Fig. 3.1
Cerebellar anatomy. Comparison between schematic representations of the cerebellar vermis in a sagittal section (a) with a midsagittal section of an MR autopsy in a fetus of 19 GW(b). The numbers in A represent the nine different lobules: 1 lingula, 2 central, 3 culmen, 4 declive, 5 folium, 6 tuber, 7 pyramid, 8 uvula, and 9 nodulus. The arrows in (a) and in (b) indicate the primary fissure; the dotted arrow in (a) and in (b) indicates the posterolateral fissure. The other three fissures are indicated by dotted lines in (a) from the superior to the inferior part of the vermis; they are, respectively, the horizontal fissure, the prepyramidal fissure, and the secondary fissure. The three cerebellar lobes are indicated in (a): the anterior lobe is included beyond the circle line traced from the fourth ventricle anteriorly to the primary fissure posteriorly; the posterior lobe in included beyond the circle dotted line traced from the primary fissure posteriorly to the posterolateral fissure anteriorly; finally the flocculonodular lobe is beyond by the scarcely dotted line drawn from the posterolateral fissure to the fourth ventricle. Even with high resolution of the MR autopsy, it is not possible to clearly identify all the nine lobules (b) (Drawings of this chapter by F. Triulzi)
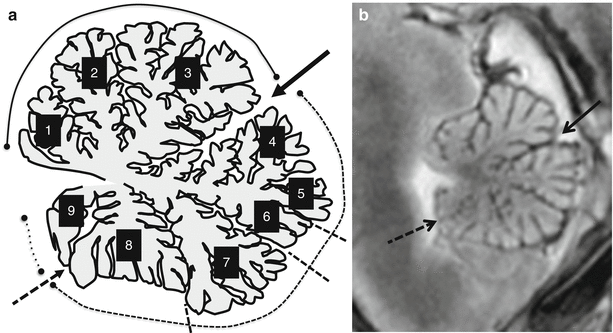
Fig. 3.2
Cerebellar anatomy. Comparison between the same scheme of Fig. 3.1 (a) and a midsagittal section of an MR autopsy in a fetus of 34 GW (b). At this stage all the different lobules and fissures are detectable on MR autopsy
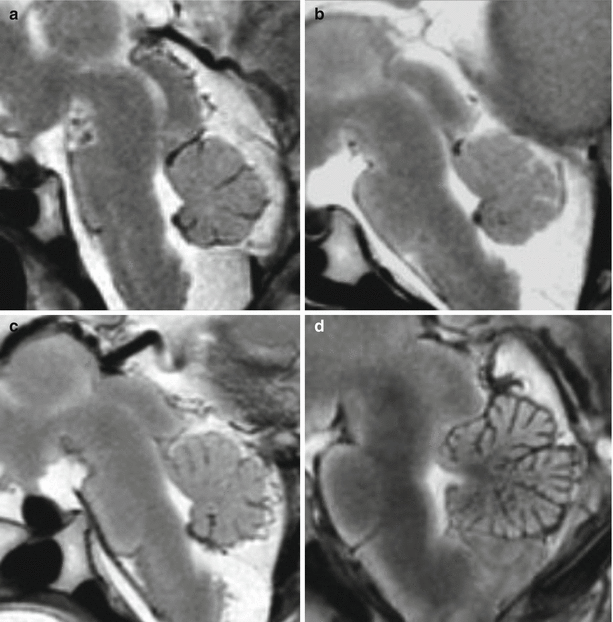
Fig. 3.3
Cerebellar anatomy. Progressive development of cerebellar vermis on MR fetal autopsy. Midsagittal section at 19 (a), 21 (b), 22 (c), and 34 (d) GW
The primary fissure is the most important landmark in the growing vermis, being easily recognizable on a standard midsagittal fetal MR image [4–6], where the development of the cerebellar vermis can be easily followed (Figs. 3.1, 3.2, 3.3, and 3.4). The anterior lobe of the vermis comprises three lobules: the lingula, the central lobule, and the culmen. The posterior lobe of the vermis is subdivided into three major fissures: the horizontal, the prepyramidal, and the secondary fissures. The primary and horizontal fissures border two lobules: the declive and folium vermis, whereas the horizontal and prepyramidal fissures border the tuber vermis. The prepyramidal and secondary fissures border the pyramid of vermis, and the secondary and posterolateral fissures border the uvula; finally, the posterolateral fissure and the fourth ventricle border the nodule and flocculonodular lobule from the posterior lobe of the cerebellum (Figs. 3.1 and 3.2).
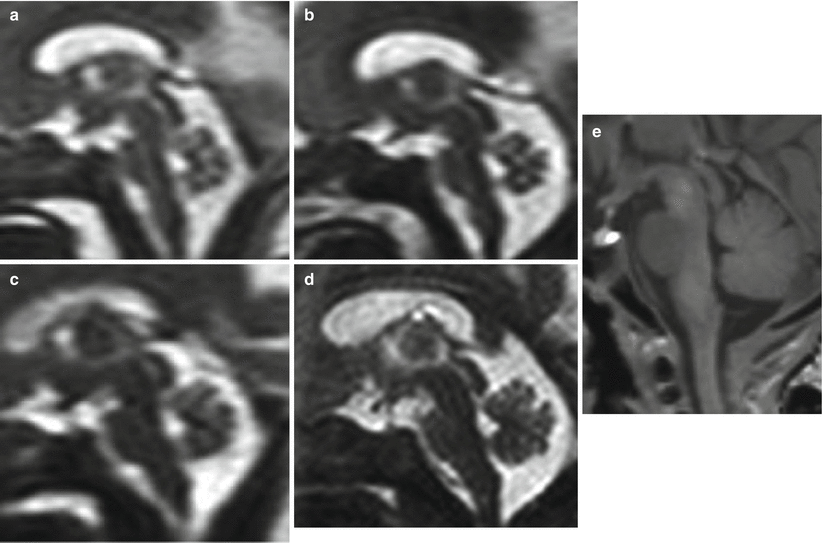
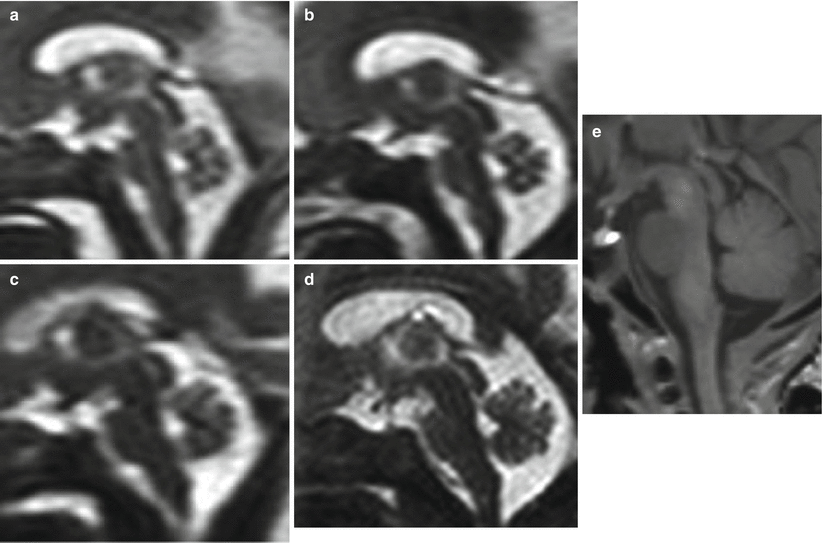
Fig. 3.4
Cerebellar anatomy. Progressive development of cerebellar vermis on in vivo fetal MR. Midsagittal section at 22 (a), 24 (b), 27 (c), and 30 (d) GW and comparison with normal midsagittal anatomy on a T1-weighted image in a normal neonate (e)
Even though all these main fissures as well as the primordial tissue of the different lobes are present by the 14th gestational week, it is quite impossible to detect all fissures and lobules on fetal MR during the 19–22 GW period. However, the primary fissure as previously mentioned can usually be detected by the 20–21 GW providing a reference point for the relative growth process of the anterior and posterior lobes (Fig. 3.4). The primary fissure becomes much deeper within the vermis than within the hemispheres, whereas the horizontal fissure becomes progressively deeper within the hemispheres than within the vermis.
