Fig. 1
WBS (a) and chest CT (b) performed after the 1st administration of 3.7 GBq of 131I ; WBS (c) and chest CT (d) performed after the 4th administration of 3.7 GBq of 131I
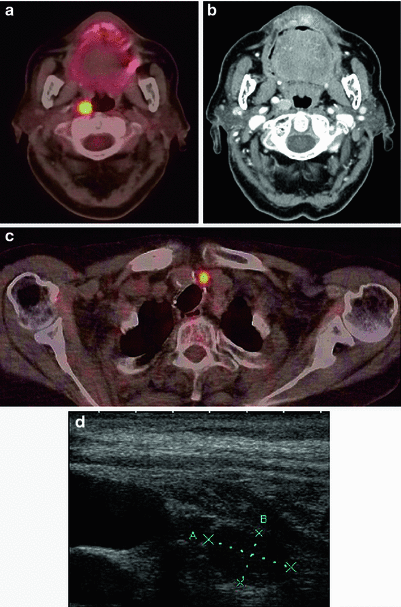
Fig. 2
Retropharyngeal lymph node diagnosed on FDG-PET/CT (a) and neck CT (b) and laterotracheal relapse diagnosed on FDG-PET/CT (c) and neck ultrasonography (d)
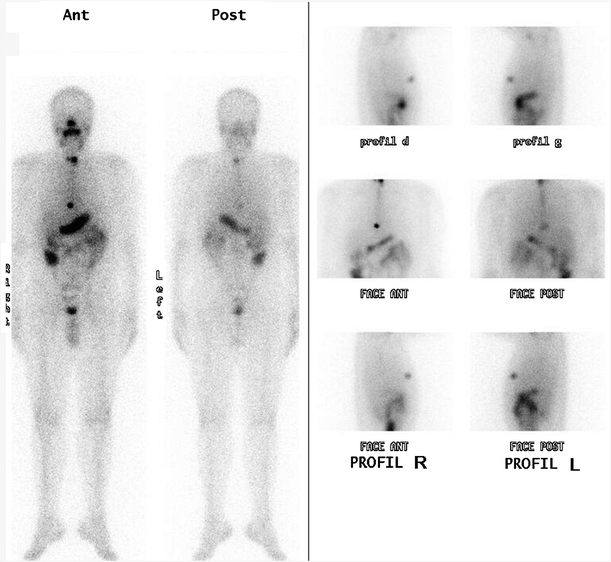
Fig. 3
Pleuropericardial cyst
2.2.2 Radiographs
Bone and chest radiographs are no longer routinely obtained for patients with undetectable serum Tg concentrations. The reason is that virtually all patients with abnormal radiographs have readily detectable serum Tg concentrations.
2.2.3 Serum Thyroglobulin (Tg) Determination
Thyroglobulin is a glycoprotein that is produced only by normal or neoplastic thyroid follicular cells, and should not be detectable in patients who have had total thyroid ablation (Van Herle et al. 1973). Serum Tg antibodies may induce falsely negative results in immunometric assays and should be quantitatively assessed at each serum Tg determination (Spencer et al. 2005). In patients who are in complete remission after total thyroid ablation, serum Tg antibodies when initially detected will decline gradually to low or undetectable levels, with a median time of 3 years (Chiovato et al. 2003). Their persistence or their reappearance during follow-up should be considered suspicious for persistent or recurrent disease.
There is a close relationship between tumor burden and the Tg level, both during levothyroxine and following TSH stimulation; this explains why serum Tg may be undetectable in patients with isolated lymph node metastases in the neck or with small lung metastases not visible on X-rays (Bachelot et al. 2002). Also, serum Tg level may remain low in patients with recurrent disease from a poorly differentiated thyroid cancer (Volante et al. 2007).
