Vascular malformations
Vascular tumors
Low-flow malformations
Benign
Lymphatic malformations
Infantile hemangioma
Venous malformations
Congenital hemangioma
Capillary malformations
Tufted angiomas
Spindle-cell hemangioma
Epithelioid hemangioma
Pyogenic granuloma
High-flow malformations
Locally aggressive/borderline
Arteriovenous malformations
Kaposiform hemangioendothelioma
Arteriovenous fistulae
Retiform hemangioendothelioma
Papillary intralymphatic angioendothelioma
Composite hemangioendothelioma
Kaposi sarcoma
Complex malformations
Malignant
Two or more vascular malformations within the same lesion
Angiosarcoma
Epithelioid hemangioendothelioma
Although historically, clinicians and investigators who focused on vascular malformations involving the central nervous system (CNS) have worked in “splendid isolation” from those involved with extra-CNS lesions, it has become increasingly evident that complete separation of these areas is artificial and counterproductive. As there are diagnostic, natural history, and management issues unique to CNS lesions, this chapter has sections dedicated separately to CNS and extra-CNS vascular malformations. But it is apparent from any overview of pediatric vascular malformations that the bridges between the two areas are real and of critical importance, at the levels of anatomy, genetics, and treatment.
Imaging Techniques
For extracranial vascular malformations, the diagnosis is often highly suggested by an informed physical exam (Is the lesion warm and pulsatile? Is there local cutaneous ischemia, ulceration, or hemorrhage? Is the lesion purplish and expansile with recumbency or Valsalva?), and imaging generally plays a complementary role. Conversely, for intracranial malformations, imaging is often the alpha and the omega of diagnosis.
The imaging tools generally available for the assessment of these entities are ultrasonography (US), magnetic resonance imaging (MRI), magnetic resonance angiography and venography (MRA/MRV), computed tomography (CT), CT angiography (CTA), and digital subtraction angiography (DSA) [2].
For extracranial malformations, US is frequently the first-line imaging choice, due to lack of ionizing radiation, wide availability, ability to obtain real-time flow dynamic information, and lack of need for sedation. Drawbacks to this modality include operatory dependence and difficulty in imaging intracranial intraosseous lesional extension. However, in addition to its intrinsic diagnostic utility, US is extremely useful for image-guided therapy [3].
CT and MRI both provide excellent evaluation of the extent of malformations and their relationship to adjacent anatomic structures. Benefits of CT include rapid time of acquisition, which can limit the need for sedation. However, much of the patient population with vascular malformations is pediatric, and avoidance of unnecessary ionizing radiation is vital. As such, every effort should be made to utilize acceptable imaging alternatives, and if CT is necessary, scans should be limited to the area of interest only. On similar grounds, multiphasic scanning should be kept to a minimum. MRI and MRA provide excellent, potentially time-resolved, tissue and spatial resolution of vascular malformations, without the need for ionizing radiation. The most significant drawback of MR, particularly in the pediatric setting, is the need for sedation in the majority of patients.
Because of its temporal resolution, unmatched spatial resolution, and vessel selectivity, DSA has a critical role in evaluating high-flow vascular malformations, that is, those with an arterial component. DSA allows for precise mapping of involved anatomy and is an intrinsic part of catheter-based endovascular therapy. DSA rarely has a role in the workup or management of vascular malformations that do not harbor an arterial component and is rarely the first-line diagnostic modality for any lesion, due to its invasive nature and patients’ exposure to ionizing radiation.
Extracranial Vascular Malformations
Venous Malformations
Venous malformations occur with an overall incidence of approximately 1 per 10,000. They are generally soft, compressible lesions that are dark in color. Recent years have seen identification of genetic loci responsible for both inherited venous malformations (via germ line mutation) [4] and sporadic VM (via somatic mutation) [5]. Regardless of anatomic location, venous malformations can cause discomfort, often related to swelling or intralesional thrombosis. The classic presentation on clinical exam is that of a fluctuant swelling with dependent positioning or during crying or Valsalva maneuver. Depending on the location of the lesion, other symptoms, such as difficulty feeding, exophthalmos, or visual blurring, may also occur.
Various schemata have been proposed for the classification of venous malformations: types I–IV, based on the relationship of the lesion to surrounding venous drainage and the morphology of that drainage, and truncular versus extra-truncular types, based on the embryological stage at which the lesion was presumed to have developed [6]. Although most venous malformations are sporadic, inherited multiple lesions can occur in the context of syndromes such as blue rubber bleb nevus syndrome, manifest as numerous head, neck, and extremity venous malformations with the possibility of new lesions potentially developing throughout life.