From a phylogenetic point of view, the cerebellum is subdivided into three parts: the archicerebellum that is essentially the flocculonodular lobe, the paleocerebellum that roughly corresponds to the vermis, and the neocerebellum that forms the hemispheres.
During fetal life, the flocculonodular lobe is the first to be visualized, being detectable as early as the 10th week of gestation. The neocerebellum grows less rapidly than the vermis during fetal life, and this aspect can be easily appreciate on coronal and axial fetal MR images which show at 19–20 GW the cerebellar hemisphere still poorly developed (Figs. 3.5 and 3.6). On the contrary after birth, the neocerebellum increases rapidly in size, proportionally more rapidly than the cerebral hemispheres [4, 5].
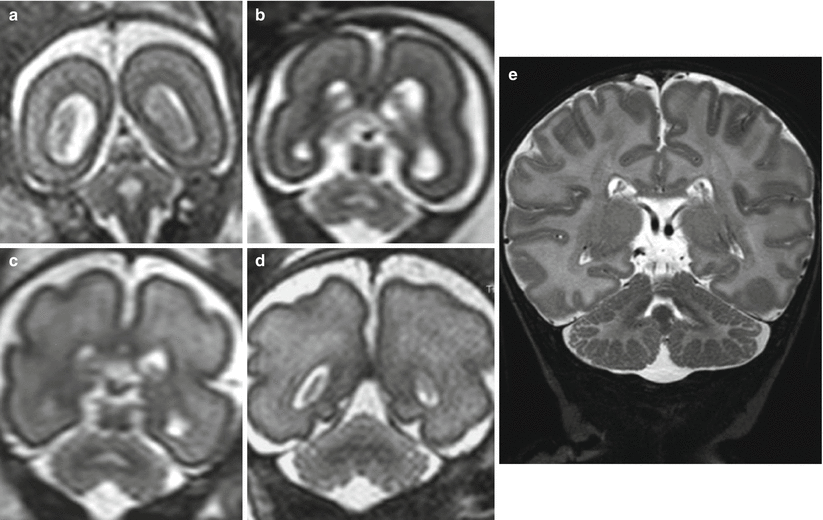
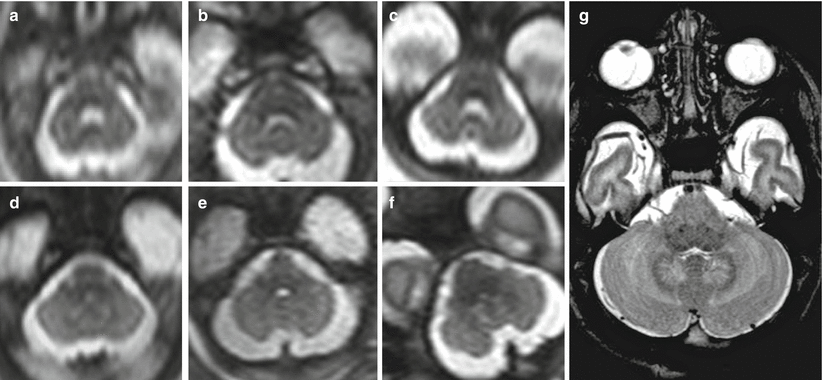
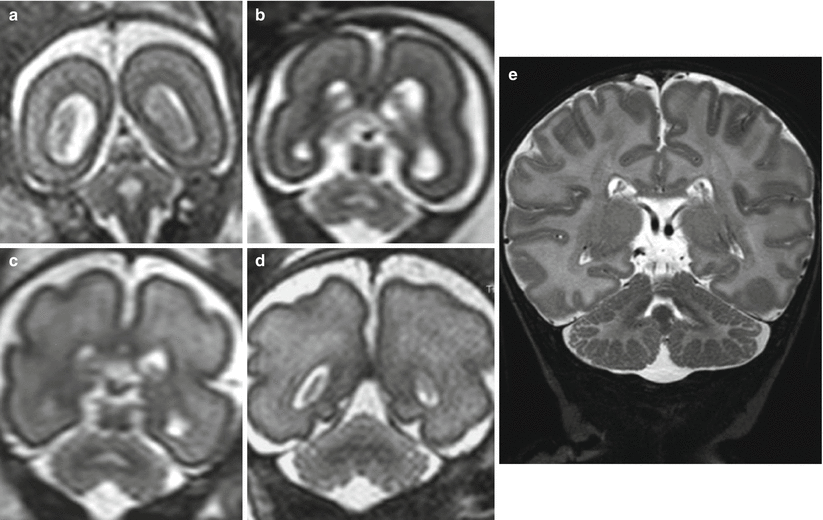
Fig. 3.5
Cerebellar anatomy. Progressive development of cerebellar hemispheres on in vivo fetal MR. Coronal section at 20 (a), 22 (b), 27 (c), and 30 (d) GW, and comparison with normal coronal anatomy on a T2-weighted image in a normal neonate (e)
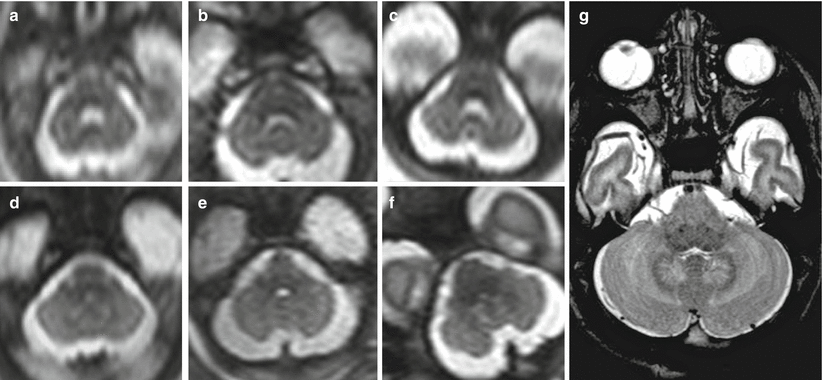
Fig. 3.6
Cerebellar anatomy. Progressive development of cerebellar hemispheres on in vivo fetal MR. Axial section at 19 (a), 21 (b), 22 (c), 25 (d), 27 (e), and 30 (f) GW and comparison with normal axial anatomy on a T2-weighted image in a normal neonate (g)
3.2 Classification of Posterior Fossa Malformations
Posterior fossa malformations have been classified according to embryological [7], etiological [8], or anatomical [9–11] criteria. The morphological–anatomical approach is varied between different authors. Tortori-Donati classified posterior fossa malformations as cystic and non-cystic [10], where cystic malformations are those associated with abnormal enlargement of posterior fossa CSF spaces. Non-cystic malformations are further divided into focal malformations involving the vermis or hemispheres or diffuse malformations involving both of them. Patel classified the posterior fossa malformation into two broad categories – as hypoplastic or dysplastic – and then further subcategorized them into focal or diffuse [9].
More recently Barkovich proposed a complex embryological and anatomical (developmental) classification initially referred to the brainstem malformations [12] and then to both brainstem and cerebellar malformations [13, 14] under the umbrella of midbrain–hindbrain malformations (MHB). Finally Doherty, Millen, and Barkovich [15] proposed a simplified version of the original classification of Barkovich [14] according to the degree of the involvement of brainstem or cerebellum (or midbrain). A short summary of this classification is reported in Table 3.1.
