Name of the artery [2]
Vascularization
Radicular (or radiculomeningeal) arteries (0.2–0.8 mm)
Nerve root, dura mater
Anterior radiculomedullary arteries (6–8) and the great radiculomedullary artery (0.5–1.0 mm)
Join the ASA (0.2–0.5 mm) and supply the two anterior thirds of the SC
Posterior radiculomedullary arteries (11–16) (0.1–0.4 mm)
Join the posterior and posterolateral spinal arteries and supply the posterior third of the SC
The junction of the anterior radiculomedullary artery with the ASA forms the characteristic hairpin configuration, located on the midline, identified on high-resolution DSA or CTA coronal reconstruction. The posterior radiculomedullary artery also gives this hairpin turn, but the junction with the PSA is off the midline.
The metameric supplementation of the ASA and PSA is not regular. Cervicothoracic, midthoracic, and thoracolumbar regions present anatomic variations of the SC vasculature (Table 2). At the upper thoracic level, a dominant radiculomedullary artery, described by von Haller in 1754, exists most of the time. This artery was observed in 86 % of patients with conventional angiography [4].
The great radiculomedullary artery (GRA) or Adamkiewicz artery, described by Adamkiewicz, originates from the segmental (intercostal or lumbar) arteries [5]. The course and morphology of the GRA are described as stable: in 70 % of cases, the GRA originates on the left side, frequently at the T8-L1 vertebral level [6]. Adamkiewicz described three anatomical types of the spinal vascular system [5]. Firstly, the ASA presents an uninterrupted course from the cervical portion to the lumbar level, with supplementation coming from intercostal arteries and the GRA. The second system presents the same configuration, but the ASA is poorly developed. In the third one, the ASA is interrupted, mostly at the thoracic level. The second and third systems present the highest risk of SC ischemia after clamping radicular arteries and consequently the GRA. Biglioli et al. reported an anatomic study on 51 adult cadavers without aortic disease, showing continuity between the vertebral artery, the anterior spinal artery, and the GRA [7]. This result supports that the sacrifice of segmental arteries during surgery or endovascular treatment of aortic disease may be justified without the risk of SC injury [8, 9]. However, this anatomic continuity may become a dangerous stealing pathway for the SC, explaining paraplegia occurring after surgery when prolonged cross-clamping of the aorta is performed [7].
Venous Drainage
Understanding the extensive anastomosing venous channels draining the SC and the spine is mandatory before a surgical or endovascular approach of spinal vascular malformations (SVM). In fact, abnormal visibility of the spinal veins is one of the main signs of arteriovenous fistula and can be identified either on CTA or DSA. Dilatation of the veins will motivate the radiologist to find the pathological communication between the artery and the vein: for that purpose, DSA remains the most sensible imaging modality.
One of the major characteristics of the spinal venous system is its variability, described as being greater than that of the arterial system [1]. The venous system is composed of an intrinsic and extrinsic system:
The intrinsic veins are divided into sulcal and radial veins.
The extrinsic or superficial venous system is composed of anterior and posterior spinal veins.
The anterior median spinal vein (diameter 0.4–1.5 mm) [2] runs with the ASA and continues to the filum terminale (vein of the filum terminale).
One posterior median, the greatest spinal vein, is accompanied by two posterolateral veins, located next to the posterolateral spinal arteries.
The extrinsic network system is in contact with the spinal pia matter. Spinal veins are characterized by a high anatomical variability: longitudinal veins can be replaced by a secondary network. Spinal veins drain into the anterior (numbers 8–20) [10] and posterior (numbers 5–10) [2] radiculomedullary veins that drain into the paravertebral and intervertebral plexuses. These venous plexuses drain in the segmental veins draining into the ascending lumbar veins, azygos system, and the pelvic venous plexuses. At the thoracolumbar level, the great anterior radiculomedullary vein (GRV; diameter 1.5–2.0 mm) may be mistaken for the GRA, but the angle of the junction of the GRV with the median vein is described as more obtuse than the hairpin configuration of the artery [11] (Fig. 1).