On imaging, venous malformations are compressible lesions of heterogeneous echotexture on sonography (Fig. 1). Color Doppler interrogation often reveals slow monophasic flow, if any. If high-velocity or biphasic flow is encountered, an alternative diagnosis of high-flow vascular malformation should be entertained. CT can delineate the extent of involvement of the surrounding tissues and will also highlight phleboliths [3]. MRI also offers excellent delineation of the extent of involvement of these malformations. Though VM may have varied appearance on conventional T1-WI and T2-WI images due to the presence or absence of associated thrombus, they are generally hyperintense on T2-WI imaging and intermediate on T1-WI imaging. VM may demonstrate variable contrast enhancement (although nearly all do clearly enhance on delayed imaging) and a lobulated contour. Contrast enhancement is important in differentiating VM from non-enhancing vascular malformations, such as macrocystic lymphatic malformations. Internal signal voids on MRI may represent phleboliths within the VM. Catheter-based angiography is not helpful in the workup of VM and has almost no diagnostic role. In cases where angiography has been done, delayed passage of contrast through abnormal veins and sinusoidal spaces has been described, though angiographic appearance is more commonly normal [7].
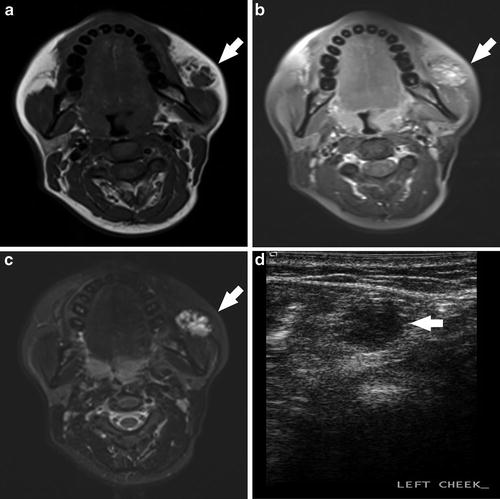
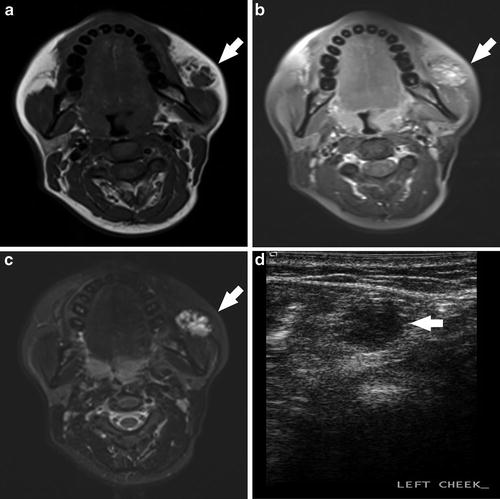
Fig. 1
A 24-year-old female visible left cheek “birthmark,” progressively expanding since age 8, with recent skin discoloration. Enlargement with Valsalva maneuvers was noted on examination. Pre- (a) and post- (b) contrast T1W images demonstrate moderate, heterogeneous enhancement and T2W (c) image demonstrates a hyperintense, multiseptated abnormality. Grayscale ultrasound (d) demonstrates a hypoechoic heterogeneous lesion. Clinical and imaging characteristics were consistent with a venous malformation
Therapy for venous malformations had traditionally been surgical [8], though sclerotherapy is the current treatment of choice. Sclerotherapy is generally painful and, as such, is most often performed under general anesthesia. Though easily manageable in most locations, post-sclerosis edema must always be considered in approaching these lesions. For example, orbital venous malformations leave little room for post-therapeutic swelling, and sclerotherapy in this location should be undertaken with the ophthalmology team available for urgent decompressive lateral canthotomy, if necessary; other options include surgical resection with or without associated endovascular liquid embolic occlusion. If there is no vision compromise, observation of these lesions is a reasonable option as well. Similarly, venous malformations of the head and neck associated with the airway, particularly those with baseline airway compromise and the need for repetitive treatments, may necessitate tracheostomy in conjunction with therapy. As mentioned below, bleomycin is associated with less post-procedural swelling than other sclerosants and can be an excellent choice in locations such as the orbit and airway.