Table 3.1
MBHB malformations
Predominantly cerebellar malformations |
Dandy–Walker malformation |
Lissencephaly and cerebellar hypoplasia |
Other cerebellar hypoplasia |
Rhombencephalosynapsis |
Cerebellar hyperplasia |
Cerebellar dysplasia |
Cerebellar and brainstem malformations |
Pontocerebellar hypoplasia (PCH) |
Molar tooth malformation |
Cobblestone malformations |
Predominantly brainstem malformations |
Congenital cranial dysinnervation disorders |
Predominantly midbrain malformations |
This latter attempts to organize such complex malformation and it seems to be more practical and easier to follow in the clinical practice; however, it does not take into account the predominant cystic aspect of some classical malformations or “pseudo-malformation” such as the persistent Blake’s pouch cyst or the enlargement of the brainstem vermis angle, which are frequently encountered in prenatal diagnosis and are extensively reported described in prenatal ultrasound literature [16–20]. Moreover some diseases such as cerebellar hyperplasia, dysplasia, or congenital cranial dysinnervation disorders are at present far to be detectable by means of fetal MR. We consequently prefer a more descriptive classification of these abnormalities as they can be encountered during fetal life and perinatal period (Table 3.2).
Table 3.2
Posterior fossa malformation on fetal MR
Anomalies of CSF spaces |
Enlargement of CSF spaces and arachnoid cysts |
Persistent Blake’s pouch |
Increase of BV angle |
Predominantly cerebellar malformations |
Dandy–Walker malformation |
Cerebral and cerebellar malformation |
Rhombencephalosynapsis |
Cerebellar and brainstem malformations |
Pontocerebellar hypoplasia (PCH) |
Molar tooth malformation |
Predominantly brainstem/midbrain malformations? |
3.2.1 Anomalies of CSF Spaces
3.2.1.1 Enlargement of CSF Spaces and Arachnoid Cysts
In the evaluation of fetal posterior cranial fossa malformations, it could be useful to consider first of all the size and morphology of posterior fossa (dotted area A in Fig. 3.7) and of the vermis (area B in Fig. 3.7) and then their mutual relation.
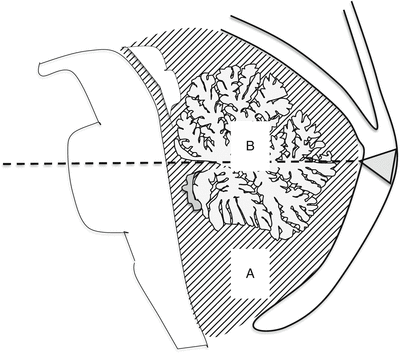
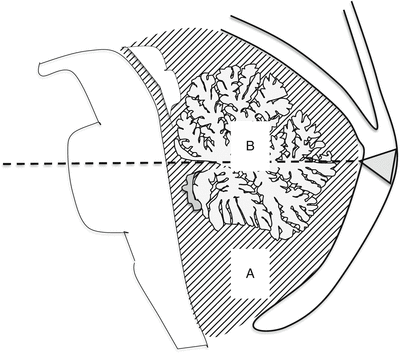
Fig. 3.7
Normal relationship between cerebellar vermis (b) and pericerebellar CSF spaces (a). The normal position of the torcular (gray triangle) is approximately at the end of an imaginary line that passes through the mid portion of the vermis
During fetal life, a normal or a slightly reduced vermis can be present within an enlarged posterior cranial fossa with a clear enlargement of the retrocerebellar spaces and/or cisterna magna.
This condition can be related to a simply enlargement of CSF spaces around vermis and cerebellar hemispheres or to the presence of an arachnoid cyst (Fig. 3.8).
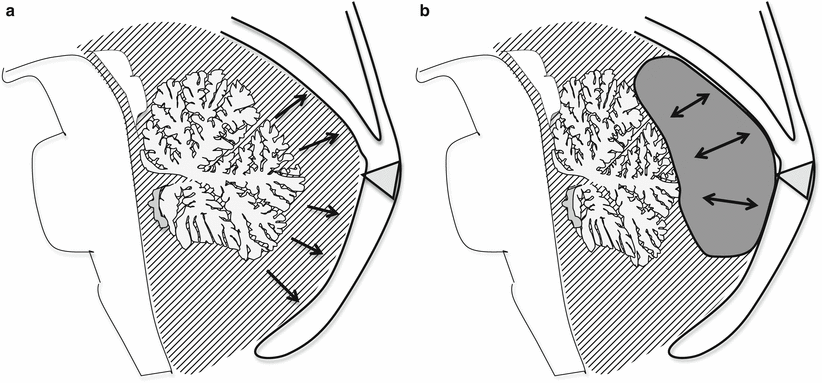
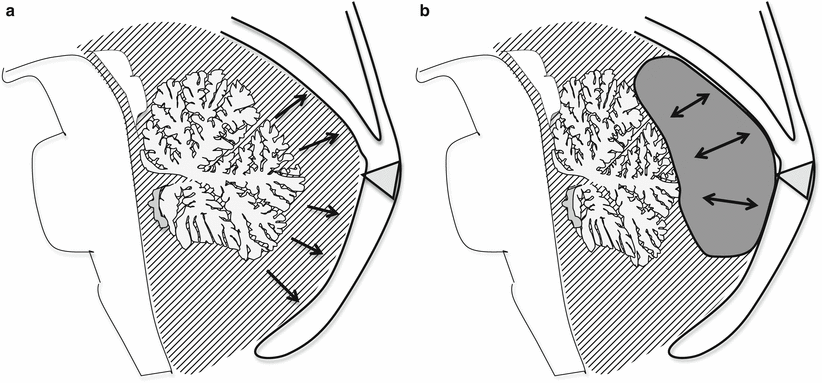
Fig. 3.8
In case of a simple enlargement of CSF space at the inferior or posterior aspect of the vermis, the relationship between vermis, pons, and torcular does not change, and no mass effect are visible (a). When a true arachnoid cyst occurs around the vermis or the cerebellum, a mass effect should be detectable (b), and in case of large cysts also the tentorium and the torcular could be pushed up
In the first case, no mass effect should be visible on the cerebellar vermis or hemispheres, and this condition can get better gradually during fetal life or even disappear (Fig. 3.9). In the simple enlargement of retrocerebellar spaces or cisterna magna, the CSF freely communicates with the surrounding CSF spaces and does not obstruct CSF circulation, and hence hydrocephalus must be absent [20].