The production of Tg by both normal and neoplastic thyroid tissue is in part TSH dependent (Schlumberger et al. 1980; Schneider et al. 1981). High serum TSH concentrations can be achieved by withdrawing levothyroxine for 4 weeks. However, the resulting hypothyroidism is poorly tolerated by some patients. This effect can be attenuated by substituting the more rapidly metabolized liothyronine for levothyroxine for 3 weeks and withdrawing it for 2 weeks (Goldman et al. 1980; Schlumberger et al. 1980; Schneider et al. 1981). The serum TSH concentration should be above an empirically determined value (>25–30 mU/L) in patients treated in this way; if it is not, withdrawal should be prolonged until it is. Intramuscular injections of rhTSH (0.9 mg for 2 consecutive days) are an alternative because levothyroxine treatment need not be discontinued; therefore, hypothyroidism is avoided, quality of life is maintained, and side effects are minimal (Haugen et al.1999; Schlumberger et al. 2007b); as shown by medico-economic evaluation, benefits of rhTSH largely outweight its cost (Borget et al. 2007). After rhTSH stimulation, the peak of serum Tg is usually obtained 3 days after the second injection. Although the magnitude of increase in serum Tg is frequently less important following rhTSH than withdrawal, the diagnostic efficiency of rhTSH for stimulating Tg production in the serum is comparable to that of levothyroxine withdrawal in most patients (Haugen et al. 1999; Schlumberger et al. 2007b; Eustatia-Rutten et al. 2004).
Modern Tg assays with a functional sensitivity of 0.1 ng/mL have an improved sensitivity for the detection of persistent disease during levothyroxine treatment RhTSH stimulation may be avoided in low risk patients with an undetectable or low serum Tg level on thyroxine treatment (< 0.3 ng ng/mL) who have no evidence of disease, but remains useful in the other patients (Schlumberger et al. 2007a; Snozek et al. 2007; Brassard et al. 2011).
2.2.4 Iodine 131 Total-Body Scan
The results of a 131I total-body scan depend on the ability of neoplastic thyroid tissue to take up 131I in the presence of high serum TSH concentrations, which are achieved by either withdrawing levothyroxine as previously reported, or by administering intramuscular injections of rhTSH (0.9 mg for 2 consecutive days, with radioiodine administration on the day following the second injection) (Haugen et al. 1999; Schlumberger et al. 2007b).
When 131I scanning is planned, patients should be instructed to avoid iodine-containing medications and iodine-rich foods, and urinary iodine should be measured in doubtful cases. Pregnancy must be excluded in women of childbearing age. For routine diagnostic scans, from 2 to 5 mCi (74–185 MBq) of 131I is given; higher activities may reduce the uptake of a subsequent therapeutic activity of 131I. In fact, stunning is a transient decrease of 131I uptake due to previous irradiation of thyroid cells, that may decrease NIS expression in irradiated cells (Lassman et al. 2004; Nordén et al. 2007). This suggests that exposure to a low diagnostic activity of 131I should be avoided in patients who are to be treated with 131I.
The scan is done and uptake, if any, is measured 48–72 h after the activity, preferably using a double-head gamma camera equipped with thick crystals and high-energy collimators. 131I SPECT/CT fusion imaging may provide superior lesion localization, in particular for foci located in the neck, chest, or pelvis (Wong et al. 2008; Aide et al. 2009). False positive results are rare and are usually easily recognized.
2.2.5 Post-Iodine 131 Therapy Total-Body Scans
Assuming equivalent fractional uptake after administration of either a diagnostic or a therapeutic activity of 131I, an uptake too low to be detected with 2–5 mCi (74–185 MBq) may be detectable after the administration of 100 mCi (3,700 MBq). Therefore, a TBS is performed 3–7 days after the post-operative radioiodine administration (Cooper et al. 2009), and if this TBS is informative (uptake in thyroid remnants <1 %) and does not show any abnormal focus of uptake outside the thyroid bed, there is no reason to perform a less sensitive diagnostic control TBS during the subsequent follow-up, in the absence of any other abnormality (Cailleux et al. 2000; Pacini et al. 2002; Cooper at al. 2009). This is also the rationale for administering a large activity of 131I in patients with persistently elevated or increasing Tg levels (>10 ng/mL in the absence of levothyroxine treatment), even if the diagnostic scan is negative (Sherman et al. 1994; Schlumberger et al. 1997).
2.2.6 Other Imaging Modalities
These tests should be performed only in selected cases and may include spiral CT (with injection of contrast medium) or MR imaging of the neck and chest, bone scintigraphy, MRI of bones, and PET scanning using 18F-FDG. Indeed, no iodinated contrast medium should be injected during the 2 months preceding radioiodine administration.