Sclerotherapy is generally performed under sonographic and/or fluoroscopic guidance (Fig. 2) [9]. Results are best with localized venous malformations, and for very diffuse lesions, therapeutic goals generally focus around the most symptomatic portions of the malformation. One important consideration is the identification of rapid initial venous drainage. In those cases, manual compression of these draining veins for the initial 2–3 min of sclerosis can be helpful. In some cases, coil or liquid embolic occlusion of the outlet from the malformation to the adjacent draining veins can compartmentalize the lesion, ensuring that a large amount of sclerosant does not escape into the venous system. An additional technique for such situations is the double needle technique [10], whereby the malformation is accessed with two needles, using one for sclerosant injection and the other as a low-pressure escape valve, to reduce the risk of nontarget sclerosis.
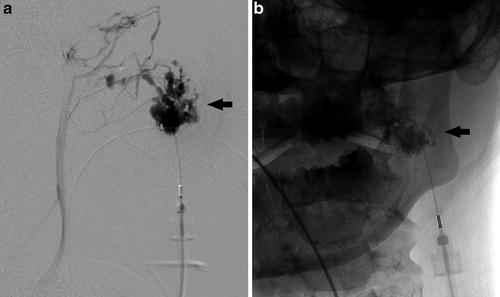
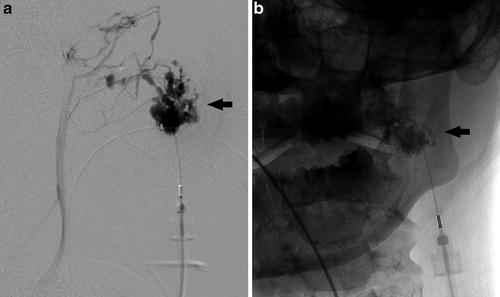
Fig. 2
Lateral projection from a direct percutaneous angiogram of the left cheek venous malformation (a) demonstrates a large, disorganized cluster of venous pouches, ultimately communicating with the normal venous drainage system. Frontal spot radiograph (b) during sclerosis with 3 % sodium tetradecyl sulfate (in a 2:1:1 mixture with lipiodol and air) demonstrates sclerosant within the malformation
The choice of sclerosing agent is dependent both on availability in a given country and on operator preference. Ethanol is the most potent of the sclerosing agents but is likely associated with the most serious potential morbidity; extravasation can result in severe damage or necrosis of adjacent soft tissue and nerve injury. Doses exceeding 0.75 mL/kg invariably result in intoxication [11]. Though there is a risk of cardiovascular collapse with ethanol, this is rare and can be avoided with careful, limited use, with the total dose kept below 1 mL/kg (maximum of 60 mL) and given gradually, in small aliquots. As head and neck lesions are rarely voluminous enough to require very large doses of sclerosant (as compared with the sometimes massive anomalies seen in truncal or limb lesions), the cardiac morbidity and pulmonary hypertension are rarely seen in this setting. In addition, sclerosants including alcohol cause hemolysis, which at a sizable scale may lead to hemoglobinuria. This is typically managed by generous hydration, urinary alkalinization, and close monitoring of urinary output [9], so other than for treatment of the smallest lesions, placement of a Foley catheter for these procedures is of great utility.
Given the potential soft tissue and nerve-related morbidity of ethanol sclerosis, many operators prefer other sclerosants, such as detergent agents. The most commonly used such agent is sodium tetradecyl sulfate (STS), with others in this class including polydocanol, sodium morrhuate, and ethanolamine. These are typically mixed with contrast agents such as Ethiodol for procedural visibility. Foaming of the sclerosant plus Ethiodol plus air is reported to increase efficacy, possibly due to increased surface area of the microbubbles generated for endothelial contact, as compared with a pure liquid [12].
Bleomycin, an antibiotic and antitumor agent originally derived from Streptomyces verticillus in 1966, is also used as a sclerosing agent for venous malformations. Given that bleomycin induces minimal posttreatment edema in comparison with other sclerosing agents, it can be of particular utility for lesions involving the airway. While bleomycin is associated with possible pulmonary fibrosis, this morbidity is seen at much larger systemic doses than are used with percutaneous sclerosis, with a threshold of >400 units. By comparison, the doses used for typical pediatric interventions are 0.5–1 mg/kg, and cases of bleomycin-associated pulmonary fibrosis have not been reported in the context of vascular anomaly sclerotherapy. Despite this, many groups obtain baseline pulmonary function tests and chest radiographs in patients undergoing bleomycin sclerotherapy and then continue to monitor pulmonary function after treatment.
