Fig. 1
Discordant mIBG/FDG PET imaging. Patient with metastatic phaeochromocytoma, referred for mIBG therapy. a 131I mIBG anterior whole body imaging shows focal abnormal uptake in the superior thoracic spine and left scapula (arrows). b Corresponding, contemporaneous 18F FDG PET MIP image in same patient demonstrating multiple FDG avid foci of abnormal uptake in addition to the known, mIBG positive thoracic spine and scapular metastases. In this case, the discordant images suggest tumour de differentiation such that mIBG uptake underestimates the tumour burden. 131 I mIBG therapy was inappropriate. The patient proceeded to surgical stabilisation of the thoracic spine metastasis followed by combination chemotherapy
Table 1
Eligibility criteria for 131I mIBG therapy
Essential requirements | Contraindications | Precautions |
---|---|---|
mIBG uptake at all tumour sites | Pregnancy | Unfit for isolation |
Haematology Hb ≥9 g/dl WBC ≥3.0 x 109/l Platelets ≥100 x 109/l | Lactation | Unmanageable incontinence |
Acute spinal cord compression | ||
Haemodynamic/neurological instability | ||
Renal function GFR ≥30 ml/min |
4 Patient Preparation
Several drug classes, summarised in Table 2, interfere with mIBG uptake and should be discontinued for approximately three half-lives prior to planned treatment (Bombardieri et al. 2010). Adequate time should be allowed to ensure that patients are haemodynamically and neurologically stable following medication changes required prior to 131I mIBG therapy. Thyroid blockade using potassium iodide/iodate is essential to prevent thyroidal uptake of free 131I iodide. Blockade should commence 24 h prior to 131I mIBG administration and be continued for 5–10 days. Prophylactic anti-emetics such as the 5 HT3 antagonists ondansetron or granisetron are strongly recommended, commencing 1–2 h before treatment and continued for 3–5 days post therapy. Prophylactic steroids may also be appropriate to mitigate any risk of radiation oedema in patients who have bulky mediastinal or hepatic tumours, as illustrated in Fig. 2. In vitro evidence indicates that steroid co-administration may, in addition, improve the therapeutic ratio in patients undergoing 131I mIBG therapy (Bayer et al. 2009).
Table 2
Common drug classes known, or presumed to interfere with NA transporter-mediated mIBG uptake [Adapted from (Bombardieri et al. 2010)]
Drug class | Common drug within class |
---|---|
Alpha adrenergic blockers | bretylium, guanethidine |
Combined alpha and beta adrenergic blocker | labetalol |
Calcium channel blockers | diltiazem, nifedipine, verapamil |
Sympathomimetics: Inotropes Beta 2 agonists Vasoconstrictors/topical/systemic decongestants | dobutamine, dopamine ipratropium, salbutamol, terbutaline ephedrine, noradrenaline, phenylephrine, pseudoephedrine |
Neuroleptics | amisulpride, benperidol, chlorpromazine, clozapine, flupentixol, fluphenazine, haloperidol, levomepromazine, olanzapine, pericyazine, perphenazine, pimozide, pipotiazine, prochlorperazine, promazine, quetiapine risperidone, sertindole, sulpiride, thioridazine, trifluoperazine, zotepine, zuclopenthixol |
Antihistamines | cetirizine, chlorpheniramine, loratidine |
Antidepressants: Tricyclic Tetracyclic Serotonin reuptake inhibitors Noradrenaline reuptake inhibitor | amitriptyline, clomipramine, dothiepin, doxepin, imipramine, lofepramine, nortriptyline, trimipramine amoxapine mianserin, mirtazapine, trazodone fluoxetine, paroxetine, venlafaxine atomoxetine, reboxetin, |
CNS stimulants | amphetamines, cocaine, methylphenidate, modafinil |
Adult patients should be continent and self-caring in order to minimise radiation exposure to nursing staff. It may be appropriate to catheterise very young children who can then be managed safely in isolation with appropriate planning and parental/carer support. Compliance is improved by complementing verbal advice with written information and by providing audiovisual equipment, computer games and toys. It is helpful if patients and their carers have the opportunity to visit the isolation facility and familiarise themselves with the environment prior to elective admission. Written informed consent should be obtained prior to treatment.
