Fig. 1
Scheme illustrating the principle of pretargeting: a the biotinylated antibody binds to the antigen expressed at the surface of the tumor cell, b binding of avidin with high affinity to the biotin of the biotinylated antibody, c administration of radiolabeled biotin, which binds to the avidin of the Ab-biotin–avidin complex
3.3 Locoregional Application
In most studies, RIT of malignant gliomas was performed via local administration of the radioimmunoconjugate (either directly into the tumor or intracavitary into tumor cysts or the surgically created resection cavity) (Reardon et al. 2007; Zalutsky 2004, 2005). Clear advantages of this locoregional approach are bypass of the blood–brain barrier, high local radiation doses but low systemic toxicity and no major interference with potential human antibodies against mouse antigen (HAMA). For this form of application radiolabeled antbodies are administered via an Ommaya- or Rickham-Reservoir, which is surgically implanted during tumor resection or during a stereotactic procedure. The reservoir consists of a small elastic silicon reservoir, which is implanted subcutaneously with a connected catheter placed into the tumor, the tumor cyst or the resection cavity. All reagents may be injected through the reservoir directly into the resection cavity (Fig. 2).
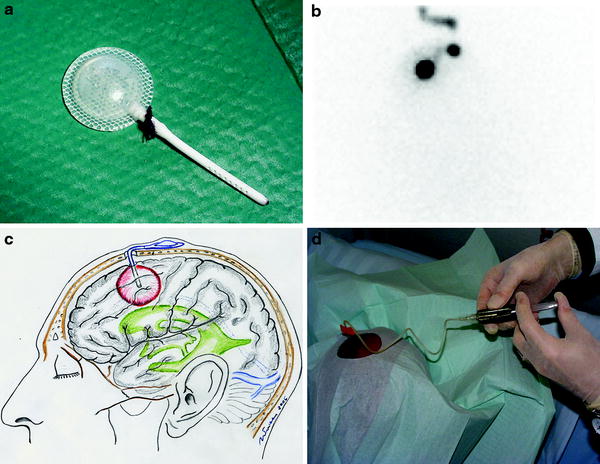
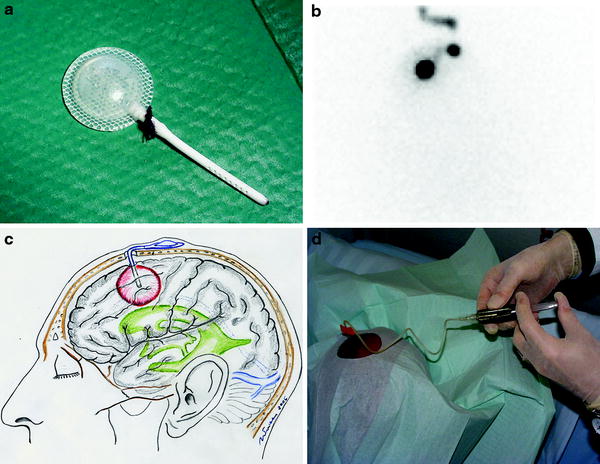
Fig. 2
Illustration of local administration of the radioimmunoconjugate: a Ommaya reservoir, b planar scintigraphy following administration of [99mTc]HSA by a butterfly needle into the subcutaneous reservoir and via the catheter into the resection cavity, c illustration of the subcutaneously implanted reservoir and d administration of the radioimmunoconjugate into the reservoir for intracavitary RIT
4 Radionuclides
The most frequently used radionuclide for RIT of malignant glioma is 131Iodine (Bigner et al. 1998; Cokgor et al. 2000; Reardon et al. 2006, 2008; Riva et al. 1999b). Advantages of the latter are the widespread experience using this radionuclide for other cancer treatments (especially for the treatment of differentiated thyroid cancer), its cost-effective production and the ease of synthesis. Disadvantages of 131I labeling are the considerable radiation exposure for the staff caused by the additional γ-particles. Furthermore, compared to other ß-emitters the maximal energy of 131Iodine is relatively low (0.61 MeV) resulting in a minor tissue penetration (medium/maximum tissue penetration range: 0.5/2.1 mm). Some studies were performed using 90Y-labeled mAb (Grana et al. 2002; Paganelli et al. 2001, 2006; Riva et al. 1999a). Physical (physical half life: 64.4 h) and radiobiological features of 90Yttrium make it also suitable for RIT. The high-energy ß-particles (maximum energy: 2.26 MeV) allow a deeper particle penetration compared to 131Iodine (medium/maximum tissue penetration range: 3.6/11 mm) resulting in higher radiation doses to the target area. Staff radiation exposure is much lower and, therefore, also allows treatment of high-maintainance patients. Another interesting radionuclide for RIT is the metallic 188Rhenium. Physical half-life (17 h) is much lower compared to 131Iodine (8d). Similar to 90Yttrium the maximum energy of ß-particles is high (2.12 MeV), resulting in a similar tissue penetration (medium/maximum tissue penetration range: 3.1/10 mm). Furthermore, additional γ-emission (155 keV) is useful for dosimetry. As a generator product 188Rhenium is characterized by good availability. Another promising radioisotope, which generated promising results in the treatment of other tumors (e.g., neuroendocrine tumors) but—so far—was not used for RIT of gliomas is Lutetium-177. Its lower γ-ray energy could simplify radiation protection, facilitate dosimetric,and distribution measurements and could lead to a lower radiation exposure of the normal brain with a lower risk of radiation necrosis.
