Antibody
Type
Trials
3F8 (Cheung et al. 2012b)
Murine IgG3
Phase I, II
14G2a (Murray et al. 1994)
Murine Ig
Phase I
Ch14.18 (Yu et al. 2010)
Chimeric IgG1
Phase I, III
Hu14.18K322A (Navid et al. 2010)
Humanized IgG1
Phase I
Hu14.18-IL2 (Shusterman et al. 2010)
Immunocytokine
Phase I, II
Hu3F8 (Cheung et al. 2012c)
Humanized IgG1
Phase I
2.2 B7H3
4Ig-B7H3 is a costimulatory protein expressed on a wide range of pediatric solid tumors including >90 % of neuroblastoma tissue samples tested by immunohistochemistry, with restricted expression on normal tissues (Modak et al. 2001; Xu et al. 2009). It is thought to regulate tumor cell migration and invasion (Chen et al. 2008), and to inhibit NK cells and T cells (Sun et al. 2003; Flies and Chen 2007). 4Ig-B7H3 transcript is ubiquitously expressed in tumors and normal tissues by qRT-PCR but B7H3 protein was found only on tumors and not on most normal tissues both by Western blot and by immunohistochemistry (Sun et al. 2002; Chapoval et al. 2001). Expression of the 4Ig-B7H3 protein appears to be modulated by the micro RNA mIR-29. The binding kinetics of the anti-4Ig-B7H3 murine MoAb 8H9 when studied by Biacore testing revealed a slow dissociation rate (k off) which is crucial for sustained in vivo drug efficacy (Xu et al. 2009). Radioiodinated 8H9 targeted and suppressed rhabdomyosarcoma xenografts in mouse models (Modak et al. 2005).
2.3 L1CAM
L1-cell adhesion molecule (L1-CAM) is a member of the immunoglobulin superfamily of proteins and plays an important role in neuronal development in the fetus, specifically in axon guidance, neural cell migration, and differentiation. It is expressed on the cell surface of several human tumors including neuroblastoma, expression on normal tissues being restricted to nerves and kidneys. ChCE7 a high-affinity, internalizing chimeric anti-L1-CAM IgG1 MoAb has been studied in patients with neuroblastoma (Novak-Hofer et al. 1992; Hoefnagel et al. 2001).
2.4 Other Molecules for Potential MoAb Targeting
Significant experimental data exist for considering several other cell surface molecules as candidates for radioimmunotherapy (RIT). These include ALK (Carpenter et al. 2012), the neurotrophin receptor tyrosine kinase B (TrkB), the receptor tyrosine kinase-like orphan receptors (ROR) (Rebagay et al. 2012), and insulin-like growth factor 1 (IGF-1) and its receptor (IGF1-R) (Houghton et al. 2010). MoAbs against these targets are currently in various stages of preclinical evaluation.
3 Considerations for the Use of Radioimmunotherapy for Neuroblastoma
The radiation sensitivity of neuroblastoma leads to the use of external beam radiotherapy in several clinical scenarios: (A) External beam radiotherapy is considered the standard of care to prevent soft tissue recurrence in patients with neuroblastoma after achieving soft tissue remission (Matthay et al. 2009). (B) Isolated refractory skeletal sites are often irradiated in an effort to induce remission (Kushner et al. 2001a, 2009). (C) Patients with central nervous system relapses are treated with craniospinal radiation (Croog et al. 2010). (D) Palliative radiotherapy may assist with pain-control (Caussa et al. 2011). However, the toxicities associated with external beam radiotherapy are often severe: growth failure and asymmetry, learning difficulties, and other neurological and neuroendocrine sequelae, and an increased risk of secondary malignancy (Laverdiere et al. 2005). Targeted radionuclide therapy has the potential to diminish some of these toxicities by sparing normal tissue damage associated with external beam radiation. Indeed 131I-MIBG therapy has been extensively used for patients with resistant neuroblastoma. However, at the higher doses typically used, myeloablation occurs in most patients. Furthermore, response rates are suboptimal (<30 %) and usually transient (DuBois and Matthay 2008). A small number of patients have received 90Y-DOTATAC (Menda et al. 2010) or 177Lu-DOTATATE therapy without objective responses being observed (Gains et al. 2011). The former also carries the risk of possible renal damage. The availability of a valid cell surface target makes RIT an attractive proposition for high-risk neuroblastoma. RIT may be an effective adjunct to the established modalities of chemotherapy, external beam radiotherapy, and surgery, particularly against minimal residual disease which is typically distant and occult. Moreover, since most children with neuroblastoma are treated with immunosuppressive, high-dose chemotherapy, passive immunotherapy with MoAbs is feasible without rapid induction of neutralizing antibody responses, potentially permitting repeated administration of RIT.