Although not helpful as a primary treatment agent, as mentioned above, coils can be a useful adjunct, in particular, for malformations with rapid communication to adjacent veins. Liquid embolic agents, such as acrylate glue (nBCA) or ethylene vinyl copolymer (Onyx), although designed for the treatment of high-flow vascular malformations, can also be used to isolate venous malformations from adjacent veins into which they drain, allowing for safe and effective sclerotherapy.
Capillary Malformations
Capillary malformations are vascular lesions involving the superficial dermal layers and occur in 3 of every 1,000 newborns, darkening with age. Histopathologically, capillary malformations represent ectatic blood vessels in relatively denervated dermis. The most important feature of these lesions, aside from cosmesis, is their association with vascular syndromes such as Sturge-Weber syndrome, Klippel-Trenaunay syndrome, and Parkes Weber syndrome. In addition, it is commonly the case that capillary malformations involve the skin over an underlying fast-flow lesion, such as AVM, and at times, may involve the skin over lymphatic or venous malformations as well.
In the case of Sturge-Weber, which involves ocular and leptomeningeal abnormalities ipsilateral to the capillary malformation, the skin lesion itself generally conforms to a distribution tracking the trigeminal nerve subsegments. Up to 25 % of all patients with a V1 distribution capillary malformation have additional signs of Sturge-Weber syndrome [13], while the association is weaker with V2 and V3 distribution lesions. Neurological involvement in Sturge-Weber syndrome typically results in seizures, hemiplegia, and developmental delay.
The imaging evaluation of capillary malformations is primarily focused on assessment for associated pathology in underlying tissue. Doppler ultrasound is of particular utility in confirming or excluding associated arteriovenous shunting. Treatment of capillary malformations, aimed at improving cosmesis, is generally by way of pulsed-dye laser. Although only 15–20 % of lesions clear completely. For capillary malformations with underlying AVM or other vascular anomalies, laser therapy will not have real utility, and repeated attempts at treatment most often lead to skin injury with little benefit.
Lymphatic Malformations
Lymphatic malformations affect between 0.02 % and 0.05 % of the population, with half identified at birth and nearly all by 2 years of age [14]; they occur with equal incidence among females and males. The pathogenesis of these malformations is likely related to a defect occurring during embryonic lymphangiogenesis, whereby buds of lymphatic tissue become sequestered during development. Lymphatic malformations are most often cutaneous in location, though 10 % may involve the viscera. However, they are particularly trans-spatial in nature, frequently involving all types of soft tissue and bone within a region. Lymphatic endothelium may be confirmed pathologically with staining for the D2-40 antibody.
Lymphatic malformations (LMs) can be classified as microcystic, macrocystic, or mixed. Many authors suggest that cysts <2 cm be classified as microcysts and those >2 cm as macrocysts, while others advocate for classification based on typical appearance: those cysts appearing sonographically anechoic, with clearly delineated spaces and thin echogenic walls, are categorized as macrocystic, while those appearing as a near-solid echogenic mass are classified as microcystic [9]. As the classification system has most relevance in guiding treatment, this latter schema has utility in its favor.
Macrocystic lymphatic malformations are most commonly found in the axilla, chest, and neck and can frequently be identified on prenatal ultrasound. In that setting, important syndromic associations to consider include Down, Turner, or Noonan syndrome. On examination, macrocystic malformations are typically soft and compressible, although striking changes in size and firmness can occur with hemorrhage or infection. Mixed microcystic and macrocystic lymphatic malformations are common in the head and neck and can result in facial deformities; when involving the tongue or pharynx, airway compromise may occur, particularly in the setting of hemorrhage or infection. Syndromic associations have also recently been described with the CLAPO syndrome, with the initial description consisting of six patients with lower lip capillary malformation, face and neck lymphatic malformation, facial and limb asymmetry, and partial or generalized overgrowth. Interestingly, periorbital lymphatic malformations have been associated with intracranial vascular anomalies, including developmental venous anomalies, cavernous malformations, and others [15].