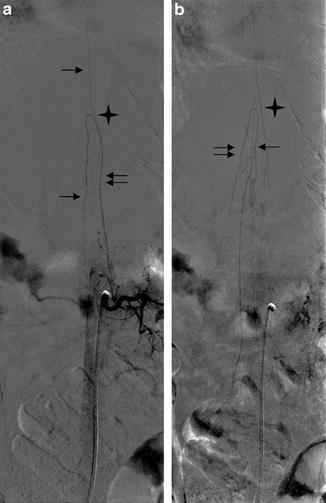
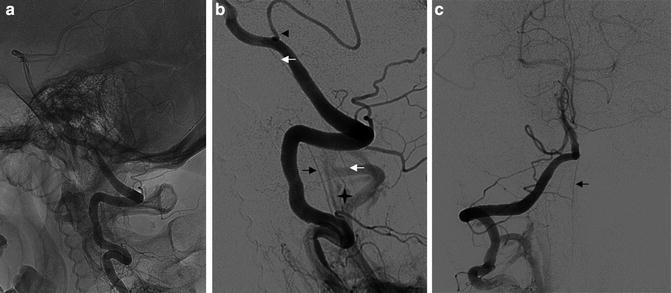
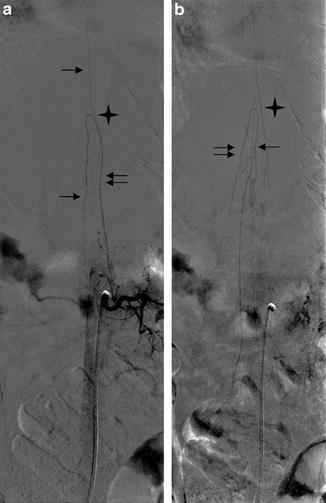
Fig. 1
DSA, AP view. Fifty-year-old man, suspicion of arteriovenous fistula. Injection into the L2 left lumbar artery: opacification at the arterial phase (a) and venous phase (b). (a) The great radiculomedullary artery (GRA) (double arrow), its hairpin turn (star), and the anterior spinal artery (ASA) (arrow) are opacified. (b) The great radiculomedullary vein (GRV) (double arrow), the hairpin turn (star), and the spinal vein (arrow) can present the same aspect as the arterial distribution
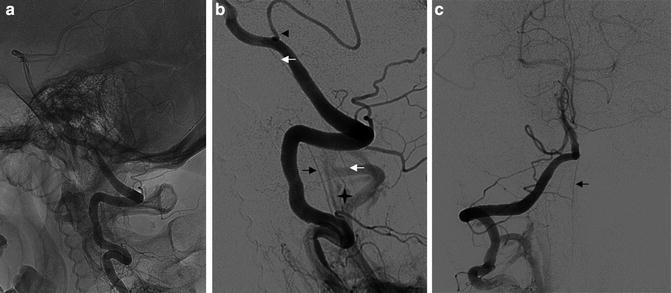
Fig. 2
DSA, lateral view (a, b) and AP view (c). Fifty-five-year-old man presenting a renal tumor cervical spine metastasis. Injection in the right vertebral artery. The anterior spinal artery (ASA) (black arrow) takes its origin at the level of V3, and one posterior spinal artery (PSA) (white arrow) comes from V4 at the level of the posterior cerebellar artery (PICA) (arrow head). The star shows the space occupied by the spinal cord, between the ASA and the PSA
The radiculomedullary veins communicate with the epidural venous plexus, into which the intradural veins are draining. Intradural and epidural veins are separated by valves at the level of the dura. Groen et al. [12] differentiate three levels of intercommunicating veins:
The internal vertebral venous plexus, formed by intradural and epidural veins, communicating with the intracranial venous system and draining in the external vertebral veins
The external vertebral venous plexus, located around the vertebra
The basivertebral veins, within the vertebra
Table 2
Main feeders of the SC blood supply at the different spine levels
Anterior feeders (for ASA) | Posterior feeders (for PSA) | Main feeder and particularity | |
---|---|---|---|
Cervical region | Intracranial section of the vertebral arteries (Fig. 2) | Vertebral artery (Fig. 2) | Artery of the cervical enlargement (level C5-C6) arising from the vertebral artery |
PICA | Radiculomedullary arteries arising from the deep and ascending cervical arteries and the vertebral artery | ||
Variation: anastomoses with the occipital and ascending pharyngeal artery (external carotid artery). Artery of the cervical enlargement can originate from the costocervical and thyrocervical trunk. Duplication of the anterior spinal artery is possible | |||
Thoracic region | Supreme and posterior intercostal arteries | Two dorsal feeders entering the spine above and below the GRA | One radiculomedullary artery (level T4-T5) arising from the intercostal artery |
Variation: two (48 %) or three (7 %) anterior radiculospinal artery [13] | |||
Discontinuity of the ASA is possible | |||
Lumbar region | Lumbar arteries | Artery of the lumbar enlargement | |
Anastomosis: at the level of the conus medullaris, connection of the anterior and posterior spinal arteries forming a basket shape anastomotic network | |||
Variation: duplication of the radiculomedullary artery | |||
Sacral region | Lateral sacral artery and iliolumbar artery from the internal iliac artery | Variation: spinal arteries caudal to the aortic bifurcation (rare) | |
Medial sacral artery |
Arteriography and CT Angiography of the Vasculature of the SC: Techniques, Results, Advantages, and Disadvantages
Arteriography
Technique
Spinal DSA can be performed without general anesthesia, usually reserved for children and patients who cannot cooperate or lie flat. The pain induced by a tumoral vertebral lesion has to be evaluated during the consultation with the radiologist and the anesthesiologist: in fact, a painful lesion can motivate the sedation of the patient, as the patient may present difficulties to remain immobile during the image acquisition. Most of the time, local anesthesia with intravenous midazolam and fentanyl is enough. Drugs are injected for the comfort of the patients, as they will be asked to hold their breath during the procedure to decrease artifacts. After local anesthesia, a 4 or 5 F sheet introducer is placed in the femoral artery. Catheterization of segmental arteries is performed from the higher to the lower level, mostly with a Cobra C2 (Terumo) or a Simmons 1 (Terumo) catheter. When the ostium of a segmental artery is catheterized, manual injection of contrast material is performed. To catheterize the lower ostium, the radiologist has to gently retrieve the catheter without turning it until he gets a new stabilization, considering the ostia of the segmental arteries as ladder rungs. Hence, it is recommended to begin the catheterization of segmental arteries from one side before doing the other side.