The choice of radionuclide is a major consideration in the development of RIT, however, there are a limited number of agents for use in RIT in children. 131I is the only radionuclide that has been used clinically for RIT of neuroblastoma. Both 3F8 and 8H9 (Modak et al. 2005) are not internalized, thus dehalogenation has not been a major concern. 131I emits high energy gamma emissions that may be highly cytotoxic but may not be optimal for use in children due to concern for toxicity to normal tissues. Alpha particles, short-lived, high energy particles with short path length may have potential advantages for the targeting of the micrometastatic neuroblastoma cell clusters typically seen in the bone marrow. Indeed, 3F8 has been successfully radiolabeled with 225Ac and has been investigated in animal models (Miederer et al. 2004). For external scintigraphy, the high energy emitted by 131I limits dose and therefore sensitivity. Gamma imaging is limited in quantification as compared to PET that allows for superior assessment of radiation dosimetry. The positron emitter 124 I has a half-life of 4.2 days that allows for multiple time point imaging for assessment of bio-distribution and dosimetry, though has a complex decay scheme with only 25 % of the transitions resulting in positron emissions. 124I is the first and only positron emitter that has thus far been used to conjugate MoAbs for clinical use in children and 124I-3F8 has been used to develop a method to accurately measure kinetics and microdosimetry in humans (Daghighian et al. 1993; Pentlow et al. 1991).
A further consideration in the use of RIT in children is the maintenance of radiation safety precautions. This is a particular consideration in neuroblastoma, since most patients receiving RIT are <6 years of age. Radiation isolation or lead shielding is required for patients treated with high doses of 131I-MoAbs. Clear guidelines to parents and caregivers are necessary to ensure compliance with radiation safety precautions especially after discharge from hospital. External dose rate measurements can be used to estimate clearance and retention in the body and are highly relevant in the safe administration of RIT to children (Dauer 2007). Newer guidelines allow for outpatient therapy in those who are capable of maintaining precautions at home (Zanzonico and Siegel 2000).
4 Radioimmunodetection of Neuroblastoma
Imaging of tumors with radiolabeled MoAbs seeks to enhance the sensitivity and specificity of disease detection. More importantly, it provides vital pharmacokinetic information as well as dosimetry measurements, not easily available with conventional radiological imaging modalities. The use of SPECT and PET has added greatly to the precision in quantifying radioactivity targeted by radiolabeled antibodies in vivo. Four radiolabeled MoAbs have been used in clinical trials for radioimmunodetection of neuroblastoma: the anti-GD2 antibodies 131I-3F8 (Cheung et al. 1989), 131I-14G2a (Murray et al. 1994), and 99mTc-ch14.18 (Reuland et al. 2001) and the anti-L1-CAM MoAb 131I-chCE7 (Hoefnagel et al. 2001).