Imaging of lymphatic malformations is often focused on delineation of infiltrative components or to help refine differential possibilities when with dealing indeterminate cystic lesions. The specific appearance is dependent upon the macrocystic, microcystic, or mixed nature of the lesion. Macrocystic lesions have a multilocular cystic sonographic appearance without evidence of internal flow (Fig. 3). Layering debris from prior hemorrhage or infection may also be seen. Conversely, microcystic lymphatic malformations typically appear as an echogenic mass due to the density of acoustic interfaces within the malformation. By CT, macrocystic LMs typically appear as hypodense, septated masses [3]. MR imaging generally reveals hypointense T1-WI signal and hyperintense T2-WI signal with or without fluid-fluid levels. Post-contrast imaging may demonstrate mural or septal enhancement, but no enhancement is seen within cystic spaces of the malformation.
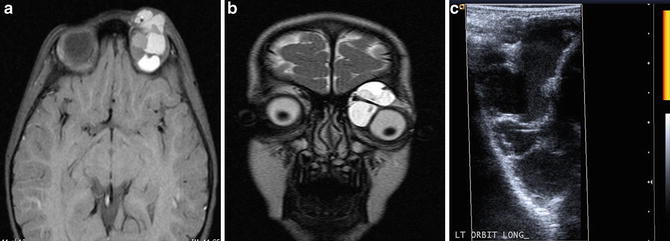
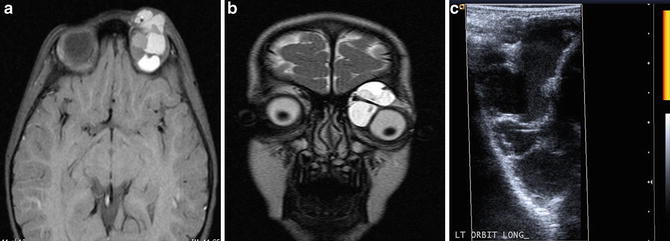
Fig. 3
A 3-year-old female with exodeviation and ptosis, leading to discovery of an orbital macrocystic lymphatic malformation. Axial pre-contrast T1W image (a) and coronal T2W image (b) demonstrate a multiseptated, heterogeneous left orbital malformation. Power Doppler ultrasound image (c) confirms a multiseptated cystic lesion, with no evidence of internal hypervascularity on power Doppler interrogation
Therapy of LM is guided by the size, area of involvement, and symptoms related to the malformation. Treatment modalities for macrocystic malformations include sclerotherapy, in some cases laser therapy, and surgical excision (Fig. 4). Unfortunately, recurrence is not rare after resection, and scarring and damage to adjacent nerves or blood vessels may occur. Sclerotherapy of LM typically involves cannulation of each cyst, in which fluid is then aspirated and a similar volume of sclerosing agent is injected. Some lesions may be so large as to necessitate placement of a pigtail catheter which can remain in place for several days, facilitating repeat aspiration and injection.
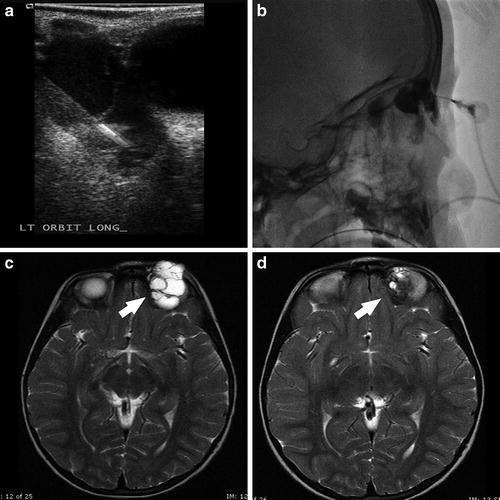
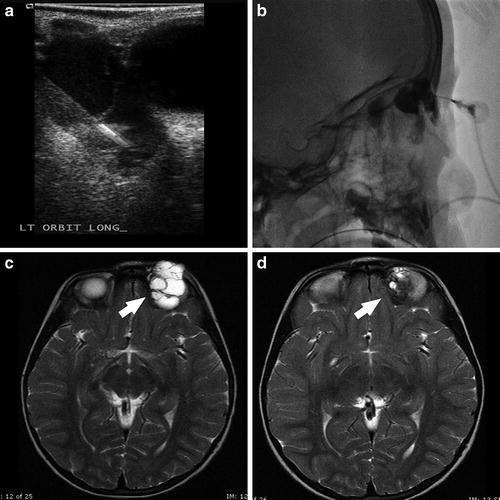
Fig. 4
A 3-year-old female with left orbital macrocystic lymphatic malformation. Grayscale sonographic image (a) demonstrates percutaneous needle access of the malformation, with confirmation of intralesional position noted on spot lateral radiography (b). Axial T2W images prior to (c) and following (d) sclerotherapy with doxycycline 10 mg/mL diluted in iodinated contrast demonstrate interval decrease in size of the malformation (white arrows)
Sclerosing agents used for lymphatic malformations include ethanol, doxycycline, sodium tetradecyl sulfate, bleomycin, alcoholic solution of zein (Ethibloc), and OK-432 (Picibanil) [9]. Doxycycline is the most commonly used of these agents, usually at a concentration of 10 mg/mL, due to its low morbidity and high efficacy; it is, however, painful on injection, and requires general anesthesia, even in adults. In neonates, doses greater than 150 mg in a single treatment setting are avoided, due to risks of metabolic acidosis, hypoglycemia, and hemolytic anemia. OK-432 (Picibanil), derived from a low-virulence strain of Streptococcus pyogenes, is not currently approved for use in the United States. A large prospective randomized trial of OK-432 demonstrated that ~94 % of patients with macrocystic lymphatic malformations had regression or resolution of their malformations [16]. Alcoholic solution of zein is a combination of corn protein (zein), oleum in ethanol, and sodium diatrizoate, also not available in the United States but used in Canada and Europe, with outcomes comparable to other agents [17].