Bone relief of the spine is essential to get a good projection of the spinal artery: the spinous process has to project between the pedicles on the anteroposterior (AP) view. The GRA is well recognized thanks to its hairpin configuration that projects on the midline, at the level of the junction with the ASA. If the ASA mostly presents as a straight vessel situated on the midline in young patients (Fig. 3), it can present loops in older patients (Fig. 4). The radiologist has to be precise on the level of the segmental artery giving rise to the GRA. The posterolateral radiculomedullary artery presents a smaller course with a lateral projection. During the injection of a segmental artery, a blush of the vertebra is clearly identified (Fig. 3). Difficulties to catheterize segmental arteries can be explained by an agenesia (Fig. 5) or thrombus of the artery in the case of an atheromatous aorta.
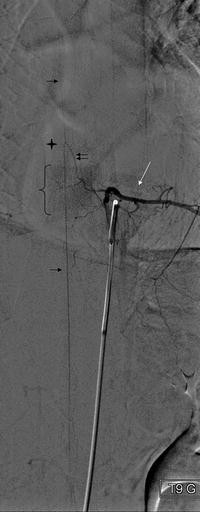
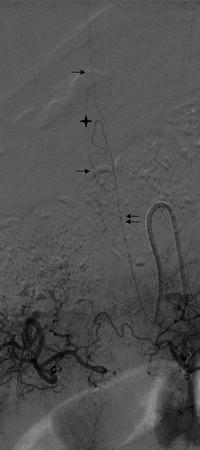
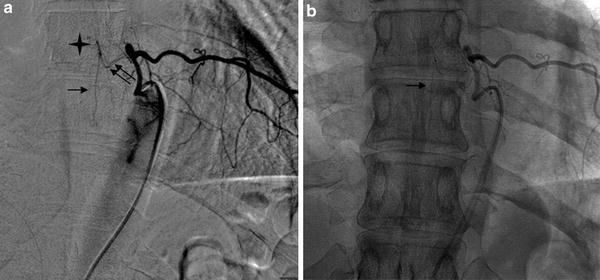
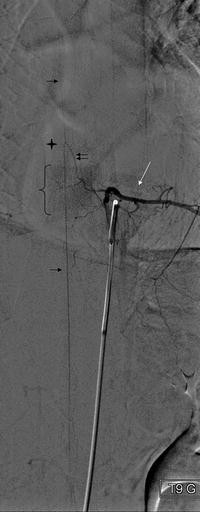
Fig. 3
DSA, AP view. Thirty-five-year-old woman, suspicion of medullary arteriovenous fistula. The GRA (double arrow) is visible after injection into the left T9 intercostal artery. The hairpin turn (star) and the ASA (arrow) run on the midline. The intercostal artery presents a short ascension and divides in a ventral branch and a dorso-spinal branch. Enhancement of the vertebra body (brace) and of the paraspinal muscles (white arrow) is clearly identified
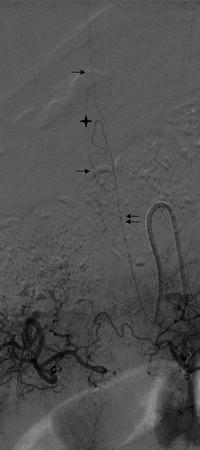
Fig. 4
DSA, AP view. Seventy-year-old patient, suspicion of arteriovenous fistula. Catheterization of the left T10 intercostal artery. GRA (double arrow), the hairpin (star), and the ASA (arrow) are clearly identified. Note the loops of the ASA, on the contrary to case described on Fig. 3
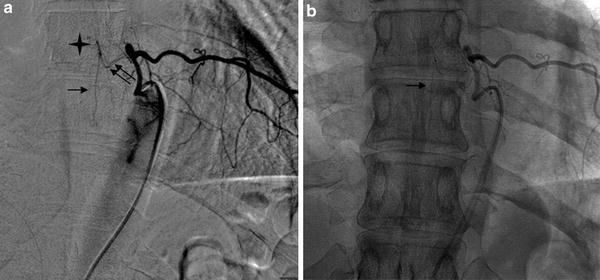
Fig. 5
DSA, AP view with (a) and without (b) subtraction. Thirty-three-year-old woman. Preoperative imaging for an intercostal tumor. Injection into the left T8 intercostal artery. The posterior radiculomedullary artery (double arrow) is opacified. This artery presents an angulation and a hairpin configuration (star) as the ASA, but is localized off the midline (image b). The PSA is visible (arrow)
Vessels that may supply the spinal arteries have to be known and explored according to the level of the spinal lesion (Table 3). Except for the diagnosis of arteriovenous fistula, which may require a global visualization of the spine and SC blood supply, the arterial exploration of a spinal lesion concerns the level of the lesion and one or two levels above and below.