Besides these ß-emitters, the Auger emitter 125Iodine was also used for RIT (Emrich et al. 2002; Quang et al. 2004). Since the penetration range of Auger electrons is only about 100 nm, incorporated auger emitters cause very high local radiation doses without harming the surrounding tissue. Therefore, a relevant radiation effect requires the internalization of the radioimmunoconjugate into the tumor cell. Over the past decade, new alpha emitters have also emerged and started to be considered for RIT. The specific properties of α-particles (shorter path range and high linear energy transfer) offer new opportunities for this therapeutic approach. However, so far RIT with α-emitters is predominantly used in preclinical settings due to some unsolved questions like optimization of radiolabeling and availability, improvement of administration modalities, and a better understanding of treatment effects.
5 Clinical Studies
5.1 Antibodies Against TN-C
5.1.1 Experiences at the University of Munich Using BC-4/BC-24
Since 1998, 51 patients (21 female, 30 male, mean age: 48 ± 13 years; 13 anaplastic astrocytomas WHO III, 38 glioblastomas WHO IV) have been treated with locoregional RIT. Surgery had been performed in 50/51 patients, percutaneous radiation therapy in 49/51 patients, and chemotherapy in 19/51 patients before initiation of RIT. 23/51 patients even had undergone re-operation before RIT. A total of 37 patients were treated in a phase I/II study using 131I-labeled TN-C-mAb (BC-4) and 14 patients within a dose escalation study using 188Re-labeled TN-C-mAb (BC-24). Radioimmunoconjugate was administered in all patients via an Ommaya reservoir, which was implanted during surgery (n = 19), re-surgery (n = 21), or during stereotactic procedure (n = 11). A total of 27 patients had only minor tumor manifestation (remaining contrast enhancing tumor in MRI less than 1 cm in diameter) and in 24 patients no macroscopic tumor rest was seen at study entry. Communication between the resection cavity and the liquor system was excluded in all patients by administration of 99mTc-labeled human serum albumin (HSA) via the reservoir followed by scintigraphy.
RIT was performed in several cycles (1–5 cycles) within 6–8 weeks each ([131I]TN-C-mAb (n = 37): 1,000–1,850 MBq/cycle; total: 3,010 ± 1,470 MBq; [188Re]TN-C-mAb (n = 14), dose escalation protocol: four patients: 370 MBq (1 cycle)/4 patients: 740 MBq (2 cycles à 370 MBq)/3 patients: 925 MBq (2 cycles à 370 MBq and 1 cycle à 185 MBq)/3 patients: 1,100 MBq (2 cycles à 370 MBq and 2 cycles à 185 MBq)).
8/51 patients are known to be still alive (18–136 months after initial diagnosis, mean: 73 ± 37 months): 5/8 long-term survivors without suspicion of recurrence and 3/8 patients under chemotherapy for tumor recurrence. 30/51 patients died (13–68 months following initial diagnosis; mean: 29 ± 13 months) and 13/51 patients were lost for follow up (12–145 months after initial surgery; mean: 47 ± 38 months). Two representative examples of MRI and FET PET follow-up are given in Figs. 3 and 4.