5 Treatment Administration/Delivery
Depending upon local statutory requirements, 131I mIBG treatment is usually administered in a dedicated facility with appropriate lead shielding and en suite facilities. High activity 131I mIBG is supplied frozen to prevent radiolysis and must be thawed slowly in a 37 °C water bath immediately prior to administration. Routine quality assurance procedures include verification of the activity to be administered using an isotope calibrator and confirmation of radiochemical purity, the acceptable limit for free 131I iodide being <7 %. The thawed 131I mIBG is usually diluted in 0.9 % saline prior to intravenous administration via a peripheral cannula or long line using a shielded infusion pump over 60–120 min. Semi-automated vital signs monitoring is recommended during infusion in adult patients, but may prove counterproductive in small children. Changing circulating catecholamine levels, probably resulting from displacement of intracellular catecholamine stores, can cause blood pressure and pulse rate fluctuations during infusion. Short acting phentolamine and esmolol should be available for emergency use during treatment administration. In practice, however, it is usually safer to discontinue temporarily or reduce the 131I mIBG infusion rate until haemodynamic stability is restored than to administer additional alpha or beta blockers.
Following treatment, repeated whole body dose rate measurements are obtained for dosimetry purposes and to determine the date for safe discharge from isolation in accordance with local statutory requirements. Post therapy imaging is always performed prior to discharge to confirm tumour targeting and disease distribution. The high photon flux and opportunity for delayed imaging following background washout allows the tumour burden to be defined more reliably than by diagnostic scintigraphy (Hickeson et al. 2004) and facilitates retrospective dosimetric analysis.
6 Administered Activity
In the 1980s, 131I mIBG therapy was prescribed cautiously, using both low activities per treatment fraction and low cumulative activities. The primary clinical objective was symptom palliation and treatment safety was of paramount importance. Administered activities, in general, delivered whole body radiation absorbed doses well below the 1 Gy expected to result in myelosuppression and were below dose thresholds likely to cause non-haematological toxicity.
With experience, however, many centres developed more intensive treatment protocols, delivering repeated, high activity therapy at frequent treatment intervals. 131I mIBG uptake is usually optimal during the first treatment cycle and may decrease following subsequent administrations as the result of favourable treatment response, reduced efficiency of the NA transporter or a combination of both. In the light of this experience, most clinicians now prescribe a relatively high activity for the first treatment cycle.
Published experience consistently demonstrates improved objective response in terms of tumour regression, reduced tumour markers and symptom benefit following activity escalation. In the absence of randomised controlled trials, it is unclear whether this advantage reflects dose intensity (activity per treatment cycle) or treatment duration (cumulative activity). Mathematical modelling supports high activity therapy for microscopic, rapidly dividing tumours, assuming a reasonably uniform dose distribution delivered by relatively short range, particle emitting radionuclides (O’Donoghue et al. 1995). The hypothesis that early eradication of radiosensitive tumour might mitigate later emergence of less well-differentiated clonogenic lines formed the basis for trials of myeloablative, single cycle, high activity 131I mIBG in NBL with bone marrow or stem cell rescue (Goldberg et al. 1998).
An alternative approach recommends repeated, cycles of lower activity treatment administered repeatedly over months or years for sustained benefit. Lower activity 131I mIBG therapy might offer a therapeutic advantage in slower growing, macroscopic tumours (O’Donoghue et al. 2000). Advocates of this approach report similar efficacy, durable response and significantly lower myelotoxicity than high activity regimens (Buscombe et al. 2005; Navalkissoor et al. 2010; Nguyen et al. 2004), but no randomised controlled comparison has been undertaken.