Table 3
Arteries supplying the spine and potentially the SC depending on regions
Upper cervical spine | Vertebral artery |
Ascending cervical artery | |
Deep cervical artery | |
Occipital arterya | |
Cervicothoracic spine | Vertebral artery |
Thyrocervical and costocervical trunks | |
Supreme intercostal artery | |
Intercostal arteries | |
Bronchial arterya | |
Lumbosacral spine | Lumbar arteries |
Iliolumbar artery | |
Medial sacral artery | |
Lateral sacral artery |
Advantages and Disadvantages
DSA presents a higher spatial and temporal resolution than CTA and MRA. Moreover, angiography may be the first step before an endovascular treatment such as embolization of an arteriovenous fistula or preoperative embolization of vertebral tumors. Concerning the vascular disease of the spine, DSA remains the best diagnostic imaging tool for the anatomic comprehension and therapeutic planification. Even if new generation CT scanners can improve the anatomic comprehension of arteriovenous fistula, cases reporting the feasibility of CT scanner in dynamic assessment of spinal fistula remain anecdotal [14].
DSA presents the main disadvantage of being invasive, time consuming, complicated, and consequently associated with a risk of SC ischemia [15]. Moreover, DSA exposes patients to potentially high doses of radiation and contrast. The risk of paraplegia after arteriography was estimated to be 1.2 % in a series of 480 patients, which is significant compared with the 3–5 % occurrence of paraplegia after aortic surgery in a surgical series [16]. However, these high rates of complications are described for patients suffering from dissection or aneurysm of the aorta. Other retrospective case series reported a minimal rate of neurologic complications and emphasized the important role that spinal DSA still presents for the diagnosis of spinal vascular malformations or preoperative spine surgery imaging (Table 4) [17, 18]. Hence, with the use of new endovascular technologies and nonionic contrast agents, it can be assumed that modern spinal DSA carries a very low risk of neurologic and systemic complications [18].
Table 4
Case series of spinal DSA
Study | Number of patient | Indication | Localization of the GRA (%) | Complications (%) |
---|---|---|---|---|
Forbes 1988 [19] | 96 | AVM (88), tumor (8) | NA | 3.7 systemic |
2.2 neurologic | ||||
Savader 1993 [20] | 50 | Thoracoabdominal aortic aneurysm | NA | 4.6 |
Heinemann 1998 [21] | 46 | Aneurysm and chronic dissection | 65 | 0 |
Kieffer 2002 [15] | 487 | Aortic aneurysm | 86 | 0.8 neurologic |
0.4 renal failure | ||||
0.6 groin complication | ||||
Williams 2004 [14] | 151 | Aortic aneurysm and dissection | 43 | 0 |
Chen 2011 [18] | 302 | Spinal vascular malformation (most common indication) | 97.4 | 1 systemic |
0.7 groin complication | ||||
Nair 2013 [17] | 228 | Spinal tumor embolization | NA | 0 |
Angio-CT
Technique
Many reports propose protocols of injection and technical points to visualize the segmental artery giving rise to the GRA. Many case series describe different times of injection, aortic triggering, and contrast agents for different aortic diseases. Detection rates of the GRA are variable, ranging from 18 % to 100 % [22–29] (Table 5). This variability may be attributable to the patient’s hemodynamic characteristics, the type of aortic disease explored, and technical factors such as imaging delay, volume, and flow of contrast medium. Authors tried to determine the optimal protocols for the detectability of the GRA. Nakyama et al. proposed a contrast medium injection using an imaging delay of 18 s after triggering and an iodine dose of 720 mg l/kg body weight [30]. Dual-phase CT angiography with a catheter inserted into the right atrium has been evaluated for preoperative visualization of GRA in patients with aortic aneurysms [31]. Results were promising, but the problem of the double dose of radiation and contrast medium remains (Table 5).
Some authors have evaluated the interest of multidetector-row CT angiography with intra-arterial contrast injection (IA-CTA) to depict the GRA. Authors reported very high percentages of visualization of the GRA and were able to precise the origin of the GRA in almost all cases [29, 32]. Only one study attempted to compare the sensitivity of CT angiography and conventional angiography in the visualization of the GRA before thoracoabdominal aorta repair for aneurysms or dissection [32]. The CT angiography was performed after IA (IA-CTA) injection of contrast, and a conventional angiography was done just after the CT. It was possible to visualize the GRA in 90 % with IA-CTA. Moreover, in 23 % of the patients, the GRA was visualized with the IA-CTA but not with conventional angiography.
