not be widely used in clinical scenarios, especially in primary and secondary medical centers [29]. Moreover, the superb resolution of DSA to depict intracranial arteries may be affected by slow flow in severely stenosed arteries that prevent or slow the contrast agents to fill in the vessel, which sometimes may lead to the overestimation of the severity of luminal stenosis or the hemodynamic impact of an ICAS lesion.
Routine CT and MR Perfusion Imaging
In the presence of an occluded intracranial artery, downstream cerebral blood flow may fall below the threshold of 20 mL/100 g tissue/min, under which the function of neurons is impaired. If the cerebral blood flow falls below 12 mL/100 g tissue/min, irreversible damages could happen to neuronal cells [30]. Thus, hypoperfusion has been identified as an important mechanism for ischemia in patients with symptomatic ICAS, with affected brain tissues becoming the infarct core or ischemic penumbra, depending on the extent of hypoperfusion in different ischemic areas. The proportion of salvageable tissues, or the ischemic penumbra, and the infarct core, in the case of acute ischemic stroke, to a great extent depends on the balance between the residual antegrade flow across the occlusive artery and retrograde flow via collaterals. Therefore, routine perfusion imaging methods CTP and DSC-PWI, respectively, based on the imaging modalities of CT and MRI, is valuable in evaluating the perfusion status of focal and downstream brain tissues, and hence reflecting the collateral status, in patients with acute symptomatic ICAS [17].
The potentially salvageable ischemic penumbra is an ideal target for reperfusion therapies which require proper patient selection, while the mismatch between the hypoperfused area as revealed by CT and MR perfusion imaging and the acute infarct core serves as a reliable tool for the identification of the penumbra [27]. The mismatch between hypoperfused area on DSC-PWI and the ischemic area on diffusion-weighted imaging (DWI) (Fig. 8), and the mean transit time-cerebral blood volume mismatched by CTP (Fig. 9), has been tested in several large clinical trials to guide selection of patients indicated for thrombolysis beyond the 3-h time window. Although definite clinical benefits of imaging-guided thrombolysis in acute ischemic stroke have not yet been demonstrated in these trials, there was a trend that imaging-detected mismatch might be correlated to increased reperfusion [27]. According to the most up-to-date guideline, CTP or DSC-PWI may be considered for the selection of patients for acute reperfusion therapy beyond the time windows for intravenous fibrinolysis [27]. However, substantial heterogeneities in the acquisition and post-processing protocols for CT and MR perfusion imaging in exiting studies may limit comparisons between studies and generalization of the study results. Thus, further studies are warranted and needed to validate the role of these two perfusion imaging methods in defining the penumbra for thrombolytic therapy.
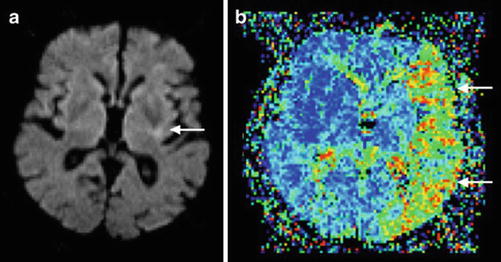
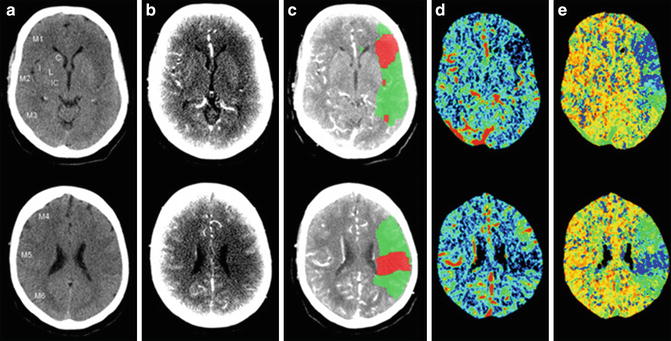
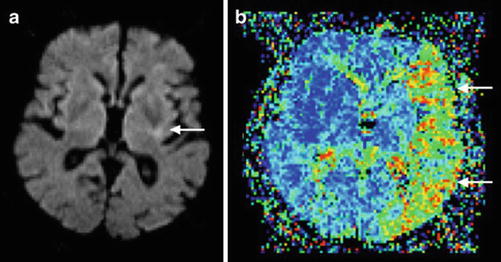
Fig. 8
MR perfusion/diffusion mismatch in a patient with left middle cerebral artery occlusion. Note the small area of infarct core (arrow) in the diffusion-weighted image (DWI; a) in comparison with the large area of perfusion abnormality (arrows) in mean transit time (MTT; b) resulting in positive mismatch with a large penumbra (Adapted from Kloska SP, Wintermark M, Engelhorn T, Fiebach JB (2010) Acute stroke magnetic resonance imaging: current status and future perspective. Neuroradiology 52: 189–201. doi: 10.1007/s00234-009-0637-1; with permission)
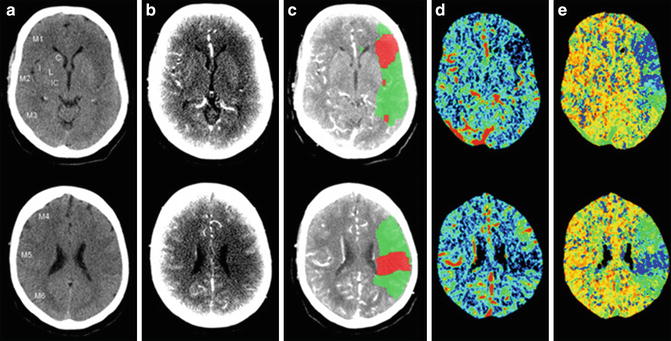
Fig. 9