Several treatment regimens are used in routine practice. The majority of centres administer fixed 131I mIBG activities of 7.4–11.2 GBq (100–300 mCi) at 3–6 monthly intervals. There is no consensus regarding the optimal number of treatment cycles or total treatment duration, which will usually be determined on an individual patient basis according to realistic treatment objectives. A more rational approach would be to undertake prospective dosimetry and standardise treatment prescription according to predicted whole body absorbed dose. This is discussed in more detail later.
7 Follow-up/Post Treatment Monitoring
The main toxicity of 131I mIBG therapy is temporary myelosuppression, manifested by thrombocytopaenia and neutropaenia. The nadir is delayed by comparison with chemotherapy and typically occurs 6 weeks post treatment, with slow recovery over the next 6–8 weeks. The rate and completeness of recovery reflects underlying bone marrow reserve. Haematological toxicity depends upon red marrow dose, which is in turn, governed by administered activity, 131I mIBG clearance and disease distribution. Toxicity is significantly higher in patients who have bone marrow involvement at the time of treatment and in those who have been heavily pre-treated with combination chemotherapy or external beam radiotherapy. Myelosuppression is cumulative in patients receiving repeated courses of 131I mIBG therapy. Despite appropriate thyroid blockade, hypothyroidism is reported in up to 25 % of patients treated with 131I mIBG (van santen et al. 2005) and annual thyroid function testing for life is advisable.
Late adverse effects such as myelodysplasia and second malignancy have been reported following 131I mIBG therapy but this risk has not been assessed systematically. Limited data quality combined with relatively short follow-up in a typical, heavily pre-treated NET patient population makes it difficult to distinguish between the effects of previous cancer therapy and radioisotope treatment. As outcomes improve, it will be essential to collect and share survivorship information relating to late effects of treatment.
8 Treatment Timing
131I mIBG is conventionally prescribed to treat refractory, advanced disease. Radiopharmaceutical uptake and, therefore, treatment efficacy may be compromised by previous interventions such as external beam radiotherapy or chemotherapy which reduce the efficiency of the NA transporter.
Experience using mIBG in a neo adjuvant setting is relatively limited. Anecdotal reports note the value of pre-operative mIBG therapy in achieving sufficient tumour shrinkage and reduced vascularity to facilitate R0 surgical resection of solitary tumours that were otherwise deemed inoperable. The feasibility and efficacy of 131I mIBG therapy as first line treatment for NBL was pioneered in the Netherlands (Hoefnagel et al. 1991). Exploiting the high metabolic activity observed in untreated tumours, the objectives of this approach were to reduce tumour volume whilst minimising both toxicity and induction of multidrug resistance. Combination chemotherapy was then reserved to treat minimal residual disease following surgical resection of the primary tumour. In an initial study, 31 children with inoperable Stage III/IV NBL received two or more cycles of 131I mIBG, followed by surgery and vincristine/etoposide/carboplatin/ifosfamide chemotherapy. 70 % ORR was reported following treatment induction allowing 19 of 27 evaluable children proceeded to R0/R1 primary tumour surgical resection (Hoefnagel et al. 1994). Subsequent cohort expansion to 49 children showed 38 % 5-year survival (Hoefnagel 1999). Treatment was well tolerated and haematological toxicity proved significantly lower than would have been expected in children who had bone marrow infiltration at the time of 131I mIBG treatment. Despite favourable outcomes, the significant logistic impediments to 1st line 131I mIBG therapy in the paediatric population have prevented wider acceptance and adoption of this approach.
9 Clinical Efficacy
The initial, investigator-led development of 131I mIBG treatment met scientific standards in place in the 1980s but would not match current criteria for evidence-based practice. The lack of randomised controlled trials does not, however, diminish the value of extensive experience reported in early peer reviewed publications which consistently supports the efficacy and tolerability of 131I mIBG therapy.
Although the treatment aim is cure, disease arrest, symptom palliation and control of hypersecretory function are more realistic goals for the majority of adult patients referred for 131I mIBG therapy.