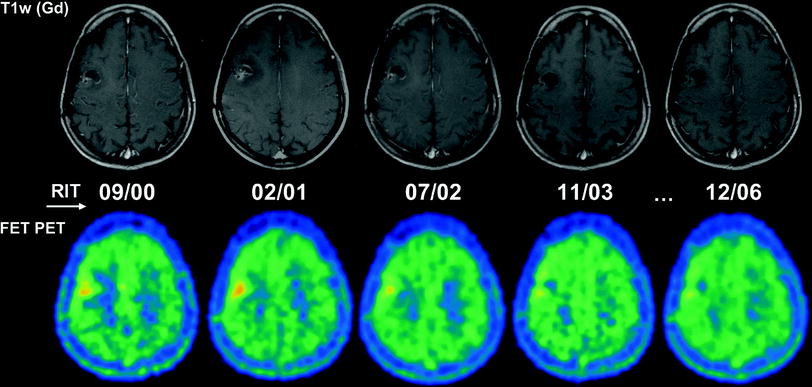
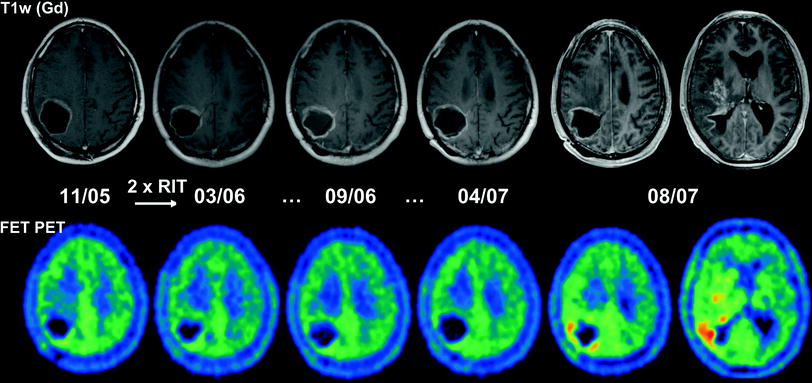
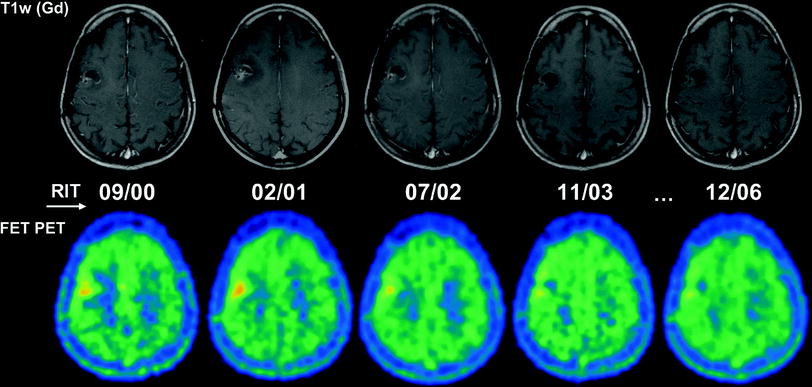
Fig. 3
Serial MRI and FET PET images of a 35-year-old woman with anaplastic astrocytoma following surgery (05/99) and two cycles of RIT using [131I]TN-C-mAb (cumulative activity: 2,460 MBq) with recurrence free follow-up. The patient is still alive under routine MRI controls 128 months following initial surgery
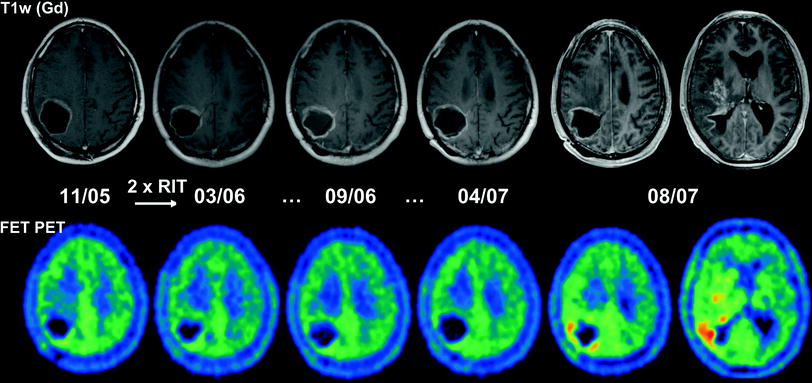
Fig. 4
Serial MRI and FET PET images of a 41-year-old man with glioblastoma following biopsy (05/05), percutaneous radiation therapy (05-06/05) and implantation of an Ommaya reservoir into a tumor cyst (11/05). Following RIT (two cycles: 715 MBq [188Re]TN-C-mAb) a recurrence free interval is followed by the growth of diffuse recurrence with wide spread tumor infiltrations far away from the tumor cyst 19 months after the end of RIT (patient was lost for follow-up in a palliative situation 44 months following initial surgery)
Median survival was 36 months for all patients (n = 51) with 68 months for the prognostically more favorable group of anaplastic astrocytomas (n = 13) and 29 months for glioblastomas (n = 38). For the [131I]TN-C-mAb (n = 37) group median survival was 32 months, for the [188Re]TN-C-mAb group (n = 14, dose escalation study, no dose limiting toxicity up to 1,100 MBq) median survival was 38 months.
Transient neurological symptoms were seen in 13 patients (seizures, headache, mild deterioration of neurological symptoms). Long-term adverse events were rare, consisting of four cases with mild radiation necrosis (in one case paired with deterioration of pre-existing motoric weekness of the right extremities; follow-up of this patient is illustrated in Fig. 5) and two cases with focal hair loss at the Ommaya reservoir. 35/51 patients developed human antibodies against mouse antibodies (HAMA), however, without any influence on further RIT cycles. Mild hematological toxicity was seen in some patients treated with high activities of [131I]TN-C-mAb. No severe hematological, renal, or hepatic toxicities were seen.
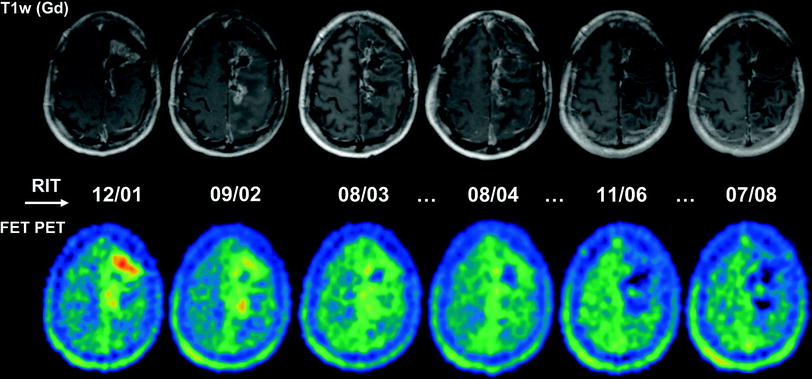
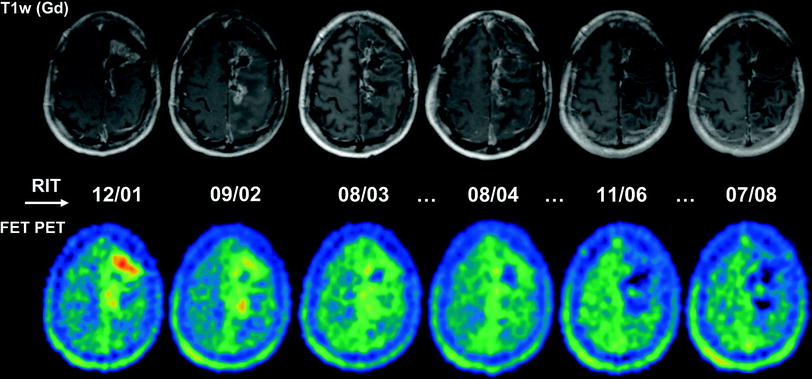
Fig. 5