4.1 131I-3F8
The murine IgG3 MoAb 3F8 selective for GD2 was the first MoAb to be studied in patients with neuroblastoma (Cheung et al. 1985). It has the slowest dissociation rate among anti-GD2 antibodies (Cheung et al. 2012c) and mediates dose-dependent destruction of NB by human complement, lymphocytes (Munn and Cheung 1987), cultured monocytes, (Munn and Cheung 1989), and granulocytes (Kushner and Cheung 1936). 3F8 in combination with GMCSF has shown clinical utility for patients with chemorefractory osteomedullary disease and to consolidate first remission in high-risk neuroblastoma patients (Cheung et al. 1998). 131I-3F8 showed excellent tumor targeting in localizing to neuroblastoma at primary and metastatic sites in lymph nodes, bone marrow, and bone in 42 patients. When compared to 131I-MIBG, or to MRI scans, 131I-3F8 was more sensitive and more specific in detecting sites of metastatic disease. 18 of 20 patients with soft tissue disease demonstrated by CT/MRI had positive 131I-3F8 scans: upon surgical resection, one of the two negative tumors showed ganglioneuroblastoma and the other had only microscopic foci of residual NB (Yeh et al. 1991). 131I-3F8 had a relatively high tumor uptake of 0.08 to 0.1 % injected dose per gram (Larson et al. 1992).
4.2 131I-14G2a and 99mTc-ch14.18
The anti-GD2 murine MoAb 131I-14G2a was evaluated in the radioimmunodetection of NB detecting a majority of metastatic lesions (Murray et al. 1994). The chimeric MoAb ch14.18 consisting of the variable region of 1gG2a and the constant regions of human IgG1-K (Gillies et al. 1989) was studied In a recent seminal phase III study in combination with IL-2, GMCSF and cis-retinoic acid. Patients treated with this combination had improved progression-free and overall survival when compared to patients receiving standard therapy. Ch14.18 is now being evaluated for FDA approval. 99mTc-ch14.18 had a higher sensitivity and specificity than 131I-MIBG in the detection of NB relapses in 18 patients studied. Metastases were detected earlier with 99mTc-ch14.18 and tumor uptake persisted for a shorter duration after anti-neuroblastoma chemotherapy therapy was initiated when compared to 131I-MIBG, indicating possible disease response (Reuland et al. 2001).
4.3 131I-chCE7
131I-chCE7, a chimeric antibody that recognizes an L1-CAM isoform has also been used in the radioimmunodetection of patients with NB. In a group of seven patients with relapsed NB, sequentially imaged with 131I-MIBG and anti-L1(131I-chCE7), the latter targeted most tumor sites, although some discordance with results from conventional imaging modalities was observed (Hoefnagel et al. 2001).
5 Systemic Radioimmunotherapy for Neuroblastoma
Although anti-GD2 immunotherapy with “naked” MoAbs in combination with cytokines appears to have benefit in patients with minimal residual disease, it has limited clinical utility for patients with recurrent NB or those with refractory soft tissue or significant osteomedullary neuroblastoma. RIT may have a potential therapeutic role to play in these groups of patients.
131I-3F8 is the only MoAb that has been used for RIT of pediatric solid tumors, three early phase trials have been performed thus far. Toxicities were evaluated in a phase I dose escalating study (doses 6–28 mCi/kg) conducted on 24 patients with NB (23 patients with refractory stage 4 and 1 patient with unresectable stage 3 with ascites). Acute toxicities included expected grade 4 myelosuppression requiring autologous bone marrow rescue or GMCSF therapy, fever, mild diarrhea, and pain during infusion; the only late toxicity observed was hypothyroidism in spite of thyroid suppression. Maximum tolerated dose (MTD) was not reached. The calculated average tumor dose was 150 rad/mCi/kg, the average cumulative blood radiation dose was 740–888 MBq/kg at a dose of 20–24 mCi/kg, and the total body dose was estimated at 500–700 cGy, substantially less than total body irradiation dose used in myeloablative regimens that have been used as conditioning prior to autologous transplant for neuroblastoma (Cheung et al. 2001a; Larson et al. 2000). Six patients survived for more than 20 months after 131I-3F8 treatment and one patient died of progressive disease before bone marrow infusion; 10 patients were evaluated for response, 2 patients demonstrated complete response of the bone marrow disease, and 2 showed partial response