Mean transit time (MTT, e)-cerebral blood volume (CBV, d) mismatch in a patient with acute ischemic stroke, approximately 1 h after symptom onset. The upper row and lower rows, respectively, show the ischemic signs in the left middle cerebral territory at the basal ganglia level and the supraganglionic level. (a–e), respectively, represent non-contrast CT (a), source images of CT angiography (b), penumbra (green) and infarct (red) maps by CT perfusion superimposed on CTA source image (c), CBV maps (d), and MTT maps (e). doi:10.1371/journal.pone.0075615.g001 (Adapted from van Seeters T, Biessels GJ, Niesten JM, van der Schaaf IC, Dankbaar JW, Horsch AD, Mali WPTM, Kappelle LJ, van der Graaf Y, Velthuis BK, on behalf of the Dust Investigators (2013) Reliability of visual assessment of non-contrast CT, CT angiography source images and CT perfusion in patients with suspected ischemic stroke. PLoS ONE 8: e75615. doi: 10.1371/journal.pone.0075615. Copyright: © 2013 van Seeters et al.)
In addition to its application in detecting mismatch, perfusion imaging adds additional information to angiographic investigations in the evaluation of collateral circulation in symptomatic ICAS, due to the reason that downstream perfusion status in the case of intracranial arterial occlusion could be partly determined by the collateral compensating ability. Till now, there have been several methods using perfusion imaging alone, or by combining with angiographic imaging, to evaluate the extent of collateralization, some of which suggested beneficial effects of good collateral flow in acute ischemic stroke [18].
As compared with MR perfusion, CTP is more accessible and less time-consuming, which may be more feasible to be applied in the hyperacute phase of a symptomatic ICAS in clinical practice. However, the need for contrast agents may limit the use of routine CT and MR perfusion imaging in patients with contraindications and may also lead to contrast-induced complications. More recently, arterial spin-labeling perfusion-weighted MR imaging (ASL-PWI) has been used to measure cerebral blood flow and reveal perfusion status of individual cerebral arteries [22]. In some small studies, the ability of ASL-PWI has been tested to detect the ischemic penumbra so as to guide patient selection for intravenous thrombolytic therapy, which may be an alternative for patients with contraindications to the use of contrast agents. The application of ASL-PWI in ischemic stroke due to ICAS is further discussed below.
Novel Neuroimaging Methods to Evaluate ICAS
Besides of the routine methods as mentioned above, there are several novel imaging methods increasingly applied in the assessment of ICAS, which may facilitate more comprehensive assessment and more efficient risk stratification of such lesions. For instance, high-resolution magnetic resonance imaging (HR-MRI) is increasingly used to evaluate constituents and morphology of intracranial plaques and the vessel wall, which is promising in detecting vulnerable plaques and revealing mechanisms for ICAS-related stroke [31]; QMRA to quantify cerebral blood flow, which could simultaneously visualize vascular anatomy and evaluate the hemodynamic impact of ICAS lesions [32]; ASL-PWI, with no need to employ any contrast agents, to delineate perfusion status and detect mismatch for reperfusion therapies in acute stroke cases, to reveal perfusion territory and quantify cerebral blood flow of individual intracranial arteries, and to reveal and quantify collateral functions [22]; and four-dimensional CTA (4D CTA) or time-resolved CTA or multiphase CTA to provide angiographic and perfusion information based on CTP without adding additional single-phase CTA scan, which could improve the imaging quality as compared with routine single-phase CTA and could reveal flow directions and collateral status [33, 34]. Moreover, there are another two novel methods to evaluate the hemodynamic impact of symptomatic ICAS, paralleling to the evaluation of fractional flow in coronary artery disease, respectively based on two routine imaging methods TOF-MRA and CTA [35]. The MRA-based method is a newly developed index termed signal intensity ratio (SIR), representing the relative change of signal intensities (SI) across a symptomatic ICAS lesion on TOF-MRA images; while the CTA-based method utilizes the CFD technique to simulate blood flow, so as to depict the hemodynamic characteristics of symptomatic ICAS lesions [35]. All of these novel methods are of potential values in facilitating more comprehensive assessment and more accurate risk stratification of ICAS lesions, discussed in detailed below, which need further investigation and validation in the near future.