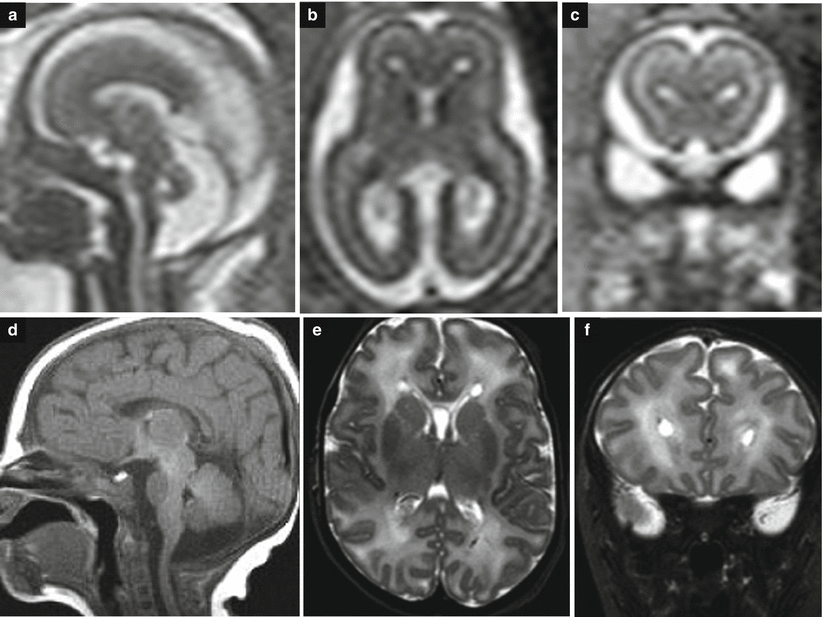
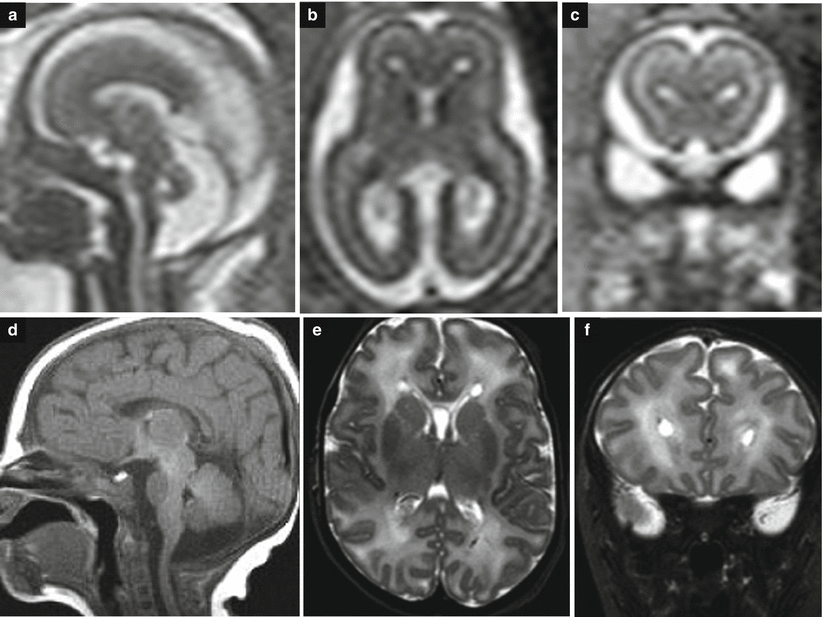
Fig. 3.9
Enlargement of fetal pericerebellar CSF spaces. At 21 GW pericerebellar spaces are enlarged, and the vermis is slightly hypoplastic (a); cerebral hemisphere are normal with small periventricular frontal cysts (b, c). At birth CSF spaces are normal as well as the cerebellar vermis (d); the periventricular cysts are still evident (e, f)
The diagnosis of an arachnoid cyst is usually possible only if it is accompanied with a mass effect on the vermis and cerebellar hemispheres (Fig. 3.10). The mass effect rarely decreases during fetal life, and more frequently an increase in size may be demonstrated during perinatal period. An arachnoid cyst may occur everywhere in the posterior fossa, and it may also result in progressive scalloping of the occipital bone [20].
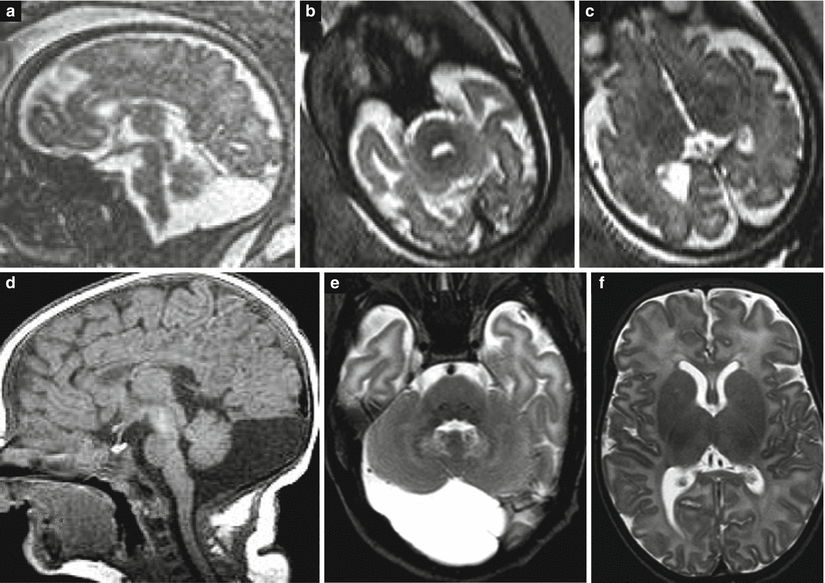
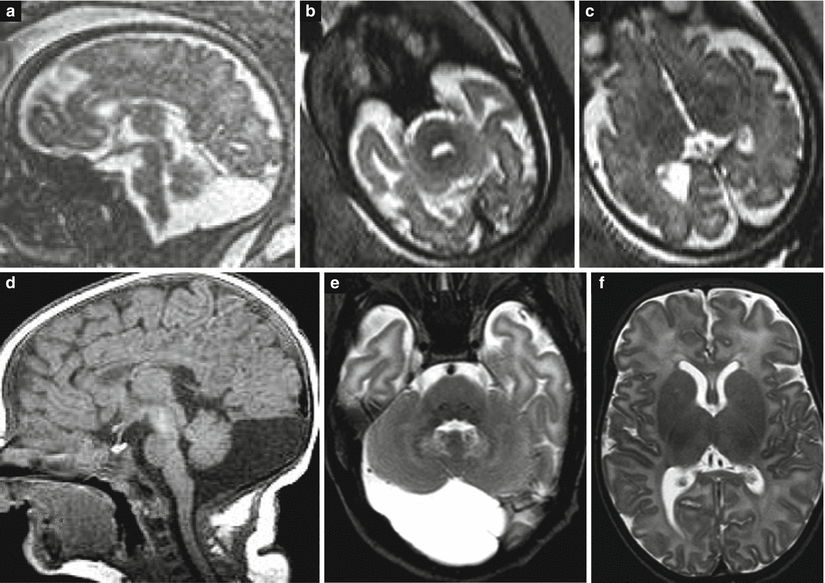
Fig. 3.10
Arachnoid cyst. At 34 GW (a–c) an enlargement of CSF spaces posterior to the vermis is evident; tentorium is pushed upward (a); these findings are confirmed after birth (d–f) where a slight mass effect is evident also on right cerebellar hemisphere; torcular is pushed not only upward (d) but also on the left side (e)
3.2.1.2 Persistent Blake’s Pouch
Blake’s pouch is an inferior protrusion of the fourth ventricle, which results from a fingerlike expansion of the posterior membranaceus area (PMA) [22, 23]. PMA is a transient structure which initially does not communicate with the subarachnoid space but that eventually becomes a permeable structure forming the foramen of Magendie. Persistence of the Blake’s pouch has been demonstrated into the fourth gestational month; a failure of permeabilization leads to a cystic CSF collection, inferior and posterior to the cerebellum, the Blake’s pouch cyst (BPC) (Fig. 3.11).