of soft tissue disease (Modak and Cheung 2007; Cheung et al. 2001b). Based on the lack of extramedullary toxicities encountered in the phase I study, 131I-3F8 at a dose of 20 mCi/kg was added to a multimodality program (N7) for all high-risk NB patients. Forty-two newly diagnosed patients were enrolled and 35 completed treatment with 131I-3F8. All patients engrafted after bone marrow rescue. Among the first 20 patients treated with 131I-3F8, median time to achieve ANC >500/ul was 16 days and for platelet count >20,000/ul was 41 days. As in the phase I study, toxicities included myelosuppression, fever, and hypothyroidism, without any extramedullary toxicity. One patient died of infectious complications. With continued follow-up (6–10 years from diagnosis), overall survival for NB patients newly diagnosed at >18 months of age is ~40 % (Cheung et al. 2001b). Using the DOSCAL program developed at Memorial Sloan-Kettering Cancer Center that implements S-factor, average absorbed doses for liver, spleen, red marrow, lung, and tumor were 2.3, 2.5, 2.2, 1.9, and 13.7 cGy per mCi respectively (Larson et al. 2000). There were no unexpected late effects among children treated on this regimen. All patients developed human anti-mouse antibody (HAMA) response (Larson et al. 2000; Cheung et al. 1992; Modak and Cheung 2005).
External dose rate measurements varied with patient size and disease burden and in general correlated with dosimetry based on blood sampling. They were used to guide management and discharge planning of patients treated with 131I-3F8 (Dauer 2007).
Preclinical studies indicate that the combination of targeted radiotherapy and anti-angiogenesis effectively suppressed NB xenografts even at relatively low doses of 131I-3F8 (Modak et al. 2006). A clinical trial based on these observations has recently been completed in 24 patients with resistant NB (NCT01114555). 131I-3F8 was dose-escalated from 4 to 8 mCi/kg/dose, while the dose of the anti-angiogenic agent bevacizumab was maintained at 15 mg/kg/dose. Maximal tolerated dose (MTD) for 131I-3F8 was not reached. All patients developed grade 4 myelosuppression. Nine required autologous stem cell rescue (ASCR), all of whom engrafted at a median of 11 (range 4–15) days. Preliminary assessment of data indicate that bevacizumab did not appear to impair 131I-3F8 targeting to sites of NB or BM recovery after autologous stem cells rescue (Modak et al. 2010).
6 Compartmental Radioimmunotherapy for CNS Neuroblastoma
Despite eradication of systemic neuroblastoma metastases, isolated central nervous system (CNS) relapse is recognized with increased frequency. In published series, CNS relapse afflicted up to 16 % of patients otherwise in remission with median time to CNS relapse of 13–20 months from diagnosis (Blatt et al. 1997; Kramer et al. 2001b; DuBois et al. 1999). Risk factors include MYCN-amplified neuroblastoma and lumbar puncture at initial diagnosis (Kramer et al. 2001b; Matthay et al. 2003). Myeloablative chemotherapy as consolidation for initial disease did not prevent these CNS relapses, (Kushner et al. 2006) which evolved irrespective of induction regimens, or whether patients had received anti-GD2 antibody 3F8 or ch14.18. Unlike the beneficial effect of prophylactic CNS treatment in survival for patients with leukemia and small cell lung cancer (Vines et al. 2003) the rarity of CNS neuroblastoma makes prophylactic treatment difficult to justify. Once neuraxis metastases are clinically evident, conventional treatment modalities were generally considered palliative with a survival in most series of 4–14 months despite multimodality therapy including surgery for isolated lesions, stereotactic radiosurgery, craniospinal radiation, and intrathecal and systemic chemotherapy (Kramer et al. 2001b; Shaw and Eden 1992; Kellie et al. 1991). Compartmental radioimmunotherapy (CRIT), by virtue of prolonged residence time, and slow and incomplete transfer to the systemic circulation, has the potential to significantly enhance targeting to tumors located within that compartment while reducing systemic toxicity. The addition of CRIT with 131I-3F8 or 131I-8H9 administered via an Ommaya catheter into the cerebrospinal fluid (CSF) to a multi-modality treatment regimen (Table 2) has succeeded in significantly improving progression-free survival for patients with CNS relapse of NB to >65 % (Kramer et al. 2010).