New algorithms such as model-based iterative reconstruction (MBIR) have been proposed to improve the quality and spatial resolution [33]. Nishida et al. [34] reported a series of 34 patients presenting different aortic diseases with an improvement of the detectability of the GRA in comparison with CT reconstruction algorithms (adaptive statistical iterative reconstruction and filtered back projection). This improvement of delineation of the ASA has been reported in a short series of ten patients [35].
Ou et al. [36] reported a series of 40 children (mean age, 7.5 ± 5 years) explored with CT angiography for the assessment of the GRA. The GRA was visualized in 95 % of the children. One disadvantage of CT angiography is the artifacts generated by the proximity of bone structures. This problem may be encountered and overcome with MRI because the bone shows no signal.
Advantages and Disadvantages
CTA presents the advantage of being noninvasive in comparison with conventional arteriography. New generations of scanner allow very fast acquisition, with an excellent spatial resolution, which is necessary for the visualization of the vessels of the SC. Moreover, rates of detection of the GRA reported are extremely high, even if there is a publication bias and no comparison with arteriography most of the time. For aortic disease, CTA allows a precise visualization of an aneurysm or a dissection (extent, diameter, type of aneurysm, or dissection). CTA is logistically more accessible than MRI in most institutions. Moreover, the availability of the software Osirix, dedicated to DICOM image, allows the detection of the GRA with good agreement in comparison to the standard workstation [24].
However, CTA involves ionizing radiation, and the exploration of the abdominal cavity is associated with a relatively high effective dose of 20 mSv [37]. The larger the abdomen, the lower the contrast-to-noise ratio. In consequence, it is possible that the high rate of detection of the GRA, mostly reported in the Japanese population, is not the same with Caucasian patients.
It is difficult to find a dedicated timing for the visualization of the GRA (Table 5). High concentration of contrast is mandatory, but the difficulty is to determine the exact timing of the acquisition, as the enhancement of the GRA is visible for approximately 10 s. After that very precise temporal window, the image is polluted by venous drainage, which can be mistaken for the SC arterial supply. This drawback is more obvious in the case of a spinal vascular malformation.
Table 5
Main case series reporting the success rates of detection of the GRA and the technical aspects of injection with CTA
Study | N= | Indication | MDCT | Contrast material | Injection | Aortic luminal attenuation | GRA visualization (%) | Continuity GRA and aorta | |
---|---|---|---|---|---|---|---|---|---|
Zhao 2009 [25] | 99 | Thoracic aortic disease | 16-row | Iohexol 120–150 mL; 350 mgI/mL; 4–5 mL/s | IV | 358 ± 62 UH | 52 | NA | |
Clarençon 2013 [32] | 30 | Aneurysm and dissection | 16-row | Iodixanol 120 mL; 320 mg/mL; 15 Ml/S; | IA | NA | 90 | 84 | |
Nojiri 2007 [29] | 27 | Aneurysm (13) and dissection (14) | 4-row | Iomeprol 400; iopamidol 370 or iohexol 300; 100 ml;4–5 ml/s (A); 120 ml;7 ml/s (D) | IA | 4.5–7 s after start of inj | 100 | 90 for the detection of the level: 61.5 for the exact continuity | |
Ou 2007 [36] | 40 | Congenital disease of the aorta | 16-row | Iohexol 300; 1.5 ml/kg; 2.5–3 ml/s | IV | 85 UH | 95 | 95 | |
Uotani 2008 [22] | 32 | Aneurysm (21) and dissection (11) | 16-row | Iopamidol 370; 100 mL;5 mL/s | IV (15) and IA (17) | 140–160 UH | IACT:94.1 | 87.5 | |
IVCT:60 | 55.6 | ||||||||
Kudo 2003 [28] | 19 | Liver disease | 4-row | Iohexol 350; 100 mL;5 mL/s | IV | NA | 68 | NA | |