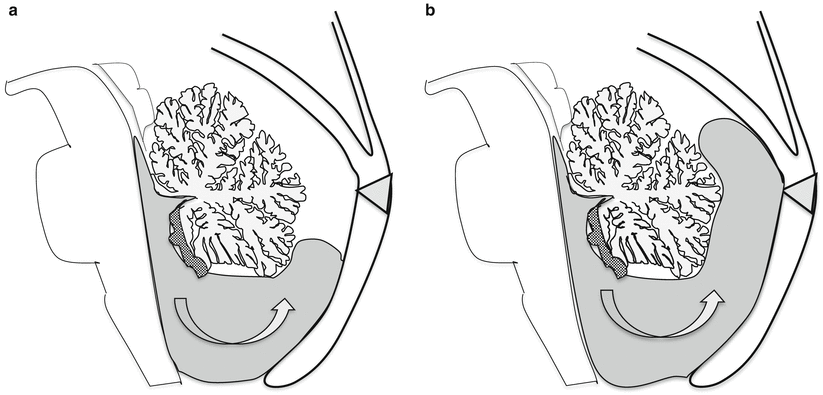
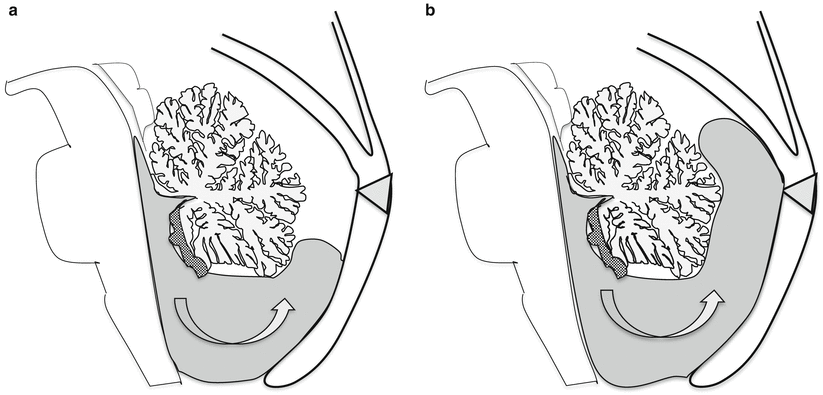
Fig. 3.11
Blake’s pouch cyst. A fingerlike expansion of the posterior membranaceus area (PMA) results in a cyst that grows inferiorly and posteriorly with respect to the cerebellar vermis (a, b) eventually exhibiting a posterior mass effect on it (b). The choroid plexus (dark grey) extends inferiorly along the superior wall of the cyst. The torcular is usually in a normal position
In BPC the cerebellar vermis usually appears normal or slightly counter clockwise rotated, and since by definition, the cyst is an extension of the inferior fourth ventricle, it is usually lined by ependymal cells [25], and the choroid plexus of the fourth ventricle usually extends inferiorly along the superior wall of the cyst [26]. Depending on the patency of the foramina of Luschka, persistent BPC can therefore present with hydrocephalus of varying severity. The timing of foramina of Luschka patency is still debated [24]; according to some authors, this event occurs around 14–17 weeks [27], but for others [28], they become patent at around 26 weeks. This can explain the reason why the majority of BPC detected at midgestation resolve spontaneously in the third trimester [24].
According to Paladini and colleagues [24], the three diagnostic criteria to make a diagnosis of BPC by means of fetal ultrasound are: (1) normal anatomy and size of the vermis, (2) mild/moderate anticlockwise rotation of the vermis, and (3) normal size of the cisterna magna, i.e., the mild to moderate counterclockwise vermis rotation is the main diagnostic clue. That is quite the same criteria for a presumptive diagnosis on fetal MRI together with the presence of a hydrocephalus (Fig. 3.12).
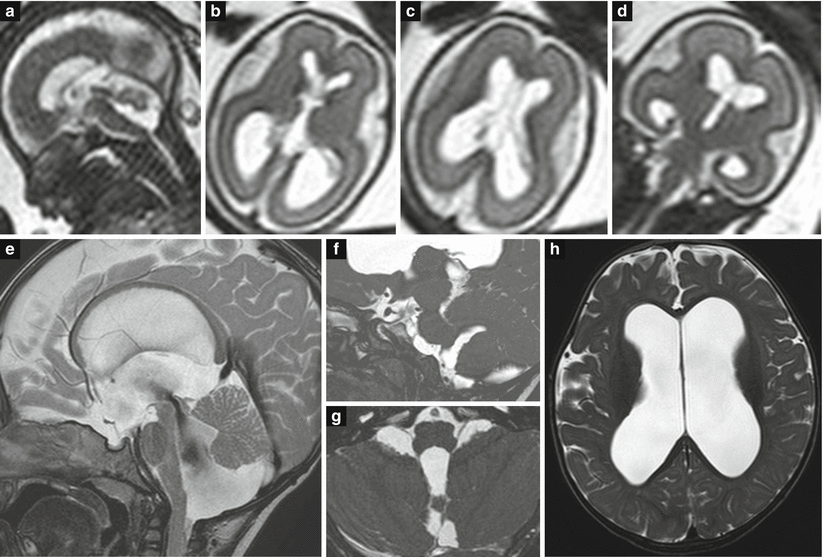
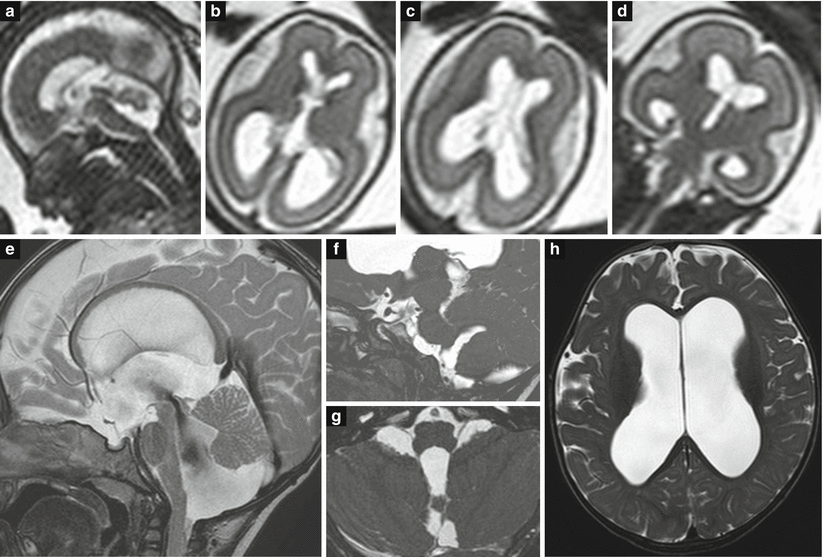
Fig. 3.12
Blake’s pouch cyst. Fetal MR at 24 GW (a–d) shows a slight counterclockwise rotation of the vermis with a moderate ventriculomegaly. After birth (e–h), the ventriculomegaly is further evident as well as the imperforate foramina of Magendie and Luschka where a thin membrane is detectable on high-resolution 3D T2-weighted images (f, g).
Volpe and colleagues measured the entity of the anticlockwise vermian rotation in a group of 12 fetuses with BPC through the evaluation of brainstem–vermian (BV) angle and demonstrated that it was comprised between 19° and 26°, whereas in the group of 80 normal control BV angle was comprised between 4° and 17° [21].
At birth the BPC is usually always associated with some degree of hydrocephalus, the vermis is slightly still rotated, and the persistent cyst can be detectable with high-resolution 3D steady-state free precession techniques. In this case the imperforated foramina of Luschka can be as well detected (Fig. 3.12). A surgical perforation of the cyst can be needed to resolve the hydrocephalus [29].
Being a simple cyst due to imperforation of Magendie (and Luschka) foramen with a normal cerebellum and a normal brain, the presence of BPC should be associated with a normal development; however an apparently increased risk of associated anomalies, including congenital heart disease, trisomy 21 [29], and Werdnig–Hoffman disease, was reported [30].
3.2.1.3 Increase of BV Angle
During midgestation, the presence of an increase of the BV angle is not a rare finding reported at US and MR. As previously discussed, this could be often simply due to a not yet perforated PMA; however other causes such as vermian hypoplasia or dysplasia must be also considered [31]. Some authors associated this aspect to an anatomical condition defined as inferior vermian hypoplasia [32, 33]. Even when this aspect was confirmed after birth [32], we still prefer the more generic term of vermian hypoplasia in order to define a “small” vermis on fetal MR at midgestation. It is virtually impossible at this time to precisely identify all the nine cerebellar lobules and sometimes also the anterior and posterior lobes. A true inferior vermian hypoplasia (hypoplasia of the posterior lobe or of some lobules of the posterior lobe) can usually be reliably detected only in the last part of gestation and obviously after birth.