Table 2
General treatment plan for patients with relapsed CNS NB
Time | Intervention |
---|---|
Day 0 | Neurosurgical resection of bulky disease; Ommaya catheter placement |
Day 7 | Irinotecan 50 mg/m2/dose daily × 5 |
Day 14 | Craniospinal radiation therapy 1080–2160 cGy/boost 2560–3000 cGy |
Week 7 | Irinotecan 50 mg/m2/dose daily × 5 |
Temozolomide 250 mg/m2/dose daily × 5 | |
Carboplatin 500 mg/m2/dose daily × 2 (only if systemic NB present) Stem cell rescuea | |
Week 14 | Indium 111-DTPA CSF flow study |
Serial injections of intraOmmaya 131-I-MoAbs | |
Week 30 | GMCSF/3F8 every 3–4 weeks (4 Cycles) |
Weeks 40–104 | Oral Cis-retinoic acid 160 mg/m2 day × 14 days alternating with oral temozolomide 75 mg/m2/day × 42 days (5 cycles) |
6.1 131I-3F8 for IntraOmmaya RIT
Fifteen heavily prior-treated patients (ages 1–61 years; median 9 years) with recurrent, progressive, or refractory leptomeningeal solid tumors including three patients with neuroblastoma received 31 injections (0.7–22 mCi) of intraOmmaya (IO) 131I-3F8 on a phase I study. Patients first received a tracer dose of 2 mCi 131I-3F8. Acute toxicities including headache, fever, vomiting were commonly observed in the first 24–48 h after injection. These toxicities were managed with supportive treatment (anti-emetics, acetaminophen, and narcotics) and were self-limited. Toxicities included headache, fever, and vomiting. Dose-limiting toxicity was reached at the 20 mCi dose, when transient elevations in intracranial pressure and chemical meningitis were seen. No long-term toxicity has been observed. Post-injection 131I-3F8 SPECT scans showed distribution throughout the subarachnoid space along the spinal cord down to the cauda equina by 4 h and progressively over the convexity by 24 h in all patients (Fig. 1). Total absorbed CSF dose was 1.12–13.00 Gy by sampling and 1.00–13.70 Gy by analysis of region of interest (ROI) data. Total absorbed doses to the ventricles and spinal column were 320–4150 cGy to the ventricles and 100–1370 cGy respectively. Blood dose was <2 cGy/mCi. Average dosimetry ratio (Gy/mCi) of the therapy/tracer administration was 0.88 (± 0.58) and 1.08 (± 0.66) based on CSF pharmacokinetics and ROI analysis, respectively. CSF half-life by sampling was 3–12.9 h. General agreement was seen for the CSF dose (cGy/mCi) achieved for test and therapy injections. Of the three neuroblastoma patients, one showed clearance of neuroblastoma cells in the CSF. A second patient with an isolated CNS relapse was treated with multimodality therapy in addition to IO 131I-3F8. He remains in complete remission now 9 years + since relapse without significant long-term side effects (Kramer et al. 2007).