Nakayama 2008 [28] | 80 | Aneurysms | 64-row | Iohexol 540 mg/mL; 6 mL/s | IV | 150UH | 15 s | 56.3 | NA |
18 s | |||||||||
Iohexol 720 mg/mL; 6 mL/s | 21 s | ||||||||
18 s | |||||||||
Boll 2006 [38] | 100 | 40 | Iomeprol 400 mg/mL;80 mL; 3.5 mL/s | IV | 150 | 100 | 100 | ||
Utsunomiya 2008 [27] | 80 | Aneurysms | 64 | Iomeprol 300 mg/mL;100 mL; 5 mL/s | IV | 250 | 75 | 50 (best protocol) | |
350 mg/mL;100 mL; 5 mL/s | 80 | ||||||||
300 mg/mL;100 mL; 3.5 mL/s | 45 | ||||||||
350 mg/mL;100 mL; 3.5 mL/s | 50 | ||||||||
Yoshioka 2006 [27]a | 30 | Dissection (12) | 16-row | Iopamidol 370 mg/mL; 2 mL/kg; 3.5 mL/s | IV | 200 | 83 | 58 | |
TAA (18) | |||||||||
Yoshioka 2003 [39]a | 30 | Dissection (10) | 4-row | Iopamidol 370 mg/mL;2.5 mL/kg; 3 Ml/s | IV | 130 | 80 | 62.5 | |
TAA (20) | |||||||||
Nishida 2014 [34] | 34 | TAAA (6) | 64 | Iopamidol 370; 100 mL; 5 mL/s | IV | 250 | 90b | NA | |
Follow up TAAA surgical grafting (9), endovascular repair (15), chronic dissection (3), Takayashu (1) | |||||||||
Amako 2012 [31] | 110 | TAA and TAAA | 8 and 16-row | Iopamidol 370; 120 mL; 8 mL/s | IV (right atrium) | Two acquisitions: 20 and 55 s after the first injection | 100 | 90 | |
Takase 2002 [23] | 70 | TAA (20) | NA | Iopamidol 300; 100 mL; 3.5 mL/s | IV | 85 | 90 % | 31.7 | |
AAA (16) | |||||||||
Dissection (19) | |||||||||
Atherosclerosis (15) |
Spine and Spinal Cord Vascularization Imaging: Main Indications
The knowledge of the main arteries supplying the SC, in particular the GRA, is one of the major points of spinal surgical planning. The aim is to avoid neurologic complications, such as paraplegia, anesthesia, loss of bladder and bowel control, and sexual dysfunction, described after the ligature of the GRA or the segmental artery giving the GRA. Radiculomedullary arteries have to be clearly identified before an embolization. However, the interest of searching for a radiculomedullary artery before endovascular or surgical treatment of aortic disease or even before spine surgery continues to be a controversial subject. Indications of such preoperative imaging are discussed.
Spinal Surgery and Percutaneous Spinal Procedures
Concerning the vascular preoperative imaging of spinal tumors, selective catheterization of segmental arteries feeding the tumor allows the visualization of spinal arteries, which can be helpful for surgical planification. In these cases, the aim of DSA is to identify a radiculomedullary artery at the level concerned by the surgery or before a percutaneous treatment (Figs. 6 and 7). Koshino et al. showed that the GRA does not specially originate from big segmental artery and proposed that all the segmental arteries have to be preserved during surgery [6].
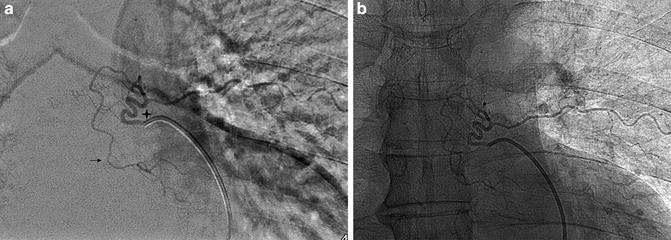
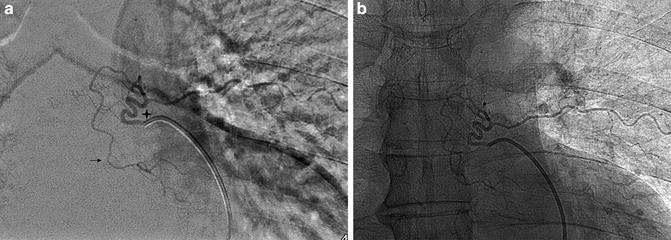
Fig. 6
DSA, AP view with (a) and without (b) subtraction. Catheterization of the left T6 intercostal artery. A longitudinal anastomosis coming from T6 supplies the level below (arrow). No left T5 intercostal artery was found for that patient
There is no interest to systematically search the GRA at levels far from the operative site. However, longitudinal anastomoses allow a new blood supply for the SC: hence, ablation of vertebras and segmental arteries giving rise to spinal artery is of course not necessarily associated with paraplegia. Murakami et al. reported their experience in total en bloc spondylectomy associated with an interruption of the GRA. Fifteen cases in which the GRA was described on DSA and ligated did not present alteration of their neurologic function. They concluded that surgeons are allowed to sacrifice up to three pairs of segmental arteries and that the presence of the GRA at the level of the surgery is not a contraindication for surgery (Fig. 7) [40].