According to Volpe and colleagues, BV measured by means of US allows not only to differentiate normal fetuses from fetuses with BPC but also to identify fetuses with vermian hypoplasia and Dandy–Walker malformation (DWM) [21]. The seven fetuses with vermian hypoplasia of their series show a range of BV between 24° and 40°, whereas the range in fetuses with DWM is between 45° and 112°.
On other words the greater the BV degree, the higher the probability of vermian hypoplasia (Fig. 3.13). Similarly to BPC, an increase of BV can be transient during pregnancy or still evident after birth (Fig. 3.14). At present there are no clear prognostic indices that may help to differentiate these two entities during midgestation. However rather than the simple value of BV angle, it seems more important the size of vermis and of course its morphology (Figs. 3.15, 3.16, and 3.17).
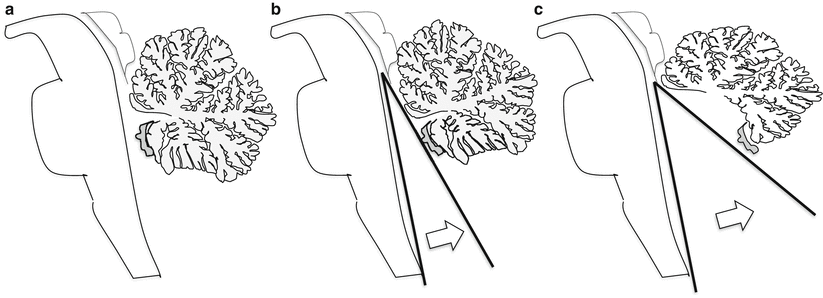
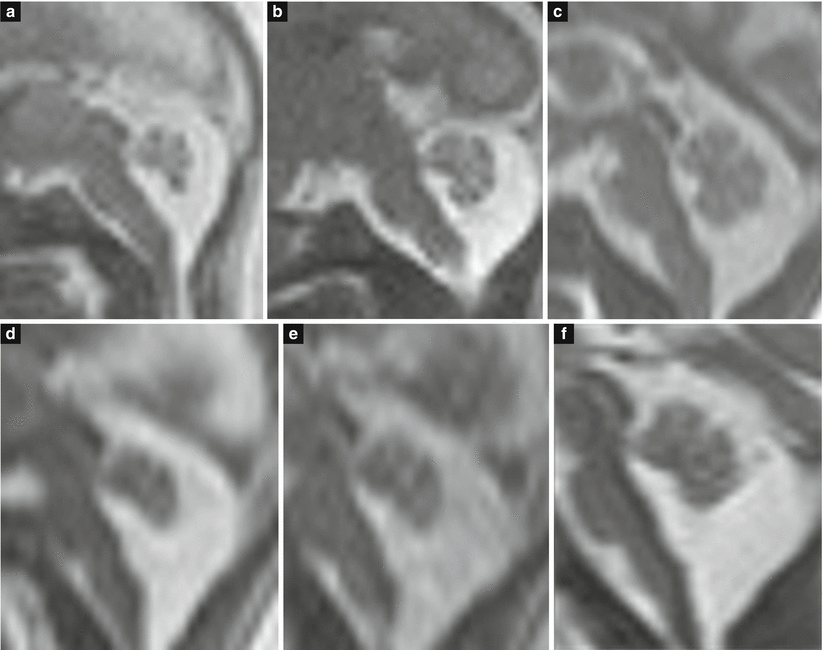
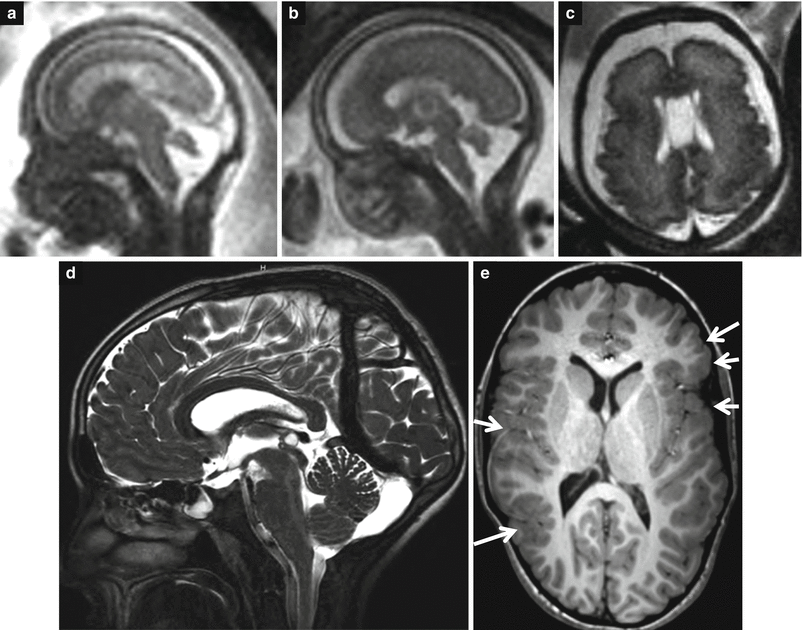
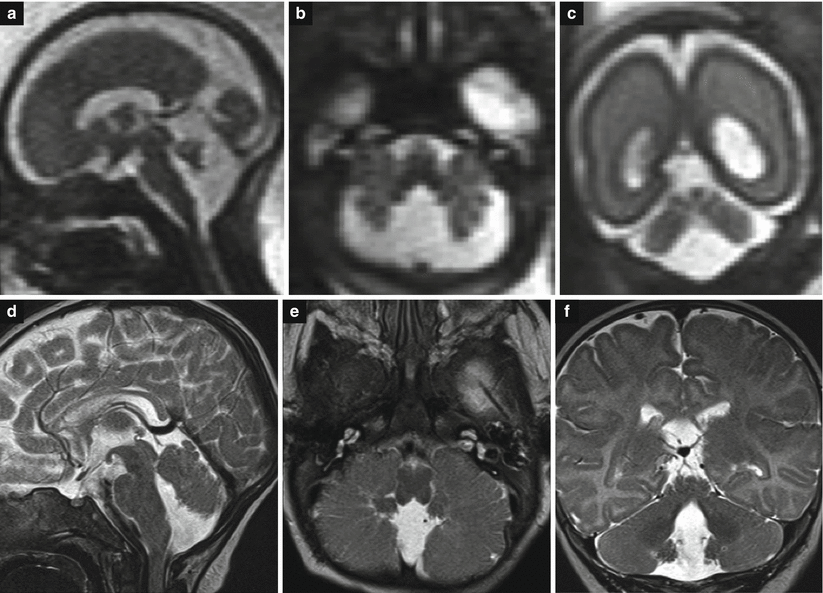
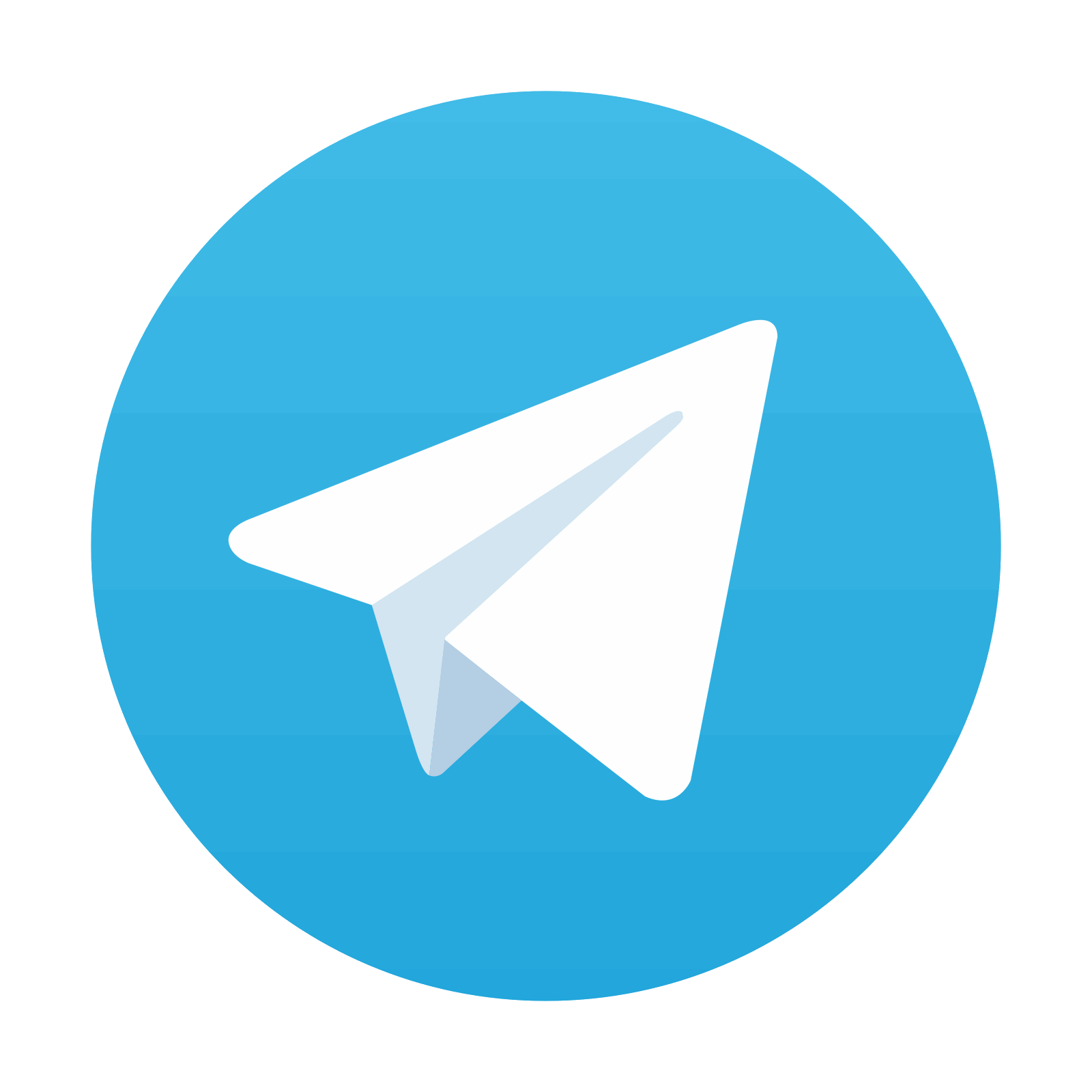
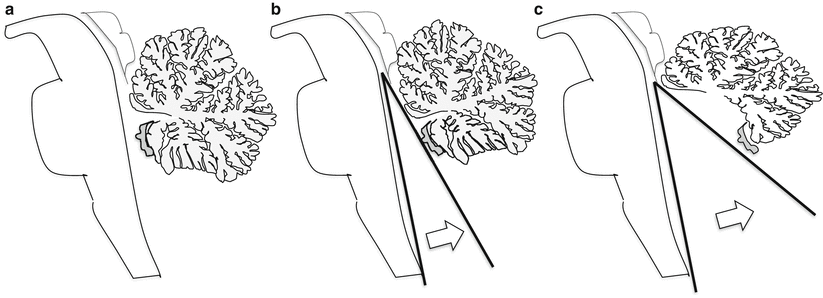
Fig. 3.13