In the natural history of LM, superinfection is frequent. As such, many practitioners give prophylactic antimicrobial therapy during and following sclerotherapy, with some extending this for 7–10 days following treatment. Involution of cysts is typically assessed at 4–6 weeks post-procedure, with staged treatments generally occurring at 6–8-week intervals.
Microcystic malformations are technically more difficult to treat, tend to have poorer response to therapy, and tend to recur after treatment more frequently than macrocystic LM [18]. Direct intralesional injection with pingyangmycin (a bleomycin variant), as well as radiofrequency ablation [19], has been described as showing efficacy.
Extracranial AVM
In comparison to low-flow vascular malformations, extracranial high-flow vascular malformations are rare, with a slightly higher prevalence in females. As is true for most vascular malformations, AVMs generally grow in proportion to the child, but they have been shown to grow rapidly during puberty, pregnancy, infection, and trauma [20]. Some extracranial AVMs have been associated with mutations of the RASA1 gene [21]. RASA1 encodes for a p120 Ras GTPase-activating protein which itself is involved in angiogenesis and cell adhesion, and children harboring mutations present with a combination of skin capillary stains and a propensity to develop AVMs throughout the body (CM-AVM). The PTEN gene on chromosome 10q has also been associated with AVMs. This gene encodes a tumor suppressor protein involved in regulating angiogenesis, cell growth, and cell proliferation, and mutations are seen in multiple eponymous syndromes, such as Bannayan-Riley-Ruvalcaba and Cowden syndrome (Table 2). The preferred designation for syndromes resulting from such PTEN mutations is PTEN hamartoma tumor syndrome (PHTS). Interestingly, greater than half of these patients have high-flow vascular anomalies of the head and neck. However, these are unlike typical AVMs, in that they often have ectopic fat within or adjacent to the malformation, disproportionate dilation of draining veins, and multiple non-contiguous sites of involvement [22]. PTEN-associated arteriovenous lesions tend to respond poorly, if at all, to embolization.
Table 2
Syndromic associations with high-flow vascular malformations
PHACES |
Lumbar |
Parkes Weber |
CM-AVM |
Cobb |
Osler-Weber-Rendu |
Wyburn-Mason |
PTEN hamartoma tumor syndrome |
In the head and neck, AVMs most frequently manifest with symptoms of hemorrhage, pain, or deformity. Particularly because total cure is so elusive and can only be entertained for the smallest of lesions, where complete resection might be possible, therapeutic goals are typically focused on symptomatic control, on preservation of critical functions such as vision, and on cosmesis. There is no universal treatment algorithm, with different teams not infrequently opting for different embolic agents, frequency of treatment, modality of treatment, etc. In those few cases where complete cure is the goal of therapy, the best results seem to occur with preoperative embolization followed by surgical resection.