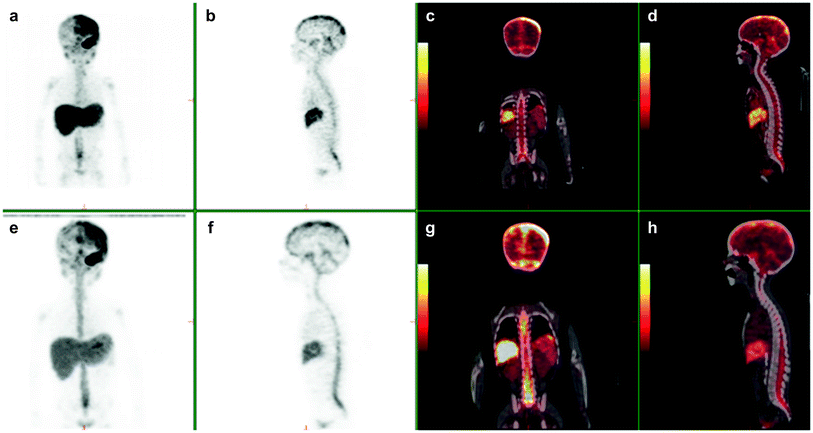
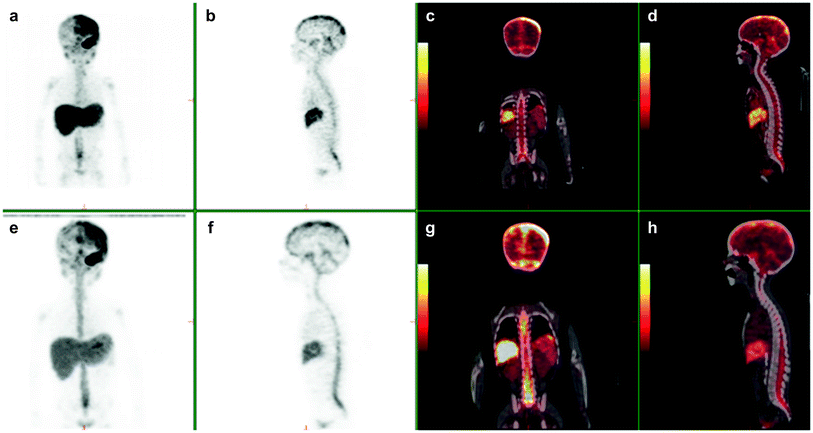
Fig. 1
3-yr-old with metastatic neuroblastoma. Patient was imaged at 2, 24, and 48 h following the intraventricular injection on 2 mCi of 124 I 8H9 via an ommaya reservoir. Maximum intensity projection images (a, e) show distribution in the body including the brain along the dura, ventricles and spinal canal. Sagittal (b, f) PET and fused (d, h) show diffuse distribution in the spinal canal. Physiologic uptake is seen in liver and excreted tracer activity is seen in the bladder (a, e and c, g)
Based on the phase I study, a phase II trial at MSKCC investigating the efficacy and cumulative toxicity of serial injections of 10 mCi 131I-3F8 in primarily pediatric patients with leptomeningeal solid tumors was initiated. Treatment is carried out in the outpatient setting with an initial dosimetry dose of 2 mCi 131I-3F8 or 124I-3F8 injection followed by 4 weekly serial injections of 10 mCi 131I-3F8 per injection, with a therapeutic goal of >2 injections (>20 mCi), dosimetry permitting. In the first phase of the trial, 4 out of 9 patients remained progression-free 6 months after completing therapy permitting the study to progress to the second phase which is ongoing. To date, 45 patients including 9 with neuroblastoma have received 2–4 therapeutic injections of intraventricular 131I-3F8. Two remain long-term survivors >5 years after therapy; 3 others did not experience a CNS recurrence of neuroblastoma.