10 Phaeochromocytoma and Paraganglioma
131I mIBG remains the best treatment option for patients with inoperable or metastatic phaeochromocytoma or paraganglioma. Pooled data from published series indicate objective response rates of 30–47 % with symptom palliation ranging from 75 to 90 % and biochemical response 45–74 % (Gonias et al. 2009; Loh et al. 1997; Safford et al. 2003). Results appear similar to those reported following palliative chemotherapy using cyclophosphamide, vincristine and dacarbazine (Huang et al. 2008; Szalat et al. 2011) although no direct randomised controlled comparisons have been undertaken. Combination chemotherapy is associated with higher toxicity than 131I mIBG therapy and has not been shown to prolong survival (Szalat et al. 2011).
A retrospective analysis of 116 patients treated using mean administered and cumulative 131I mIBG activities of 5.8 GBq and 18.1 ± 12.9 GBq, respectively, reported symptom palliation in 76 %, biochemical improvement in 45 % and tumour response in 30 % (Loh et al. 1997). Subsequent series have focussed on activity escalation. A retrospective series of 33 patients who received a mean single administration of 14.4 GBq reported 38 % radiological partial response (Safford et al. 2003). Prolonged median survival was observed among patients who achieved a biochemical or symptom response and in a subset of 15 patients who received activities >18.5 GBq. A further series of 28 patients showed similar objective response rates (30 %) between two patient groups treated using 5.55 and 9.25–1295 GBq 131I mIBG but noted a significantly shorter time to response in the higher activity group (7 months vs. 19 months respectively) (Castellani et al. 2010). Developing the concept of high activity therapy further, a Phase II study of high activity treatment (12 mCi/kg) in 50 patients with metastatic phaeochromocytoma/paraganglioma demonstrated ORR 22 % in 49/50 evaluable patients and 5-year OS 64 % (Gonias et al. 2009), compared with OS 44 % in an historical control population (Sarnaan et al. 1988). Grade 3/4 haematological toxicity was high (87 %) and 8 % of patients required autologous peripheral blood stem cell rescue. With haematological support, new patterns of toxicity were observed in late responding tissues including the lung, comprising pneumonia, acute respiratory distress syndrome and bronchiolitis obliterans. This is consistent with previous estimates of late responding normal tissue tolerance following targeted radionuclide therapy (O’Donoghue and Wheldon 1988).
Based upon this experience, it would be reasonable to conclude that activity escalation beyond 18.5 GBq (500 mCi) does not improve response rates but contributes to myelotoxcity.
11 Gastroenteric Neuroendocrine Tumours
Although approximately 70 % of GE NETs are mIBG positive (Khan et al. 2008), uptake intensity and predicted tumour absorbed dose are often too low for high activity 131I mIBG treatment to be a realistic option. Poor mIBG uptake is typically associated with less well-differentiated tumours which may be better targeted using somatostatin receptor analogues (SSR). The emergence of peptide receptor targeted therapy (PRRT) and, more recently, mammalian target of rapamycin (mTOR) and tyrosine kinase inhibitors (TKI) has reduced demand for 131I mIBG for GE NETs. A pragmatic approach would be to undertake semi-quantitative diagnostic 123I mIBG and 111In SSR imaging in all patients who might be eligible for targeted therapy to ensure optimal radiopharmaceutical selection on an individual patient basis. 131I mIBG is less nephrotoxic than PRRT and remains the treatment of choice for patients with pre-existing renal impairment (Grunwald and Ezziddin 2010).
Published experience using 131I mIBG therapy in patients to treat metastatic GE NETs indicates overall responses in the range 40–60 % (Castellani et al. 1991; Hoefnagel and Lewington 2004; Mukherjee et al. 2001) although complete radiological response is rare. Treatment delivers symptom palliation, improved QoL and reduced somatostatin analogue therapy requirements. A retrospective review of 98 patients with refractory, metastatic GE NET who were uniformly treated with 131I mIBG demonstrated a 38-month increase in median survival among patients who reported symptom palliation and improvement in actuarial survival of 22 % at 5 years (Safford et al. 2004). A trend towards prolonged survival (median 4.7 years) following high activity treatment was reported compared with 1.9 years after low activity therapy, supporting the initial prescription of activities of 15 GBq (Safford et al. 2004). Biochemical response (reduced urinary 5HIAA) and tumour regression by size criteria were observed in 15 % of patients but did not correlate with survival gain. Although it has long been assumed that symptom benefit reflects reduced tumour metabolic function, this contention is not supported by the results obtained. Reported symptom benefit appears consistently higher than can be explained by decreased hormone secretion or tumour mass alone.