Increase of brainstem–ventricular (BV) angle. The BV angle is the angle interposed between a line drawn tangentially to the dorsal aspect of the brainstem and a second line drawn tangentially to the ventral contour of the cerebellar vermis. Normal values should be less than 10° (a), but at midgestation values between 10° and 40° may be encountered (b, c). When BV angle increases close to 40°, the probability of some vermis hypoplasia increases
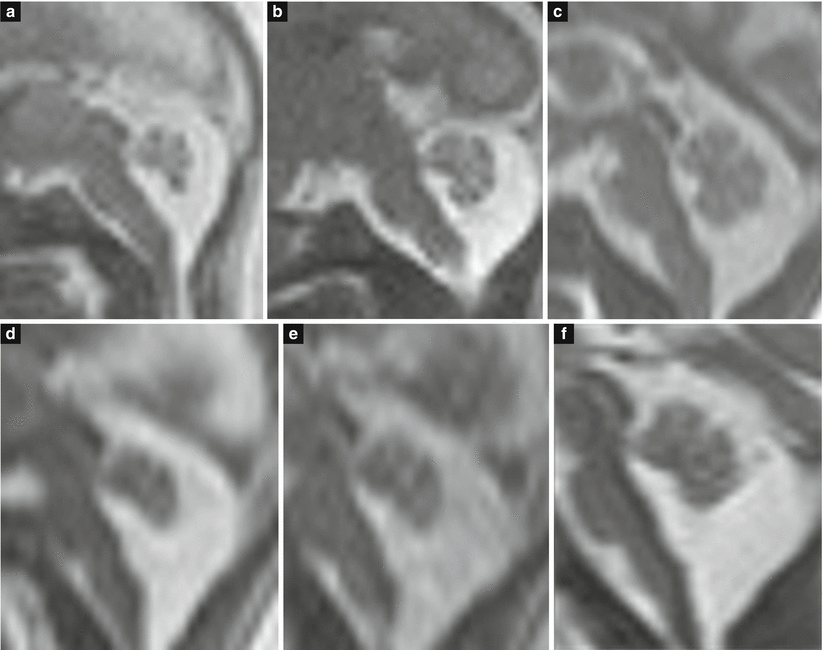
Fig. 3.14
Increase of brainstem–ventricular (BV) angle. The evolution (and the prognosis) of an increased BV angle at midgestation is largely unpredictable: in the superior row, the angle progressively decreases from the first study at 20 GW (a) to the second at 24 GW (b) and the third at 29 GW (c); in the inferior row the angle remains unchanged between the three studies at 21 GW (d), 22 GW (e), and 29 (f)
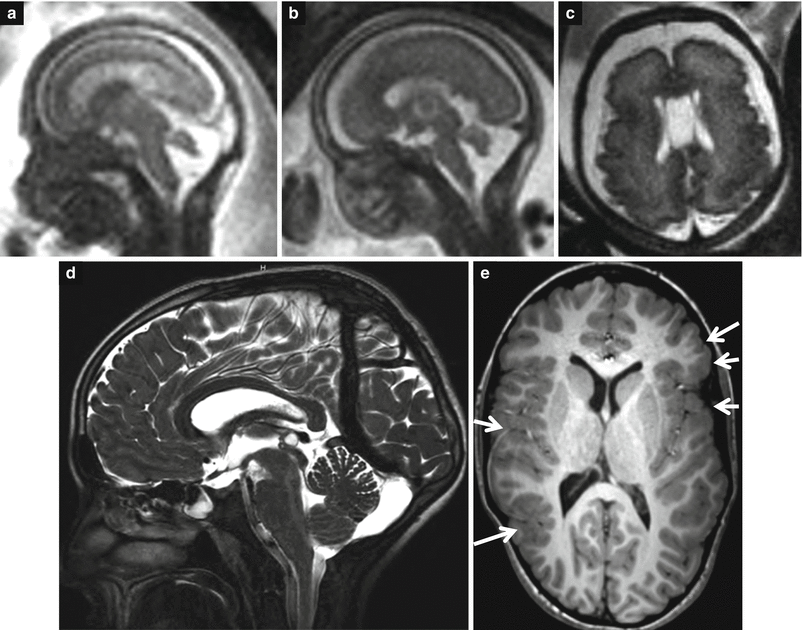
Fig. 3.15
Increase of BV angle and vermis hypoplasia. An increase of BV angle was detected at 19 GW (a) and slightly decreased after 2 weeks (b). At 32 GW some questionable cortical irregularities were detectable in the