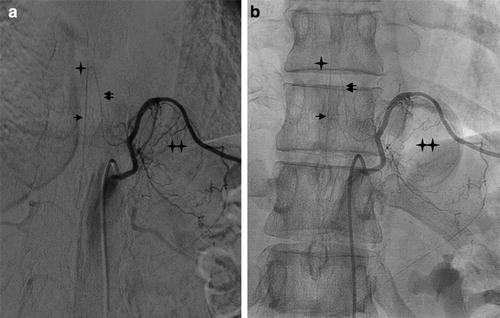
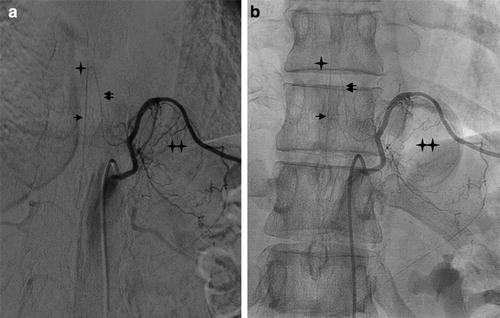
Fig. 7
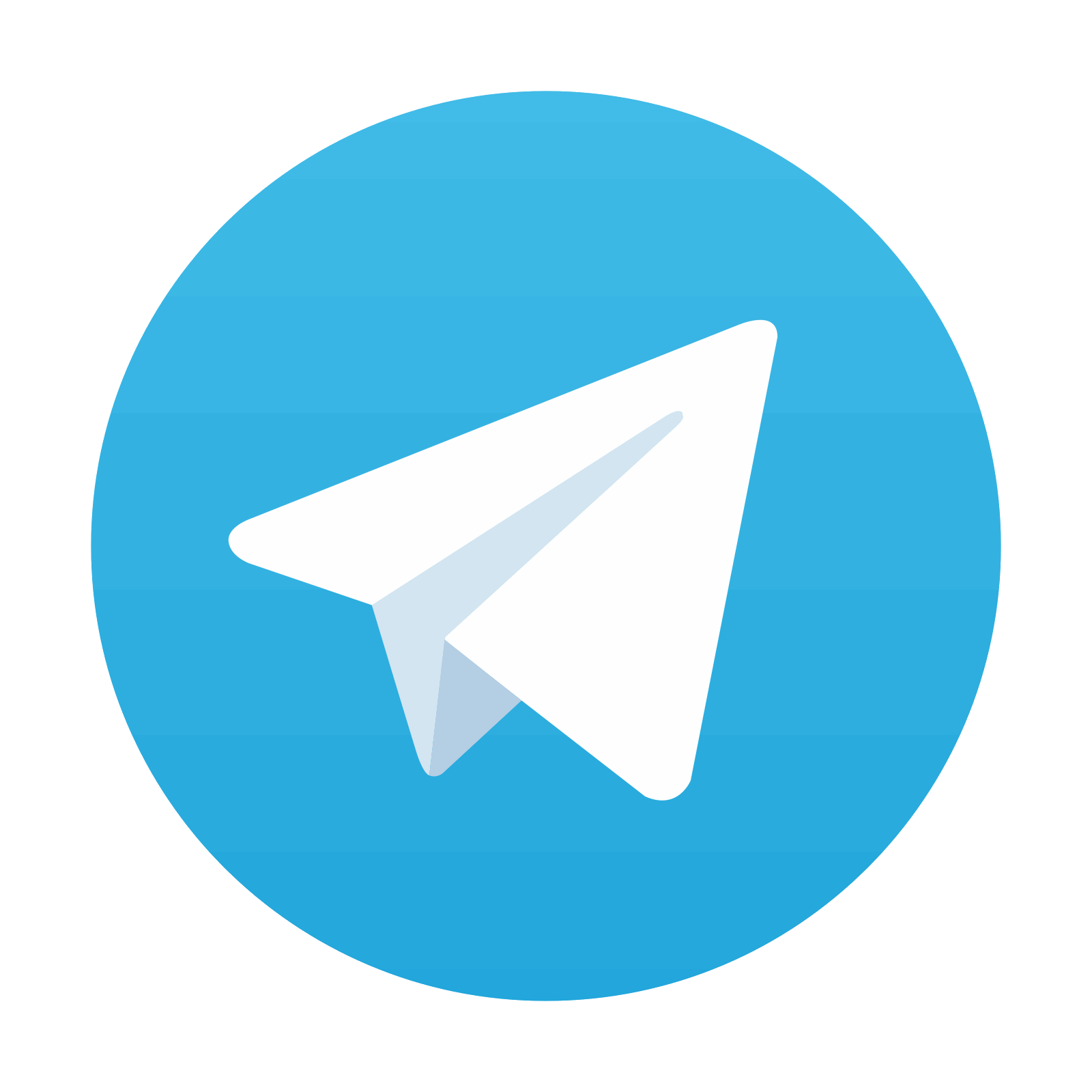
DSA, AP view with (a) and without (b) subtraction. Thirty-three-year-old woman presenting a schwannoma at the level of left T10 (double star). Injection into the left T10 intercostal artery. Because of the presence of the GRA (double arrow) coming at the level of the schwannoma, surgery was canceled, and