FDG-PET scanning is performed both for diagnosing remote neoplastic foci and for prognostic assessment (Leboulleux et al. 2007b; Cooper et al. 2009). The sensitivity of FDG-PET scan may be improved by TSH stimulation, obtained following levothyroxine withdrawal or injections of rhTSH (Leboulleux et al. 2009). Low-risk patients are very unlikely to require FDG-PET scanning as part of initial staging or follow-up. FDG-PET scanning in thyroid cancer is used
to localize disease in thyroglobulin positive (>10 ng/mL), RAI scan negative patients; it is mostly useful for the detection of lymph node metastases in the central (level VI) and retropharyngeal neck areas and in the mediastinum, or distant metastases;
for the initial staging and follow-up of patients with poorly differentiated and Hürthle cell thyroid cancers, in order to identify sites of disease that may be missed with conventional imaging, and FDG uptake is usually high in these cancers;
in patients with known distant metastases in whom high FDG uptake in large metastases indicates a high risk for disease specific mortality and poor response to RAI therapy (Robbins et al. 2006);
finally, as a measurement of post-treatment response following local or systemic therapy of metastatic foci.
Inflammatory lymph nodes, suture granulomas, and increased muscle activity are common causes of false positive FDG-PET findings. Also, asymmetrical laryngeal uptake is frequently observed in patients with vocal cord paralysis. Therefore, cytologic or histologic confirmation is required before one can be certain that an FDG positive lesion represents metastatic disease.
2.3 Follow-up Strategy
If the total-body scan performed after the administration of 131I to destroy the thyroid remnants is informative (when uptake in normal thyroid remnants is low, < 1 %) and does not show any abnormal focus of uptake outside the thyroid bed, physical examination is performed and serum TSH and Tg are measured during levothyroxine treatment 3 months later (Table 1) (Schlumberger et al. 2004; Cooper et al. 2009). In most centers, at 9–12 months after initial treatment, a neck ultrasonography is performed and the serum Tg level is measured after thyroid hormone withdrawal or rhTSH stimulation. A diagnostic 131I total body scan is no longer routinely performed in low-risk patients with undetectable serum Tg, because the vast majority of patients with 131I uptake in their metastases also have detectable serum Tg levels (Cailleux et al. 2000; Pacini et al. 2002). Thus, ablation is currently established by an undetectable serum Tg level following TSH stimulation. Furthermore, neck ultrasonography can identify lymph node metastases that are too small to produce detectable serum Tg levels and that may be not seen on 131I total body scan either because of their small size or of the absence of radioiodine uptake as observed in a third of these patients (Frasoldati et al. 2003; Pacini et al. 2003; Torlontano et al. 2004). Tests performed at 9–12 months permit a reassessment of initial prognostication: patients with undetectable serum Tg following TSH stimulation and with normal neck ultrasonography are considered cured, the risk of long-term recurrence being less than 1 % (Cailleux et al. 2000; Pacini et al. 2002). They can be reassured and the dose of levothyroxine is decreased to maintain a serum TSH concentration within the normal range, between 0.5 and 2.5 mU/L. Clinical and biochemical evaluations with serum TSH and Tg determination on levothyroxine treatment are then performed annually; neck ultrasonography is performed in case of doubt or in high-risk patients, but any other testing is unnecessary as long as the patient’s serum Tg concentration is undetectable on levothyroxine treatment and the patient does not produce an interfering anti-Tg autoantibody; there is no indication to obtain another rhTSH stimulated Tg in these patients, at least for 5 years (Castagna et al. 2008). The use of sensitive assays for serum Tg determination permit to avoid the initial rhTSH testing at 9–12 months, in low risk patients who have undetectable or low (<0.3 ng/mL) serum Tg during levothyroxine treatment (Schlumberger et al. 2007a, b, c; Brassard et al. 2011). Such assays permit early diagnosis of persistent or recurrent disease and should indeed be used during follow-up (Snozek et al. 2007).