opercular areas bilaterally (c). After birth, a slight hypoplasia of the inferior vermis is still evident affecting mainly the last three lobules (not shown). In the MR control at 9 years, a poor differentiation between medulla oblongata and pons was detectable (d), and cortical irregularities compatible with polymicrogyria were confirmed (arrows in e). The girl had a moderate intellectual disability, epileptic seizures, and scaphocephaly; cardiac surgery was undertaken immediately after birth, and a genetic syndrome was suspected even still not identified
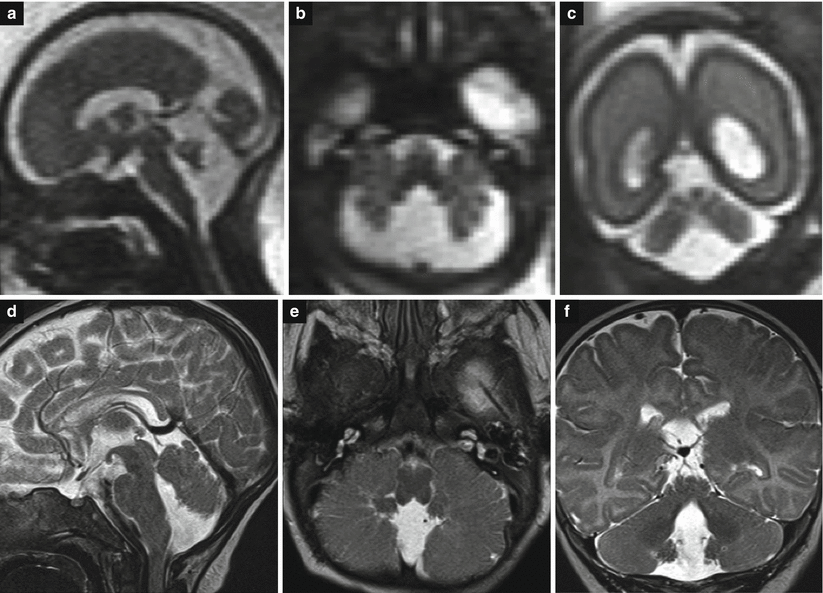
Fig. 3.16
Increase of BV angle. At 24 GW, a slight enlargement of BV angle is visible; however both vermis and cerebellar hemispheres seem to be dysmorphic and hypoplastic (a–c). These aspects were confirmed after birth where the cerebellum shows a clear dysplastic appearance with abnormally radially oriented folia on axial view (d–f)
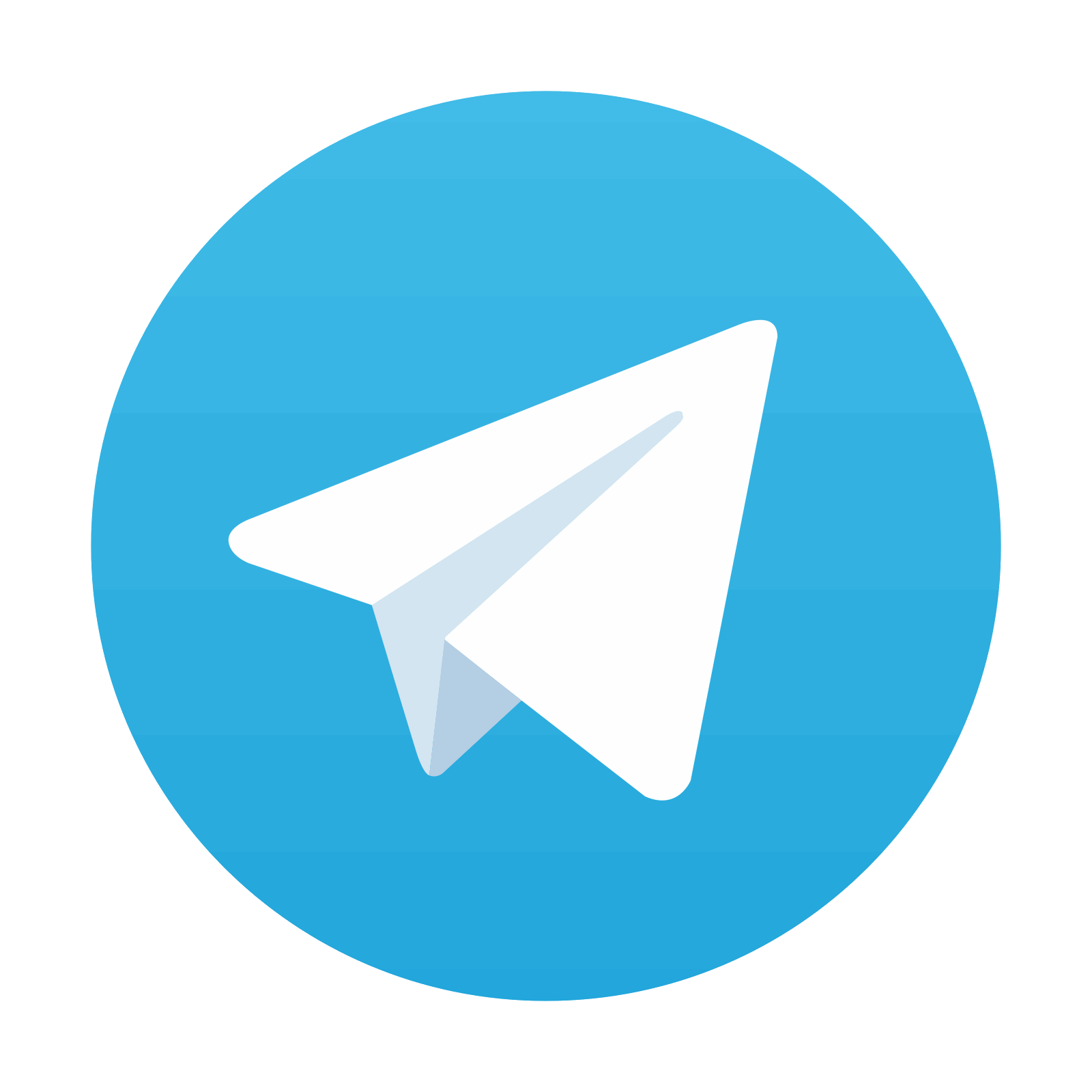
Stay updated, free articles. Join our Telegram channel

Full access? Get Clinical Tree
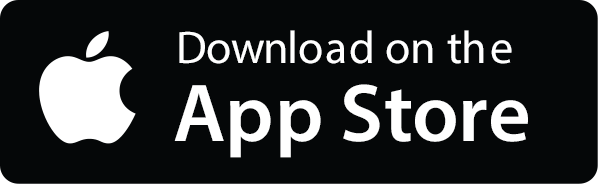
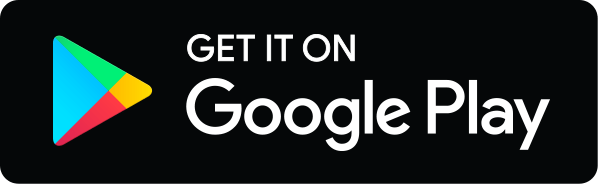