HR-MRI
HR-MRI has been used to delineate coronary and carotid plaques in enormous studies, both atherosclerotic and non-atherosclerotic. Morphology and components of atherosclerotic plaques in these arteries revealed by HR-MRI have been validated with histological findings in both animal models and humans, which reinforced the role of this technique to be the leading noninvasive in vivo imaging modality to characterize atherosclerotic plaques in these arteries [36]. Only in recent years has HR-MRI been applied in the characterization of intracranial plaques and the adjacent vessel wall (Fig. 10). In recent relevant studies, HR-MRI were usually performed under the field strength of 1.5 or 3.0 T, with higher field strength providing images of increased signal-to-noise ratio and higher quality, which could improve delineation of complex intracranial plaques [31].
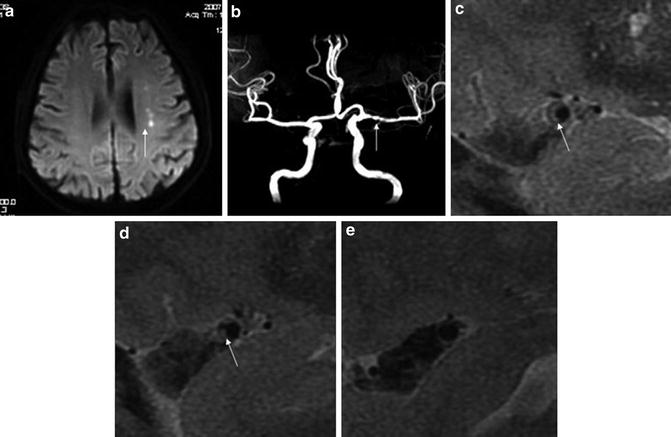
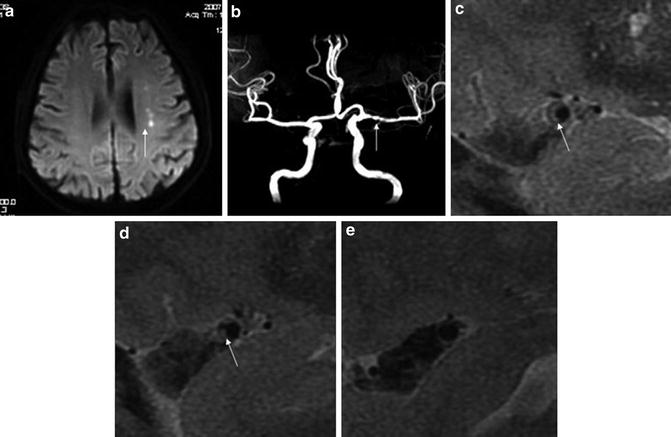
Fig. 10
High-resolution magnetic resonance imaging (HR-MRI) images (3.0 T) of a symptomatic ICAS lesion at the left middle cerebral artery (MCA). A 74-year-old male had multiple acute infarcts (arrow, a) in the distribution of stenotic left MCA (arrow, b). On consecutive sagittal T2-weighted image slices, a plaque with a band of high signal (long arrows, c and d) is seen in the stenotic segment. The reference segment (a relatively normal segment) is also shown (e) (Adapted from Xu WH, Li ML, Gao S, Ni J, Zhou LX, Yao M, Peng B, Feng F, Jin ZY, Cui LY (2010) In vivo high-resolution MR imaging of symptomatic and asymptomatic middle cerebral artery atherosclerotic stenosis. Atherosclerosis 212: 507–511. doi: 10.1016/j.atherosclerosis.2010.06.035; with permission)
In several studies using HR-MRI to depict coronary and carotid plaques, it has been demonstrated feasible and reliable to quantify the plaque size, to characterize the vessel wall, and to define the plaque vulnerability in these arteries, while its application in intracranial plaques has not been fully appreciated [31, 36]. Based on limited data, intracranial arterial stenosis due to atherosclerosis could be differentiated from that of other causes by using HR-MRI, and intraplaque hemorrhage (Fig. 11), lipid core, and fibrous cap in ICAS could also be detected. But components of intracranial plaques as defined by HR-MRI have not been widely validated with histopathological findings [31]. According to a small postmortem, in vitro study, HR-MRI at a high field strength of 7 T had substantial accuracy in detecting intracranial plaque and its components [37].