The choice of embolic agent is dependent on the overall context of therapy for a given patient. Specifically, preoperative embolization can be effectively performed with short-term embolic agents such as PVA particles, Gelfoam, or Embospheres. While these provide excellent devascularization for the purposes of reducing intraoperative blood loss, these are not durable embolic agents, and thus, if the goal of treatment is permanent (i.e., anything other than preoperative embolization), liquid embolic agents, such as ethylene vinyl alcohol copolymer (Onyx), ethanol, and n-butyl cyanoacrylate (nBCA) glue, are preferred. nBCA is a clear, liquid, monomeric substance which is frequently mixed with tantalum powder and Ethiodol for radiopacity. Exposure to anions in blood causes the glue to polymerize and form a cast that is adherent to the endothelium, with the rate of polymerization of the glue controllable by the glue-to-Ethiodol ratio. In addition to the acute inflammatory fibrosis caused by the glue, there is a delayed foreign-body giant cell granulomatous reaction [23]. Onyx is a liquid embolic agent that precipitates at its surface in contact with blood, while maintaining a liquid core. Thus embolization technique consists of the formation of a proximal plug around the microcatheter tip, with further infusion allowing for controlled delivery of the liquid embolic, with longer and more extensive embolization than is possible with nBCA. Onyx additionally causes less endothelial damage and incites less inflammatory response than nBCA, resulting in improved handling of embolized malformations during surgical resection. Some advocate ethanol, which results in endothelial denudation, as the embolic treatment of choice [24], though with potentially the greatest morbidity risk.
The sonographic appearance of arteriovenous malformations is characterized by multiple hypoechoic vascular channels, without a well-defined soft tissue mass. Doppler analysis reveals arterialized draining venous waveforms [3]. CT angiographic imaging demonstrates increased caliber of feeding arteries and enlarged draining veins. Similar findings are also noted on MRI, where the rapid flow may also manifest as abnormal flow voids on spin-echo sequences. On all imaging modalities, the absence of an enhancing soft tissue mass is critical in distinguishing these lesions from others. DSA is virtually never needed to establish the diagnosis. Dedicated diagnostic catheter angiography is the routine standard of care for intracranial lesions, where multiphasic evaluation of flow dynamics to clearly identify arteriovenous shunting, precise delineation of arterial inflow, nidal vessels, and venous drainage and superselective angiography for identification of normal parenchymal branches arising from feeding pedicles is critical [25]. In contradistinction, for extracranial AVM, diagnostic angiography can typically be performed as part of the first session of embolization.
In terms of location within the head and neck, midface lesions, supplied by the internal maxillary artery, are disproportionately represented. The cheek and ear are relatively common sites, with the latter generally confined to the outer ear and possible extra-auricular spread; middle and inner ear involvement virtually never occurs (Fig. 5) [26]. Because arterial supply to ear AVMs is almost always from end arteries, complete endovascular embolization may unavoidably induce tissue necrosis and lead to amputation. As such, ear AVMs are generally observed until symptoms necessitate intervention. Although amputation and plastic surgical reconstruction may be the likely end point, staged palliative embolizations can delay amputation for several years.
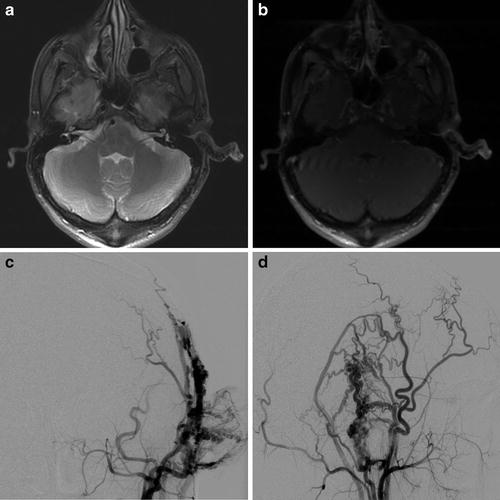
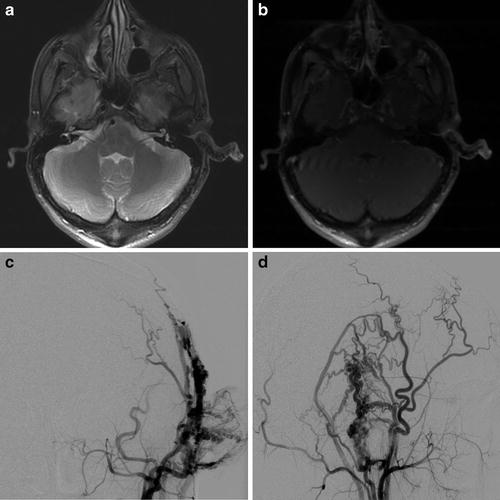
Fig. 5
A 21-year-old male with pulsatile tinnitus and progressive enlargement of a soft left auricular “mass.” Axial fat-saturated proton density (a) and T1W post-contrast (b) MR images demonstrate asymmetric enlargement of the left external ear with multiple small flow voids. AP (c) and lateral (d) projections from a left external carotid angiogram demonstrate abnormal arteriovenous shunting, consistent with a left auricular arteriovenous malformation
Mandibular and maxillary arteriovenous malformations pose unique risks for life-threatening hemorrhage associated with dental eruptions or dental extractions. Arterial supply to maxillary malformations is usually from internal maxillary branches, though ascending pharyngeal branches, labial branches of the facial artery, and posterior ethmoidal artery branches may also contribute (Fig. 6). Given the intraosseous nature of these malformations, surgical resection is challenging and necessitates bony excision and reconstruction with graft. While some authors advocate for a combined embolization and surgical approach from the outset for these lesions, others have reported that most can be controlled with embolization alone [27]. High-volume vascular anomaly centers often use embolization as first-line therapy for newly discovered maxillary or mandibular AVM and to approach any loose tooth or required dental extraction with a further preparatory embolization, with extraction in the angiography suite.