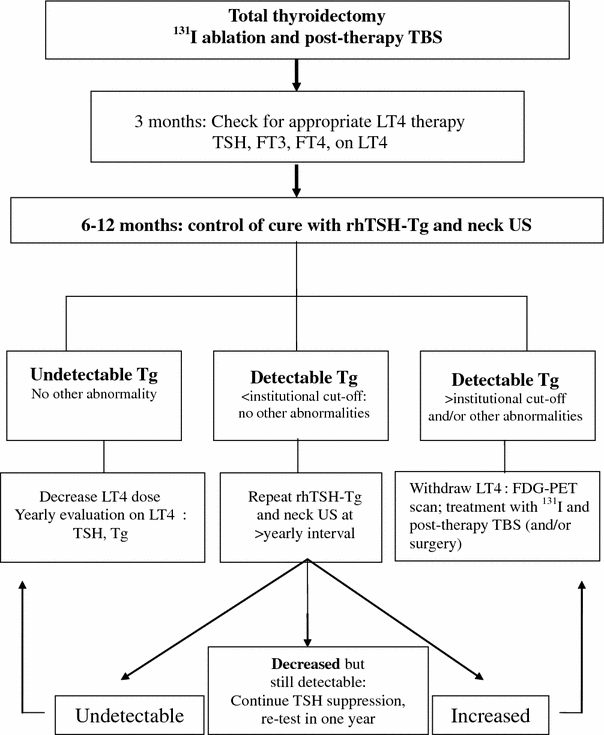
Table 1
Follow-up of thyroid cancer patients who had no abnormal uptake on post-therapy total body scan (from Schlumberger et al. 2004)
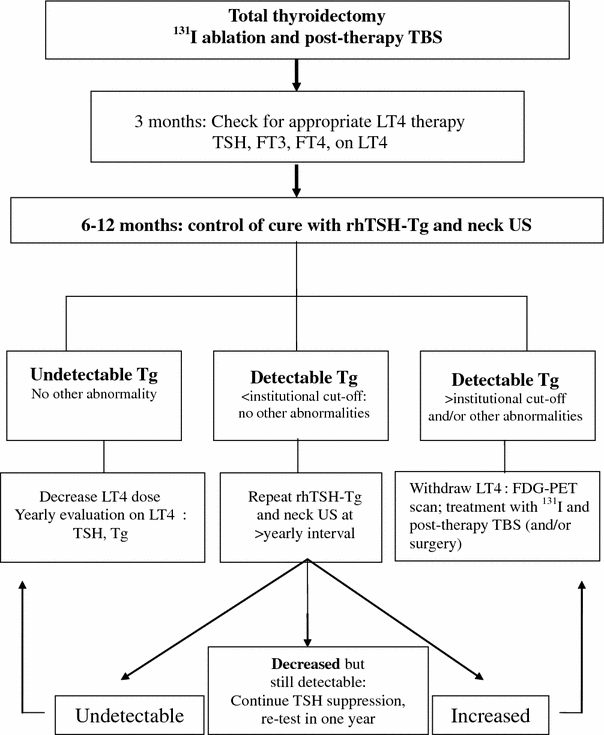
Suspicious abnormalities at neck ultrasonography should be submitted to FNAB, even if serum Tg remains undetectable following TSH stimulation. Lymph node metastases are indeed treated, but currently there is no evidence that treatment at a very early stage (when lymph nodes measure less than 5 mm in diameter) may improve the outcome as compared to treatment at a stage when they measure 5–10 mm in diameter. This is why only lymph node with a smaller diameter larger than 5–7 mm should be submitted to FNAB, and smaller lymph nodes should be carefully followed up.
At 9–12 months, serum Tg becomes detectable following TSH stimulation in 14–20 % of patients with no other evidence of disease. The discovery of any other abnormality will dictate specific treatments. In the absence of any other abnormalities, suppressive thyroxine treatment is maintained and another rhTSH stimulated serum Tg is obtained some months or years later, depending on serum Tg level and the clinical context. This second TSH stimulated serum Tg determination will be lower than the first one or even undetectable in the absence of any further treatment in two-thirds of patients who then will be considered cured. In fact, persistent thyroid cells may produce Tg for several months after 131I treatment and may disappear during the subsequent months. Serum Tg will remain elevated or will increase with time in the remaining third of patients who should then be submitted to an extensive work-up, which may include neck ultrasonography, chest spiral CT, bone scintigraphy, FDG–PET scanning and administration of a large activity of 131I with a TBS 3–5 days later, and clinical recurrence will likely be found in time. These data demonstrate that the trend in serum Tg level is probably more relevant than the actual serum Tg level by itself (Baudin et al. 2003).
In very low-risk papillary thyroid cancer patients who have had a total thyroidectomy but who were not given 131I postoperatively (Hay et al. 2002), the intensity of the follow-up strategy depends largely on the serum Tg level that becomes undetectable during thyroxine treatment in 95% of patients (Durante et al. 2012) and on neck ultrasonography. If despite adequate TSH suppression, the Tg is readily detectable and increases with time, an ablative 131I treatment may be necessary with a post therapy scan some days later. The follow-up protocol previously described is then applied.