Serial MRI and FET PET images of a 42-year-old man with anaplastic astrocytoma following surgery (10/98), percutaneous radiation therapy (11–12/98) and five cycles of RIT using [131I]TN-C-mAb (cumulative activity: 5,740 MBq, highest cumulative activity reached in the Munich patient group) with recurrence-free follow-up but mild radiation necrosis, which caused a long-term deterioration of pre-existing motoric weakness of the right extremities. The patient is still alive under routine MRI controls 135 months following initial surgery
5.1.2 Experiences at the Hospital of Cesena (Italy) Using BC-2/BC-4
In a pilot study ten patients with extensive recurrent glioblastomas were treated intratumorally with 131I-labeled murine TN-C-mAb (BC-2) (Riva et al. 1992) following dosimetric estimations (using planar scintigraphy and SPECT after locoregional administration of 37 MBq [131I]TN-C-mAb). To reach cumulative tumor doses of at least 150 Gy, four patients were treated three times, three patients two times and three patients with only one RIT cycle (activity per cycle: 110–1,145 MBq; mean: 550 MBq). No systemic dose limiting toxicity (DLT) was observed in this small patient group. Mild neurological symptoms were headache (n = 4) and one epileptic seizure. Posttherapeutic dosimetry revealed higher tumor doses compared to the pretherapeutic estimations, however, with still low doses for normal brain tissue and whole body. Short-term clinical outcome was progressive disease in four patients, stabilization in three patients, partial remission in two patients, and complete remission in one case with a disease-free period of 17 months. Following a phase I dose escalation trial (20 patients: 2 WHO II, 1 WHO III, and 17 GBM) a phase II study both using [131I]TN-C-mAb (BC-2 and BC-4) was conducted including 91 glioma patients (one WHO II, 16 WHO III, and 74 GBM) (Riva et al. 1999b). Patients showed extensive recurrent tumor in the dose escalation trial, in the phase II study 52 patients with only small residual or no tumor manifestations and 39 patients again with more extensive tumor manifestations were included.
For the dose escalation trial, patients were treated with increasing activities from 185 to 2,775 MBq. The maximal tolerable activity turned out to be 2,590 MBq per cycle with neuroimaging showing massive posttherapeutic edema above this limit but no significant changes below.