a cryotherapy was performed, taking precautions not to injure the intercostal artery. Hairpin turn (star) and ASA (arrow) are identified. Note the mass effect on the intercostal artery which is upraised by the lesion
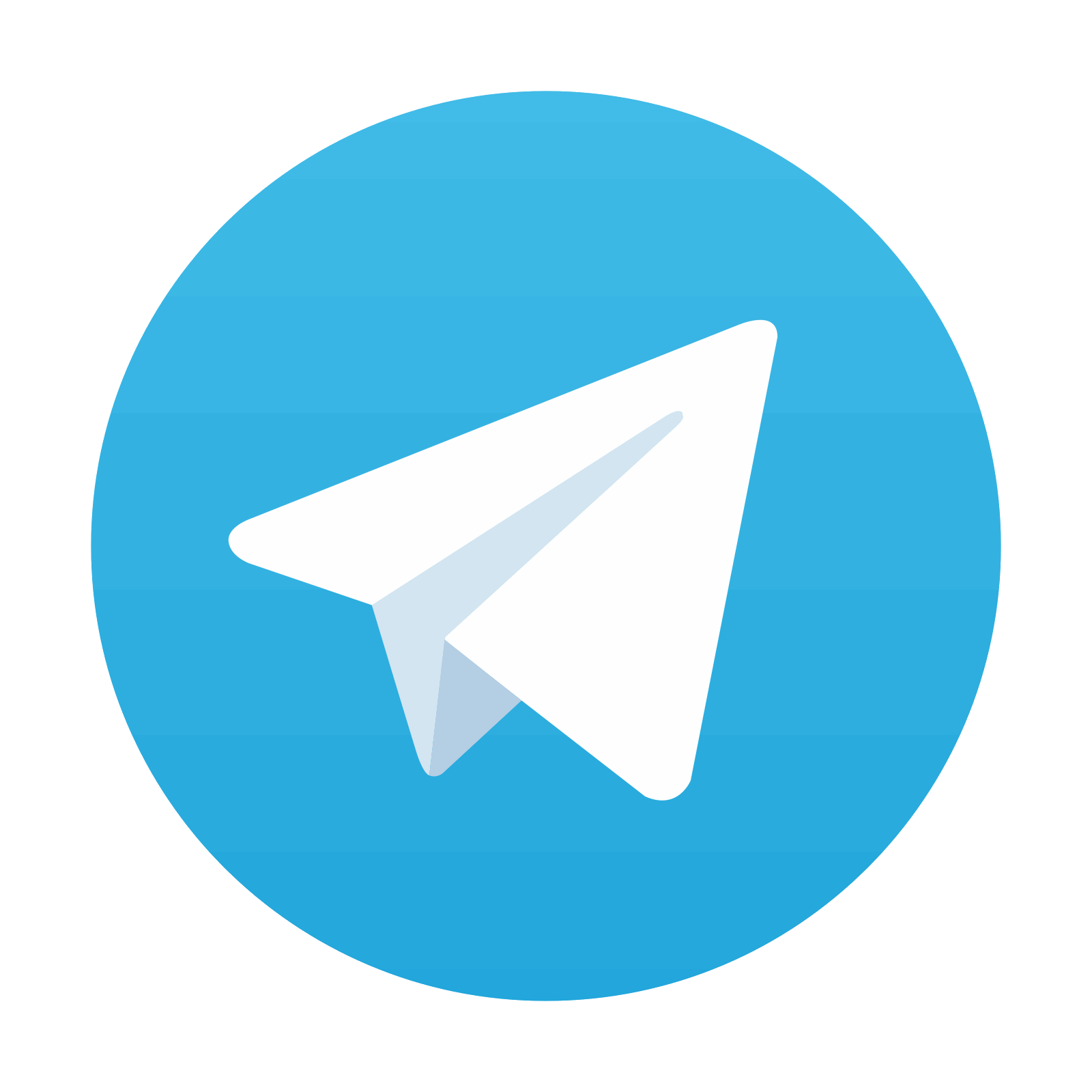
Stay updated, free articles. Join our Telegram channel

Full access? Get Clinical Tree
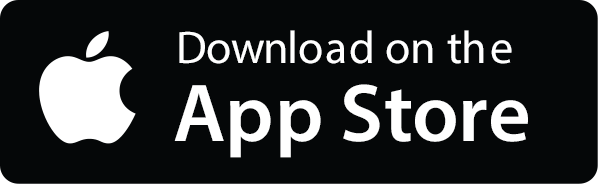
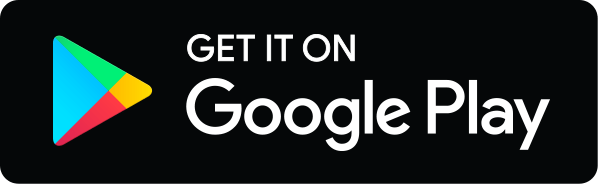
