Fig. 16.1
Nonenhanced CT scan of a patient with BSPDC demonstrating bilateral, nearly symmetric calcifications of the basal ganglia and posterior thalamus. Less intense (and more punctuated) calcifications are seen in the dentate nucleus. An additional finding includes a left-sided posterior cerebral artery stroke, which is unrelated to BSPDC
In BSPDC, the calcifications on CT show a fairly symmetric distribution [29]. The pallidum is most frequently affected [38]. A combination of calcium deposits in the striatum, dentate nucleus, thalamus, and white matter is common, whereas cortical calcifications appear to be less frequent [29, 40]. There are conflicting findings as to whether the extent and the localization of calcifications correlate with the neurological impairment [5, 25, 29, 41, 42]. In a study including CT scans of 31 BSPDC patients, the mean amount of calcifications in the basal ganglia was not different between symptomatic and asymptomatic subjects, whereas the total amount of intracerebral deposits were higher in the group of subjects with symptoms [29]. In one patient, subsequent CT scans revealed an increasing volume of calcifications corresponding to the progression of neurological impairment [29]. At a later point in time, the degree of calcifications decreased, presumably because of the onset of secondary brain atrophy. Circumscribed temporal and frontal cortical atrophy is common at a later disease stage [43]. Taxer et al. [41] found that the symptomatology may be independent of the localization of intracerebral calcifications, whereas the amount of calcium deposits corresponds to the severity of symptoms particularly with respect to extrapyramidal symptoms and dementia. In another study, patients with a parkinsonian phenotype and calcifications in the basal ganglia were clinically indistinguishable from Parkinson’s disease patients without calcifications [42]. In this study, however, also patients with unilateral or asymmetric calcifications were included, and thus, it remains questionable whether they really represent classical BSPDC.
Magnetic Resonance Imaging
Magnetic resonance imaging (MRI) has a lower sensitivity and lower specificity than CT in diagnosing intracerebral calcifications [44–48]. In T1-weighted images, the signal contrast caused by calcifications is often inconsistent. In general, calcium deposits reduce the T1 relaxation time by a surface relaxation mechanism [49]. With an increasing amount of deposits, T1 intensity is supposed to increase initially and to then decrease subsequently [49]. In the majority of cases, the T1 signal is hyperintense, but isointense or hypointense signal behavior can also be observed within the same subject or between different subjects [45, 50, 51]. The changes in T1 signal may be related to the disease stage, differences in calcium metabolism, and the volume of deposits [52]. In contrast, T2 signal changes and calcified brain regions upon CT show a varying distribution. Confluent hyperintense T2 lesions of the white matter in the centrum semiovale are common, and they do not correspond to the localization of calcifications [23, 45, 53]. It is speculated that the T2 hyperintensities represent slowly progressive metabolic and inflammatory processes that result in and correlate with progressive neurological deficits [45].
Transcranial Sonography
Transcranial sonography (TCS) has become a useful diagnostic tool in the differential diagnosis of movement disorders . TCS may also be helpful in establishing an early diagnosis of Parkinson’s disease [54]. Advantages of TCS are its widespread availability, cost effectiveness, noninvasiveness, and the avoidance of exposure to x-rays. The method allows unlimited repeated measures and is even applicable in agitated patients. An experienced investigator is able to perform a TCS scan within 5–10 min.
A striking finding in Parkinson’s disease is an enlarged area of hyperechogenicity projecting to the anatomical site of the midbrain substantia nigra [55]. Abnormal hyperechogenic areas within the caudate and lenticular nucleus have been reported in atypical parkinsonian syndromes [56], Huntington’s disease [57], multiple sclerosis [58], primary dystonia [59, 60], spinocerebellar ataxia type 3 [61], and Wilson’s disease [62, 63]. Several factors have been discussed as the possible underlying pathoanatomical correlate of basal ganglia hyperechogenicity: increased local iron [58, 64] and copper concentration [62], gliosis [58], and enlarged perivascular spaces or simply artifacts (see Chap. 6 for a more in-depth discussion). Moreover, diffuse hyperechogenicity of the basal ganglia and thalami is a frequent and nonpathologic finding in premature infants that is no longer detectable 1 month after delivery [65]. The most intense and striking hyperechogenicity in adulthood seems to derive from the accumulation of calcium compounds. Thus, BSPDC provides the unique opportunity to study basal ganglia alterations with a defined histopathology. Using TCS, small intracranial calcifications may occasionally be detected even prior to CT imaging [55, 62]. So far, striking basal ganglia hyperechogenicity in BSPDC has been reported in seven patients from different sites [21, 31, 66]. The hyperechogenic areas upon TCS correspond excellently to the symmetric distribution pattern as shown with either CT [21, 31] or MRI [66] and show almost the same level of intensity as the contralateral skull bone and the frequently calcified pineal gland (Fig. 16.2). Hyperechogenicity in extended calcified lesions as in BSPDC can be distinguished from the pattern observed in the context of a mixture of gliosis and iron and/or copper accumulation, which may be less striking. Apart from the high signal-to-noise ratio of the hyperechogenic signal, the highly symmetric distribution and the large conglomerates may be specific TCS features of BSPDC.