In consequence, the upper limit for the phase II study was set at this activity. Patients underwent up to ten RIT cycles (1,295–2,775 MBq each; mean activity: 2,035 MBq) with prolonging intervals. In total 24 patients received 3 cycles, 18 patients four cycles, ten patients 5 cycles and six patients 6 cycles (mean cumulative activity for 6 cycles: 10,345 MBq). The other patients got less than three or more than 6 cycles. Again no systemic adverse events were observed, neurological symptoms were headache (15 %) but no other symptoms. 17 patients developed surgery needing mass effect, which was rated as tumor progression. In all cases histopathological findings revealed extensive radiation necrosis with only minor (n = 14) or even lacking neoplastic cells. Similar to the Munich patient population 59 % of patients (especially following more than one RIT cycle) developed positive HAMAs however without any relevance for the pharmacokinetics of further treatments. Mean estimated doses for the resection cavity/the resection cavity wall were 300 Gy (ranging from 180 to 350 Gy) and 150 Gy (ranging from 80 to 190 Gy) per cycle, respectively. Cumulative doses for the thyroid were 4 Gy (caused by unbound 131-iodine) and only 0.5 Gy for the liver, kidneys, and bone marrow. Median survival was 46 months for anaplastic astrocytoma and 19 months for glioblastoma with better survival times for patients with only small or non-detectable tumor rest at begin of treatment. In this study, similar to the Munich experience, 13 long-term survivers were observed with survival times up to nine years.
In parallel, a phase I Study with 90Y-labeled TN-C-mAb (BC-4) was initiated, including 20 patients (two WHO III, 18 GBM) with bulky recurrent disease at study entry who were treated intratumorally/intracavitary with escalating activities (185–1,110 MBq, three patients per level) (Riva et al. 1999a). No local or systemic adverse events were documented up to an activity of 925 MBq, at 1,110 MBq 2/4 patients developed extensive perifocal edema inducing clinical symptoms with the necessity of steroid medication. Therefore, 925 MBq was supposed to be the maximal tolerable activity for [90Y]TN-C-mAb. Estimated doses for the whole cavity and the wall of the cavity were 86 cGy/MBq (ranging from 6 to 179 cGy/MBq) and 32 cGy/MBq (ranging from 2 to 70 cGy/MBq) per cycle, respectively, while again doses for liver, kidneys, and bone marrow were very low.