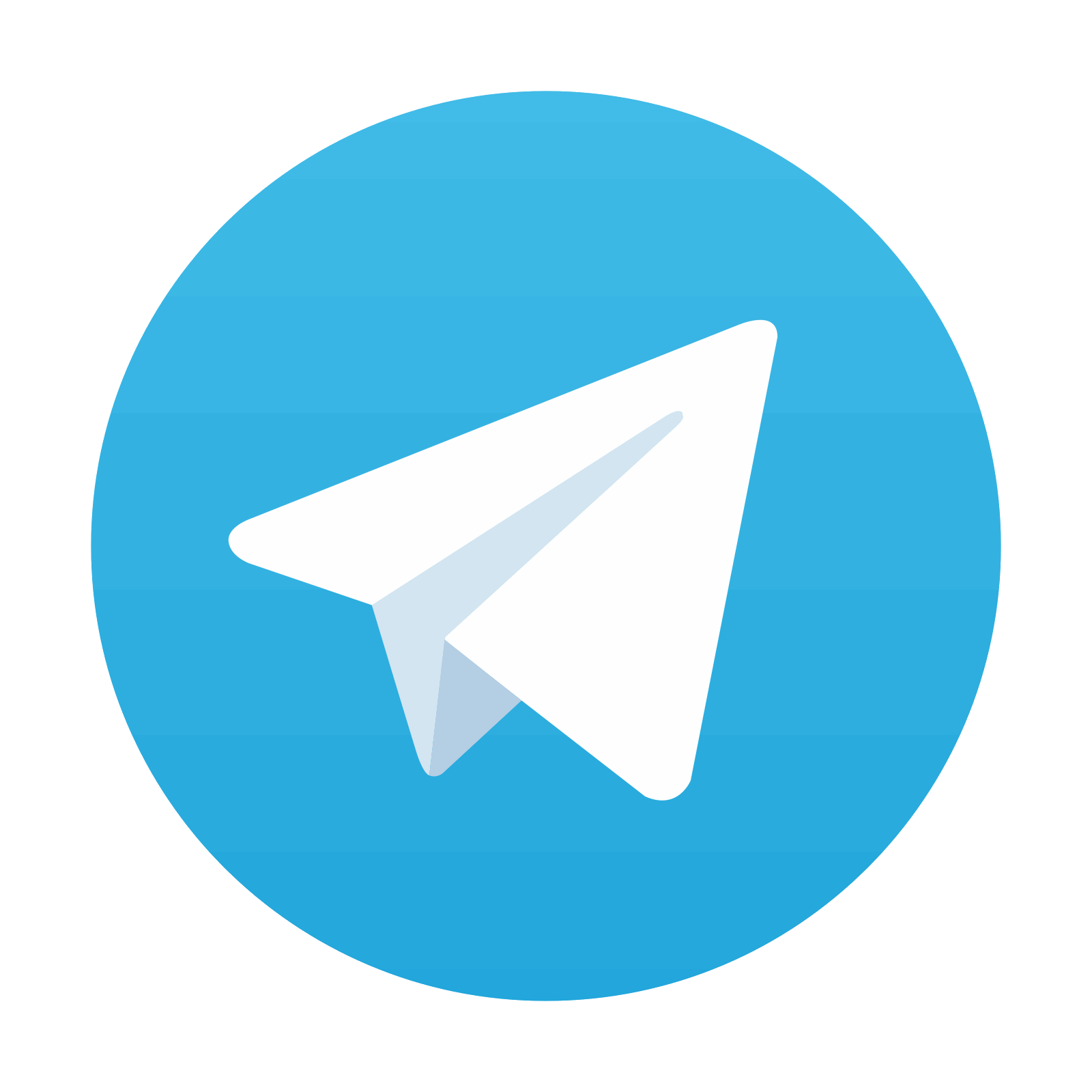
Stay updated, free articles. Join our Telegram channel

Full access? Get Clinical Tree
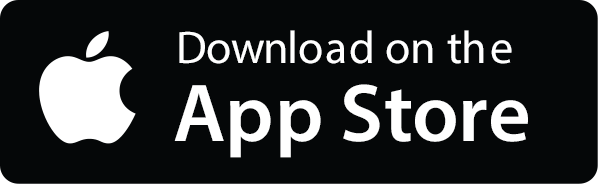
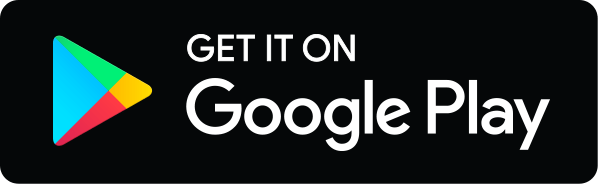