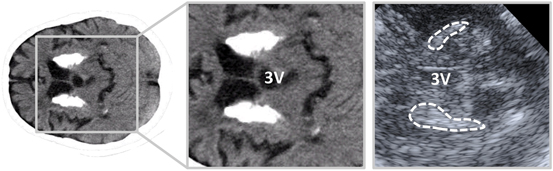
Fig. 16.2
Striatal calcifications (left image, magnified on the middle image) on a CT scan and on an anatomically corresponding TCS image (right image). Hyperechogenic striatal areas are highlighted in the TCS image. 3V third ventricle
Functional Radioligand Imaging Techniques
Functional radioligand imaging techniques, including single photon emission tomography (SPECT) and positron emission tomography (PET) , complement anatomical brain imaging methods such as CT and MRI. They are useful to study local brain perfusion, brain metabolism, and the nigrostriatal function in vivo.
In symptomatic BSPDC subjects, Tc-99m hexamethylpropylene amine oxime (HMPAO) SPECT revealed hypoperfusion in widespread subcortical and cortical regions, including the basal ganglia, thalamus, cerebellum as well as frontal, parietal, temporal, and insular cortical areas [21, 34, 51, 67, 68]. In two further case reports, hypoperfusion was restricted to the right-sided basal ganglia [69] and the thalami bilaterally [70] despite the fact that the calcifications were widely dispersed in these patients. In the latter study, Tc-99m ethylcysteinate was used instead of HMPAO to visualize the local cerebral blood flow. Using this agent, the reduction of cerebral perfusion was not per se associated with the amount of mineral deposits [70]. The clinical phenotype corresponds well to hypoperfused brain regions in BSPDC. Ones et al. [69], for example, reported a case of unilateral striatal hypoperfusion and contralateral parkinsonism . In one clinically asymptomatic subject, the regional brain perfusion was unremarkable [34].
In conformity with hypoperfusion in calcified regions and interconnected areas, 18F-fludeoxyglucose (FDG) PET revealed reductions of glucose metabolism in similar regions, including frontotemporal and cingulate cortices as well as the basal ganglia in symptomatic BSPDC, whereas asymptomatic subjects show a normal glucose brain metabolism [34, 71–73]. The hypometabolism corresponds to the clinical deficits and may result from a deafferentiation of striatal and cortical regions due to basal ganglia pathology. The multifaceted symptomatology may thus be explained by a disruption of motor, limbic, and associative cortico-subcortical neuronal circuits.
Using FP-CIT SPECT and 18F-DOPA PET, the dopaminergic nigrostriatal neurotransmission was shown to be impaired in several BSPDC patients presenting with parkinsonism . The reduced striatal tracer uptake was either asymmetric, and thus resembling idiopathic Parkinson disease [21], or symmetric [25, 68]. One patient with generalized chorea and neuropsychiatric symptoms but without features of Parkinson’s disease had a normal FP-CIT scan [21]. Normal presynaptic 18F-DOPA uptake has been observed in one asymptomatic patient and one patient with dementia and bradykinesia [44]. In two symptomatic subjects, PET displayed reduced striatal dopamine D1 and D2 receptor binding [25]. Thus, postsynaptic striatal dysfunction may be postulated in some affected individuals due to an interference of calcium deposits with postsynaptic dopamine receptors.
Conclusions and Future Directions
A combination of parkinsonism , cognitive deterioration, and cerebellar signs is a typical finding in BSPDC and should prompt clinicians to perform a cerebral CT scan, which remains the most effective screening tool. MRI is useful in detecting white matter pathology in uncalcified brain areas but its effectiveness in verifying intracerebral calcifications is limited. Preliminary data suggest that intracerebral calcifications show a distinct pattern of hyperechogenic areas upon TCS. In the hand of an experienced investigator, it may be justified to use TCS as a first screening tool. To date, however, a CT scan is still needed in order to verify or exclude intracerebral calcifications. Future developments in various neuroimaging modalities will provide the ability to detect changes early while improving our understanding of symptom-related changes in brain function.
References
1.
Kiroglu Y, Calli C, Karabulut N, Oncel C. Intracranial calcifications on CT. Diagn Interv Radiol. 2010;16(4):263–9.PubMed
2.
3.
4.
5.
6.
Stellamor K, Stellamor V. Roentgen diagnosis of Fahr’s disease. Rontgenblatter. 1983;36(6):194–6.PubMed
7.
8.
Cohen CR, Duchesneau PM, Weinstein MA. Calcification of the basal ganglia as visualized by computed tomography. Radiology. 1980;134(1):97–9.PubMed
9.
10.