In the following phase II study 43 patients (eight WHO III, 35 GBM) were included with about half of patients showing more extended tumors and the other half of patients without macroscopic tumor manifestations before RIT (Riva et al. 2000). At average 740 MBq were administered per cycle for a total of 3–5 cycles (maximum cumulative activity: 3,145 MBq). Doses for the cavity and the cavity wall were estimated to 600 and 280 Gy, respectively. Median survival for patients with anaplastic gliomas WHO III was 90 months, for GBM patients 20 months (31 months in patients with minor tumor load at study entry). Severe adverse events were not described.
5.1.3 Experiences with BC-2/BC-4 and Pretargeting at the hospital of Milano (Italy)
RIT and tumor pretargeting with the avidin–biotin system described above, either used for systemic or for locoregional administration was used by the Milan group. In a phase I/II study the toxicity and therapeutic efficacy of this approach was evaluated in 48 eligible patients (17 WHO III, 31 GBM) with documented residual or recurrent disease after conventional treatment. The three step RIT was performed by intravenous injection of the three compounds: biotinylated TN-C-mAb (BC-4), followed 36 h later by avidin/streptavidin, and again 18–24 h later by [90Y]biotin) (Paganelli et al. 1999). 111In-labeled DOTA-biotin was added for intratherapeutic dosimetry. The injected activities ranged from 2.22 to 2.97 GBq/m²/cycle, with 24 patients getting one, 18 patients getting two and six patients getting three RIT cycles at an interval of 2–7 months. For one cycle tumor doses were calculated to be 15.2 ± 8.7 cGy/37 MBq. As expected systemic toxicity was higher compared to locoregional application (bone marrow: 0.8 ± 0.5 cGy/37 MBq, kidneys: 2.7 ± 1.6 cGy/37 MBq, liver: 1.5 ± 1.0 cGy/37 MBq, brain: 0.6 ± 0.3 cGy/37 MBq). Hematologic toxicity was observed in about one half of the patients with more than 30 % severe toxicities at activities over 2.96 GBq/m². Therefore, the maximum tolerated dose was determined at the level of 2.97 GBq/m². Unfavorable allergic reactions were seen following streptavidin (especially folllowing several cycles), less frequently also on TN-C-mAb and on avidin. Two months following treatment 25 % of patients showed disease remission, 52 % had a stable course of disease and 23 % had progressive disease. Mean duration of response was 6 months with a median survival of 16 months for glioblastoma and 57 months for anaplastic astrocytoma.
These results led to a further study performing RIT in an adjuvant setting. 37 patients (17 WHO III, 20 GBM) following surgery and radiation therapy were included in a controlled open non-randomized study (Grana et al. 2002). At study entry all of them were disease free according to neuroradiological examinations. A total of 19 patients (eight GBM, 11 WHO III) underwent RIT and were treated with 2.2 GBq/m² according to the three-step pretargeting protocol, while the remaining 18 patients (controls: 12 GBM, six WHO III) were not treated. Median disease-free survival for treated GBM patients was 28 months (ranging from 9 to 59 months) with a median overall survival of 33.5 Monaten. All 12 GBM controls died following a median survival of only eight months. For treated WHO III gliomas median progression free survival was 56 months (15–60 months) with statistical significant differences between the treated and untreated groups. Mild hematologic toxicity was seen in 42 % of the RIT patients. Further on, many of the treated patients developed HAMAs against streptavidin, avidin, or the monoclonal antibody itself.