12 Neuroblastoma
Despite advances in multimodality treatment, 5-year disease-free survival for children with high risk neuroblastoma (NBL) remains poor at 30–45 % (Ladenstein et al. 1998; Matthay et al. 1999). The initial observation that over 90 % of NBLs concentrate mIBG (Leung et al. 1997) prompted the introduction of palliative 131I mIBG therapy for children with end stage relapsed or primary refractory disease. Even in this unfavourable population, early pooled experience from nine individual centres indicated objective response or disease stabilisation in 49/75 of patients (59 %) (Beierwaltes 1987; Bestagno et al. 1987; Cottino et al. 1987; Fischer et al. 1987; Hartmann et al. 1987; Sanguinetti 1987; Treuner et al. 1987; Troncone et al. 1987; Voûte et al. 1987). This experience led to new approaches designed to improve 131I mIBG treatment response in NBL. These included activity escalation, incorporating 131I mIBG treatment within myeloablative regimens and, more recently, multimodality treatment combining mIBG with chemotherapy.
12.1 Activity Escalation
A preliminary Phase I dose escalation study of 25 children with relapsed or refractory NBL delivered whole body radiation doses of 1–2.5 Gy using prospective dosimetry. 33 % PR and 39 % SD were reported. Haematological toxicity correlated strongly with WB dose but no dose response in terms of efficacy was shown (Lashford et al. 1992). A subsequent Phase I activity ranging study in 30 patients demonstrated OR 37 % following administration of 111–666 MBq/kg (93–18 mCi/kg), with superior responses in children who received ≥444 MBq/kg (12 mCi/kg) (Matthay et al. 1998). A later Phase II study in 164 children confirmed 37 % overall response in patients treated at an activity of 666 MBq/kg with peripheral blood stem cell support compared with 25 % response in a smaller cohort treated at 444 MBq/kg without stem cell back up (Matthay et al. 2007). Repeated mIBG infusions delivered at median 3-month intervals demonstrated superior responses in patients who received higher cumulative activities but haematological toxicity proved dose limiting (Howard et al. 2005). A more intensive tandem approach administering two treatment cycles, 2 weeks apart with PBSC rescue proved feasible but did not improve PFS (Matthay et al. 2009).
12.2 Myeloablative Therapy
Myeloablative therapy is now considered standard of care in children with high risk NBL. Several pilot studies have explored the incorporation of 131I mIBG within myeloablative regimens in this group. A small Phase I trial demonstrated ORR 27 % with median EFS of 18 months and median OS 48.1 m in a cohort of 22 children with primary refractory NBL. Toxicity was similar to that reported for conventional myeloablative treatment although dose limiting hepatotoxicity and veno-occlusive disease were higher than expected in children with pre-existing renal impairment (Matthay et al. 2006).