Forstl H, Krumm B, Eden S, Kohlmeyer K. Neurological disorders in 166 patients with basal ganglia calcification: a statistical evaluation. J Neurol. 1992;239(1):36–8.PubMedCrossRef
< div class='tao-gold-member'>
Only gold members can continue reading. Log In or Register a > to continue
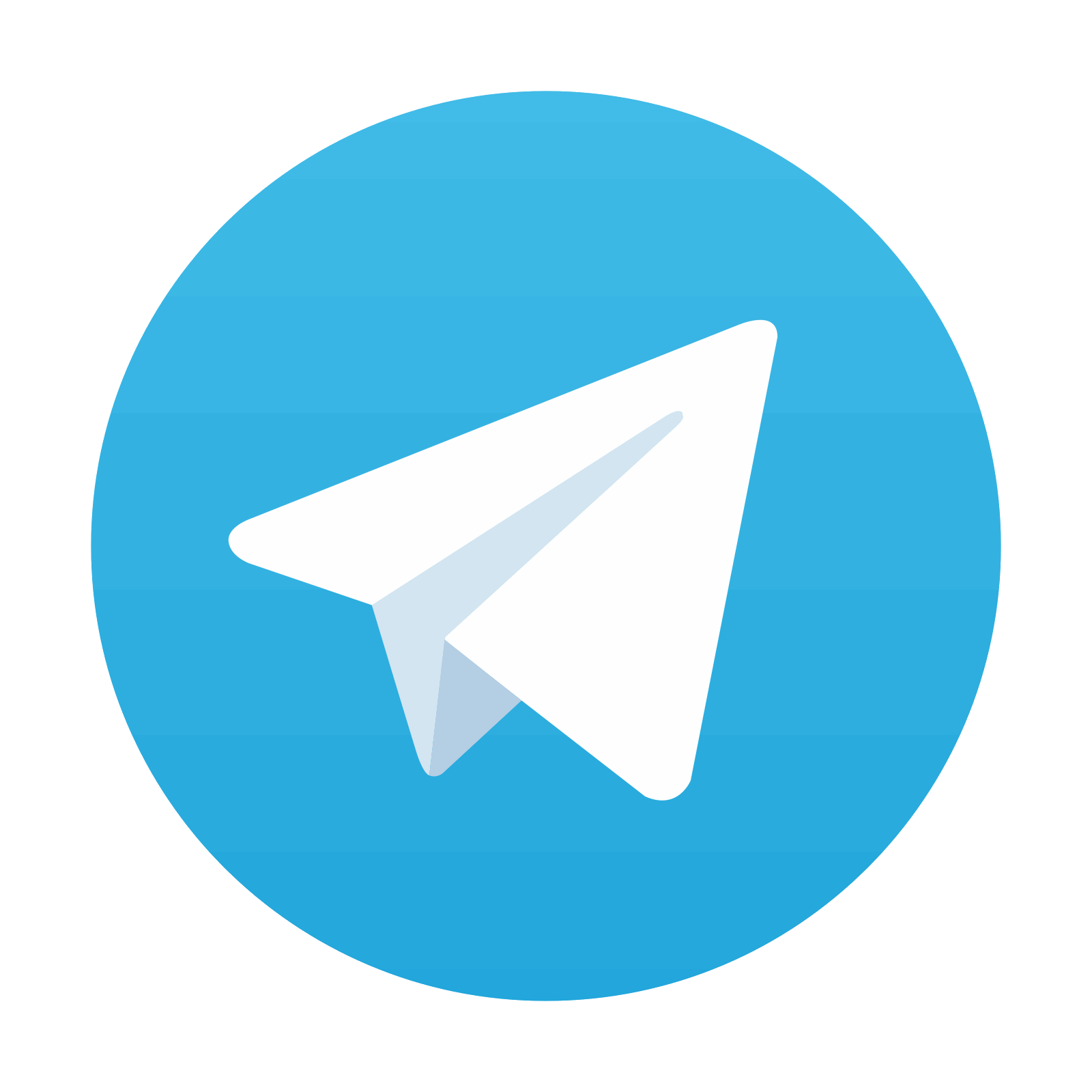
Stay updated, free articles. Join our Telegram channel

Full access? Get Clinical Tree
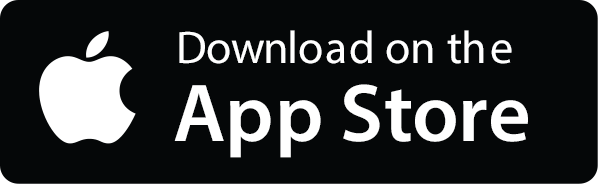
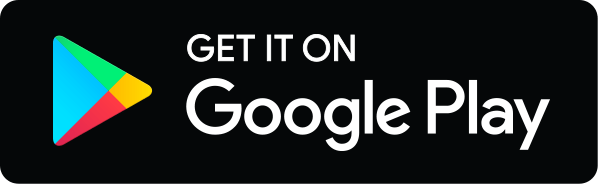