In very low-risk patients who have initially undergone only a unilateral lobectomy for a single papillary thyroid microcarcinoma (Hay et al. 2008), yearly follow-up should consist of a careful neck examination and serum Tg determination during levothyroxine treatment. With time, ultrasonography is likely to show focal nodular abnormalities in the remaining lobe in most patients with detectable Tg concentrations. Usually, biopsies of these lesions can be performed under sonographic guidance, and most prove to be cytologically benign. However, if recurrent papillary thyroid carcinoma is found on biopsy, a completion thyroidectomy should be performed.
3 Local and Regional Recurrences
Local and regional recurrences occur in 5–20 % of patients with DTC. They are more frequently observed in patients with extensive neck disease including larger primary thyroid tumors, extension beyond the thyroid capsule, extensive lymph node involvement, and with poorly differentiated carcinomas or with particular histological features (Leboulleux et al. 2005). Recurrences in lymph nodes are more frequently observed in patients with papillary thyroid cancer; recurrences in the thyroid bed and in soft tissue are more frequently observed in patients with follicular and poorly differentiated thyroid carcinoma, and usually announce a dismal prognosis. More than a third of reoperations for persistent or recurrent disease are related to inadequate initial thyroid surgery (Kouvaraki et al. 2004). Small lymph node metastases with initial radioiodine uptake may be treated with radioiodine, but their persistence after 2 or 3 treatments should lead to surgery (Pacini et al. 1994a, b) , and it may be difficult to assess cure in these patients after several treatments with radioiodine (Bachelot et al. 2005). A recurrence that is palpable or easily visualized with ultrasonography, CT or PET scanning should be excised. Compartmental dissection of previously unexplored compartments with clinically significant persistent/recurrent disease while sparing vital structures is performed because microscopic lymph node metastases are commonly more extensive than would appear from imaging studies alone. Conversely, compartmental surgical dissections may not be feasible in the setting of compartments that have been previously explored due to extensive scarring and only a more limited or targeted lymph node resection may be possible (Cooper et al. 2009).
When radioiodine uptake is present in neoplastic foci, complete excision may be facilitated by total-body scanning 3–5 days after administration of 100 mCi (3,700 MBq) of 131I because additional tissue that should be excised may be identified. In some selected centers, surgery is performed 1 day after the total-body scan, typically using an intraoperative probe. The completeness of resection is verified 1–2 days after surgery by another total-body scan, and in one series this was achieved in 92 % of cases (Travagli et al. 1998). In the absence of detectable uptake, other methods may be used to facilitate the excision of small neoplastic foci located in scar tissue or in sites that are difficult to re-dissect, such as intra-operative ultrasound or pre-operative ultrasound-guided charcoal tattooing (Hartl et al. 2009). External radiotherapy is indicated in patients with soft tissue recurrences that cannot be completely excised and that do not take up 131I (Tubiana et al. 1985; Terezakis et al. 2008).
Some patients with papillary thyroid cancer who were not eligible for further surgery or 131I therapy have been treated for regional nodal recurrence with ultrasound-guided radiofrequency ablation or percutaneous ethanol injections (Hay et al. 2005). For tumors that invade the upper aero-digestive tract, patient outcome is related to complete resection of all gross disease, with techniques ranging from shaving tumor off the trachea or esophagus for superficial invasion with the preservation of function to more aggressive techniques in case of direct intraluminal invasion, including tracheal resection and anastomosis or laryngopharyngoesophagectomy (Gaissert et al. 2007; McCaffrey 2000). Surgery is usually combined with 131I and/or external beam radiation.
4 Distant Metastases
4.1 Presentation
Distant metastases may be present initially and may reveal the disease. In other cases, metastases are discovered after initial treatment of the thyroid tumor, either on the post-ablation TBS or during the subsequent follow-up, because clinical symptoms or more frequently because serum Tg level remains elevated and increases with time (Nemec et al. 1979; Casara et al. 1993; Pacini et al. 1994a, b; Dinneen et al. 1995; Pittas et al. 2000; Bernier et al. 2001; Lin et al. 2004; Durante et al. 2006). Distant metastases are more frequently observed in patients with younger or older age, extensive neck disease and with poorly differentiated carcinomas or with particular histological features.