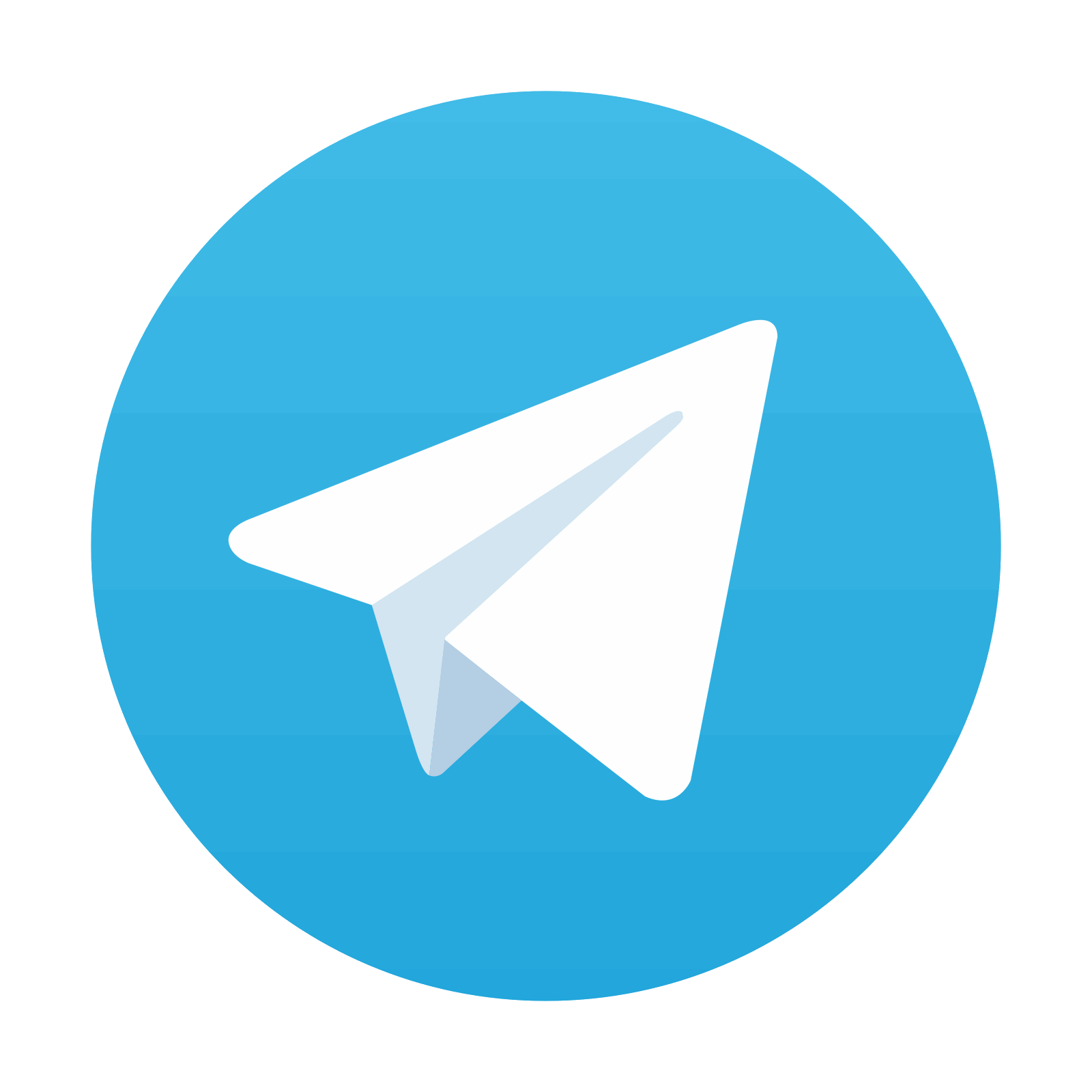
Stay updated, free articles. Join our Telegram channel

Full access? Get Clinical Tree
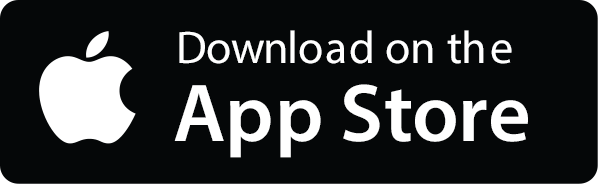
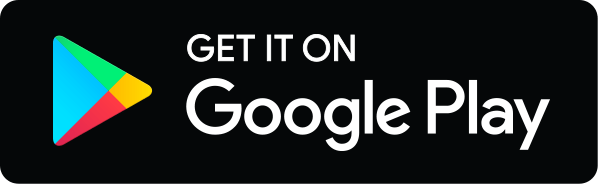