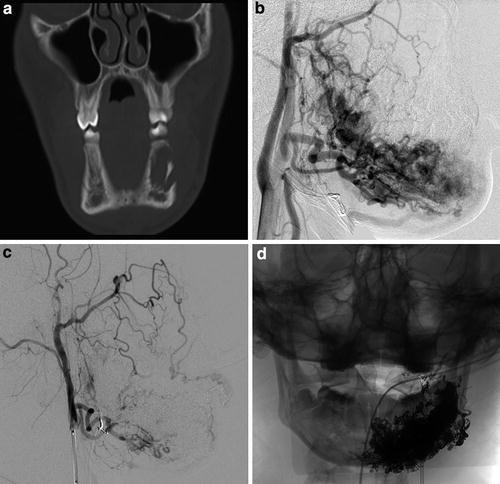
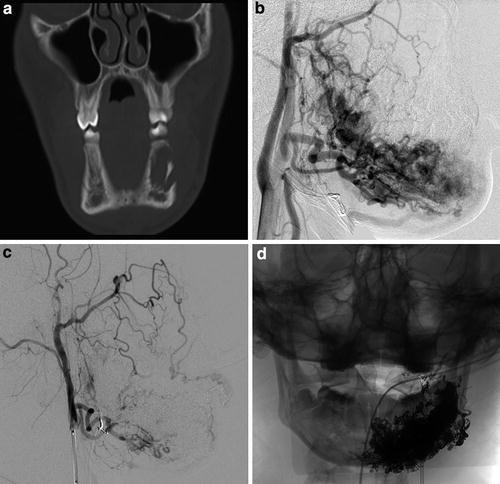
Fig. 6
A 16-year-old male with purple left perimandibular discoloration and growth, with complaint of aching left mandibular pain with prolonged mastication. Coronal CT image (a) displayed in bone windows demonstrates abnormal irregular lytic lesion in the left body of the mandible with cortical disruption. Lateral projection from left external carotid angiography (b) demonstrates arteriovenous shunting with a primarily intraosseous left mandibular arteriovenous malformation. Repeat lateral projection image from left external carotid angiography (c) and frontal spot radiograph (d) obtained following Onyx liquid embolization demonstrate interval occlusion of the majority of the malformation
Sinus Pericranii
Sinus pericranii is a link between extracranial and intracranial vascular anomalies. Patients with this condition have a transosseous venous bridge allowing for direct flow between intracranial and extracranial venous drainage pathways (Fig. 7). The intracranial contributor flowing into the transosseous bridge may be a venous sinus (with the superior sagittal sinus being by far the most common) or a pial vein or developmental venous anomaly. Sinus pericranii is rare overall, most commonly involves small venous channels, and is infrequently symptomatic, with incidental discovery on imaging being the rule. When the condition does present clinically, the most common manifestation is as a nonpulsatile mass that expands with recumbency, crying, or Valsalva. The extracranial, scalp portion of the sinus pericranii may have the mass-like, globular configuration of a venous malformation, rather than the morphology of a tubular venous channel. These cases are clinically apparent from birth and are most commonly located frontally, running from the glabella up toward the hairline, manifesting as a large, purplish, expansile lesion. Though in a minority of cases, sinus pericranii may occur secondarily as a result of trauma, the vast majority of cases are congenital, and they are frequently associated with intracranial developmental venous anomalies [28].