Distant metastases are located in lungs (50 %), bones (25 %), lungs and bones (20 %), or at other sites including brain, liver, and skin (5 %) (Durante et al. 2006). Lung metastases are frequently asymptomatic. The pattern of lung involvement may vary from macronodular to diffuse infiltrates; enlarged mediastinal lymph nodes are often present in patients with papillary thyroid carcinoma, especially children. Miliary pattern is more frequently observed in children and in young adults with papillary carcinoma, and macronodules are more frequently observed in older patients with follicular carcinoma. Bone metastases are symptomatic (with pain or fracture) in more than 80 % of cases. They frequently involve the axial bones and proximal long bones. They are osteolytic and are poorly visible on standard X rays and on bone scintigraphy, and are best seen on CT scan, FDG-PET, or MRI. They are more frequently observed in older patients with follicular carcinoma.
Nearly all patients with distant metastases have high serum Tg concentrations unless the lung metastases are not visible on radiographs. When distant metastases are discovered, a complete imaging work-up is performed, including a spiral CT scan of the neck and chest with contrast medium, an MRI of the axial bones in case of bone metastases and of the brain, and a TBS with a large activity of radioiodine. Uptake of radioiodine is found in two-thirds of cases. A FDG-PET scan should also be performed both for diagnosing remote neoplastic foci and for prognostic assessment.
4.2 Treatment of Distant Metastases: Methods
No randomized controlled trials evaluating treatment of recurrent disease have been reported, and current practice is based on retrospective studies that frequently are monocentric and extend over several decades. Until now, treatment of patients with distant metastases has been based on thyroxine suppressive treatment, surgery when feasible or other local treatment modalities, and on 131I therapy when radioiodine uptake is present.
4.2.1 Thyroxine Suppression
Thyroxine treatment should be given at suppressive doses (with TSH level <0.1 μU/mL) to all thyroid cancer patients with distant metastases (Biondi et al. 2005; Jonklaas et al. 2006; Cooper et al. 2009). It stops or slows down the TSH-dependent tumor growth of well differentiated tumors. However, it does not inhibit TSH-independent tumor growth observed in poorly differentiated cancer, but even in these patients detectable or high TSH levels may enhance tumor growth. However, in older patients, benefits of low or suppressed TSH level should carefully be balanced with the risk of atrial tachycardia (Biondi et al. 2005).
During TSH suppressive treatment, Tg production may be low and radioiodine uptake is absent. TSH stimulation increases Tg production in blood, is needed to achieve radioiodine uptake, and slightly increases both the uptake of FDG in neoplastic foci and the number of detected neoplastic foci. However, in patients with known distant metastases, there is no need for TSH stimulation when FDG-PET scan is performed for prognostic assessment (Schlumberger et al. 2007c; Leboulleux et al. 2007b).
4.2.2 Radioiodine Therapy
Radioiodine uptake is present following TSH stimulation in 2/3 of patients with persistent or recurrent disease, more frequently in younger patients, with a well-differentiated histology and with small metastases (Table 2). The outcome of 131I therapy is related to the radiation dose delivered to the thyroid cancer tissue and to its sensitivity to radiation. The absorbed radiation dose mainly results from β emission and depends on the effective half-life in the tissue and the ‘radioactive concentration’—the ratio of total uptake to the mass of thyroid tissue. In general, in thyroid cancer tissue, the iodine concentration is lower and its half-life in the tissue is shorter than in normal thyroid tissue, resulting in a much smaller radiation dose.
Table 2
Recommendations for radioiodine treatment in thyroid cancer patients with metastases
1. Only patients with significant uptake in their metastases may benefit from radioiodine treatment |
2. Treatment should be administered following TSH stimulation, and withdrawal of thyroid hormone treatment should be preferred to rhTSH injections. Iodine contamination and pregnancy should be excluded |
3. The activity per treatment is either standard (3.7–7.4 GBq or larger) or based on individual dosimetry |
4. A total-body scan performed a few days after the administration of radioiodine may guide further treatment and obviate the need for diagnostic TBS before subsequent treatment |
5. The interval between treatments is at least 6 months, during which patients are treated with thyroxine at suppressive doses (TSH < 0.1 μU/mL) |
6. Treatment should be repeated as long as radioiodine uptake is present in metastases, up to the administration of a cumulative activity of 22 GBq. Then, the decision to give further radioiodine treatments should be taken on an individual basis. Efficacy of radioiodine treatment is assessed by conventional imaging, TBS with quantitation of uptake in neoplastic foci and serum Tg levels |
One study suggested that radioiodine alone can effectively treat tumor foci exhibiting 131I uptake as long as a radiation dose of at least 8,000 cGy is delivered, whereas response is virtually non-existent for doses below 3,500 cGy (Maxon and Smith 1990). This indicates that radioiodine treatment should be given only following maximal TSH stimulation and in the absence of iodine excess. Data obtained in very few metastatic patients suggest that the uptake and the resulting radiation dose in tumor foci might be lower with rhTSH than following withdrawal of thyroid hormone treatment (Potzi et al. 2006). Therefore, in patients with metastases, radioiodine treatment should preferably be given following withdrawal and the use of rhTSH should be restricted to metastatic patients who cannot undergo prolonged withdrawal of thyroid hormone treatment or in whom serum TSH does not increase to sufficiently elevated levels. This also led to the administration of large activities of radioiodine, either standard (3.7 to more than 7.4 GBq) or based on individual dosimetry that may also prevent excessive body radiation exposure (Van Nostrand et al. 2002; Tuttle et al. 2006). However, no study has ever demonstrated improved tumor response rate with the use of activities either higher than 3.7 GBq or based on individual dosimetry.