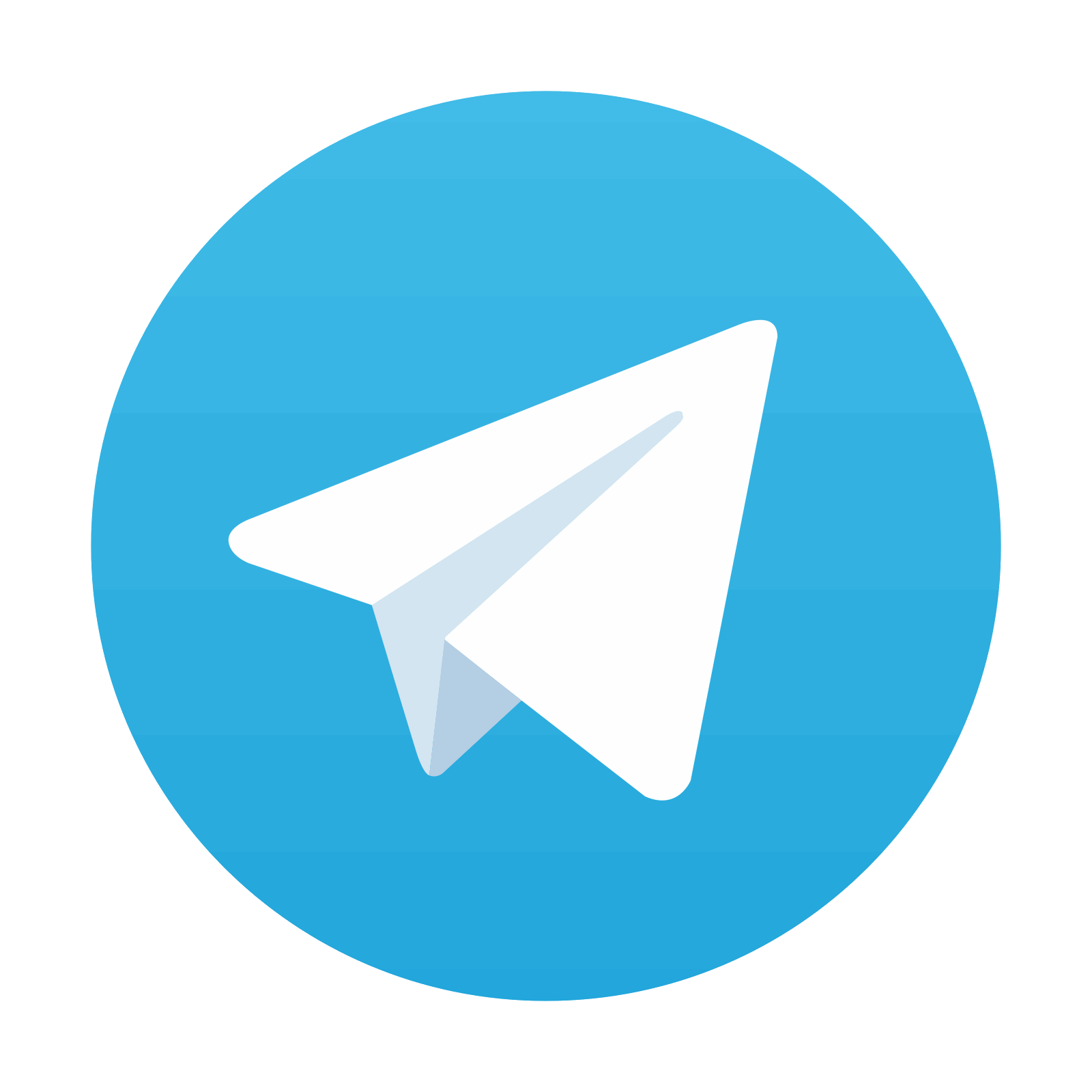
Stay updated, free articles. Join our Telegram channel

Full access? Get Clinical Tree
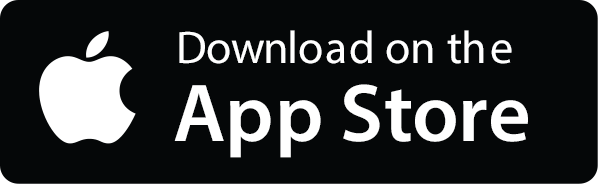
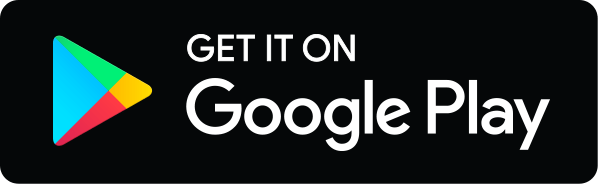