PET studies with 124I have shown that uptake and effective half-life are heterogeneous among tumor foci in a given patient, and also inside a given focus of uptake, and this may explain the ineffectiveness of 131I treatment in some patients (Sgouros et al. 2004; Jentzen et al. 2008). Furthermore, the dose distribution in tumor tissues may be heterogeneous at a cellular level, because of heterogeneous expression of NIS and the short path of β-rays from 131I in biological tissues (Schlumberger et al. 2007c). In patients with initial 131I uptake, the disappearance of uptake despite the persistence of tumor masses may signal the irradiation and disappearance of only functioning tumor cells.
Response to 131I treatment is also dependent on the tumor sensitivity to radiation, and among patients with radioiodine uptake is more frequently observed in young patients with well-differentiated thyroid tumors and when the lesions are small (<1 cm in diameter) (Durante et al. 2006). FDG uptake in metastases as quantified by SUV is closely related to these clinical prognostic parameters: responses to radioiodine treatment are observed in metastases with radioiodine uptake and with no FDG uptake. Metastases with high SUV values are likely to progress rapidly and will not respond to radioiodine therapy, even when radioiodine uptake is present (Robbins et al. 2006).
To avoid stunning, a transient decrease of 131I uptake due to previous irradiation of thyroid cells (Lassman et al. 2004), exposure to a low diagnostic activity of 131I should be avoided in patients who are to be treated with 131I.
4.2.3 Side Effects of 131I Exposure for Thyroid Cancer
Side effects of radioiodine exposure are mainly related to exposure of the body and in particular of organs that concentrate radioiodine. The renal clearance of radioiodine is greater and the body retention is lower when radioiodine is administered in euthyroid condition (following rhTSH stimulation) than in hypothyroidism (following withdrawal); the use of rhTSH will thus decrease the radiation dose to the bone marrow by one-third and also to several organs (Hänscheid et al. 2006; Remy et al. 2008).
Exposure of salivary glands may result in sialeniditis and in a subsequent decreased salivary flow, and should be minimized by abundant hydration (Mandel and Mandel 2003); irradiation of the lachrymal drainage system may induce lachrymal stasis (Kloos et al. 2002); loss of taste is frequent and is usually transient; gastric radiation exposure may be responsible for transient nausea and vomiting. Colon irradiation should be decreased by laxative treatment and bladder and gonadal irradiation should be reduced by abundant hydration and frequent mictions.
Tumor in certain locations, such as the brain, spinal cord, and paratracheal region, may swell in response to TSH stimulation or after 131I therapy, causing compressive symptoms that should be prevented by corticosteroid therapy for a few days. The use of rhTSH does not eliminate and may even increase the possibility of rapid swelling of metastatic lesions (Braga et al. 2001; Vargas et al. 1999). Pneumonitis and pulmonary fibrosis are rare complications of high dose radioactive iodine treatment given at short intervals of time. Dosimetry studies with a limit of 80 mCi whole body retention at 48 h and 200 cGy to the red bone marrow should be considered in patients with diffuse 131I pulmonary uptake (Song et al. 2006). In most patients with diffuse lung involvement, there is no respiratory sequellae of radioiodine treatment (Durante et al. 2006).
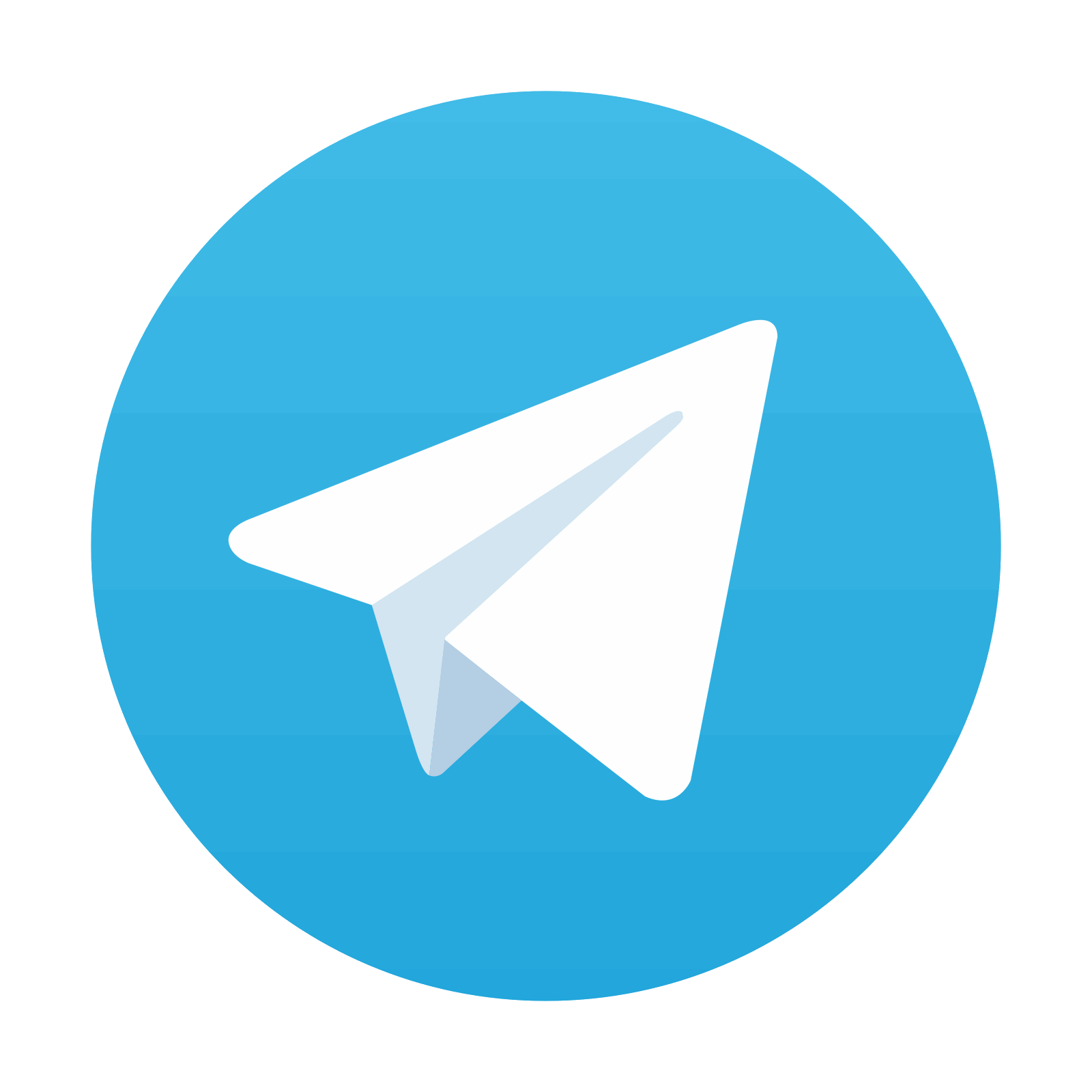
Stay updated, free articles. Join our Telegram channel

Full access? Get Clinical Tree
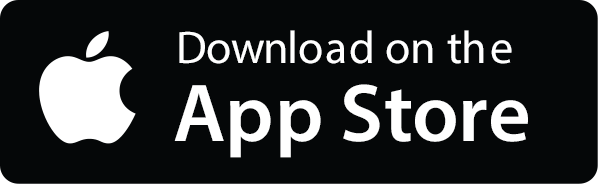
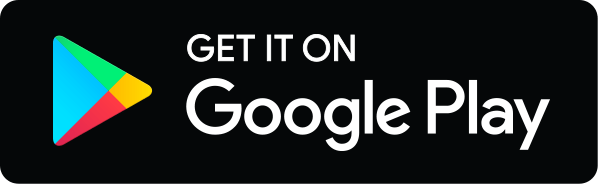