10 The Cervical Spine Although imaging protocols of the cervical spine for specific indications can vary among institutions, standard MRI of the cervical spine for degenerative pathologies usually includes the following pulse sequences: • Sagittal T1-weighted SE • Sagittal T2-weighted FSE • Axial gradient-echo • Axial T2-weighted FSE A detailed discussion of all the imaging sequences used in the cervical spine is beyond the scope of this chapter; however, salient features of commonly used sequences are discussed below. T1-weighted images are useful in identifying fracture lines. Because they are sensitive to the presence of gadolinium contrast, they are also used for contrast-enhanced imaging, which is helpful in assessing neoplasms, infections, and the postoperative spine. Typically, fat-suppressed postgadolinium T1-weighted images are used to make lesions more conspicuous. T2-weighted images are sensitive to water (and thus edema) and are useful in identifying areas of potential pathology. However, care must be taken with regard to interpreting bone marrow edema because it may be seen with a variety of conditions, including infection, inflammation, trauma, and degeneration. Although edema may focus attention toward an abnormality, many of these conditions can coexist, so additional analysis is required before finalizing a conclusion. FSE is now routinely used to acquire T2-weighted images at speeds up to 64 times faster than conventional SE T2-weighted images. Sometimes the differentiation of fat, water, and lesions can be difficult, especially on T2-weighted FSE images, and therefore fat suppression is used to make these areas more conspicuous. This sequence can be obtained by applying a fat-suppression pulse to produce fat-suppressed T2-weighted images or by obtaining a STIR sequence. Visualizing edema is helpful in identifying ligamentous injuries, and such visualization is best achieved with STIR or fat-suppressed T2-weighted images. T2-weighted images are also most sensitive for evaluating the cord parenchyma for lesions and edema, which are seen as abnormally bright signal, although the sagittal orientation is subject to linear bright artifact within the cord (Gibbs phenomenon). For this reason, axial T2-weighted images serve as a useful tool for detecting cord abnormalities and confirming lesions suspected on sagittal T2-weighted images. Gradient-echo images are very susceptible to magnetic artifacts; this important characteristic makes them useful for detecting small areas of hemorrhage, such as with cervical spine trauma and vascular malformations. However, these images can also overestimate the degree of canal and foraminal stenosis secondary to artifact from the adjacent bone. Because of the rapidity with which gradient-echo images are acquired, studies can be obtained with higher resolution than that required for other pulse sequences and even as a 3D volume set, which allows for isotropic voxels and reformations in multiple planes. This volume set can then allow one to characterize the cervical foramina in the appropriate oblique plane. For evaluation of vascular structures in the neck, MR angiography can be obtained without contrast, using 2D or 3D time-of-flight or phase-contrast imaging. These sequences create contrast between flowing and stationary structures. Phase-contrast imaging may also provide flow-velocity information. As a result of the technique, time-of-flight imaging shows fat or subacute thrombus as bright signal and may be useful in detecting small, subtle thrombi. The 3D techniques require more time and are slightly less sensitive to slow flow states. Gadolinium-enhanced MR angiography may also be obtained and is extremely accurate. Although the cervical spine is injured in only 2% to 3% of blunt trauma accidents,1 the potential for instability and critical neurologic injury makes prompt identification and management of cervical spine injuries important. Patients with suspected cervical spine injury should be evaluated initially with conventional radiographs (AP, lateral, and open-mouth odontoid views). CT imaging offers greater osseous detail than does conventional radiography and may reveal fractures or details that are not detected with radiography. CT is especially helpful in assessing fractures of the occipital condyles and cervicothoracic junction, where osseous overlap on conventional radiographs makes fracture detection difficult. MRI provides soft-tissue visualization superior to that of conventional radiography or CT and is useful for the assessment of spinal cord injury, ligamentous injury, degree of spinal stenosis, and additional fracture evaluation. Occult fractures not visible on conventional radiographs or CT images may be detected by the presence of vertebral body edema on MR images. Although MRI is extremely sensitive in identifying cervical spine fractures, their characteristics and the exact appearance of the osseous components can be challenging; CT may be a better choice for assessing such details. In addition, MRI is useful for the evaluation of obtunded patients or those with cervical spine injury, neurologic deficits, or an unreliable physical examination.2–7 MRI is indicated specifically when neurologic deficit, vascular injury, or soft-tissue injury is suspected in the setting of trauma. It is also useful in assessing posttraumatic sequelae.8 Imaging spinal gunshot injuries is controversial. Theoretically, a ferrous gunshot fragment may become mobile, but most bullets are nonferrous, and therefore such patients can usually be imaged without consequences. Unfortunately, the exact composition of a gunshot fragment is seldom known, and therefore MRI remains controversial and dependent on the clinical need.9,10 It should be noted that there are obstacles to obtaining MRI studies in the trauma setting, especially with regard to cervical spine trauma, because patients may have clinically significant neurologic deficits. These obstacles include the following: • Lack of availability of MRI capabilities on an urgent basis • MR-incompatibility of some ventilators, traction devices, and other equipment • Lack of clinical access to patients during the imaging study MRI protocols vary by institution, but commonly used sequences in trauma evaluation include the following11: • Sagittal T1-weighted images to assess the alignment of the cervical spine, vertebral body integrity, fractures, and spinal cord caliber • Sagittal T2-weighted images to assess for the presence of cord edema, compression, and spondylotic changes • Sagittal STIR images to assess for the presence of para-spinal ligamentous injury and bone marrow edema • Axial T1-weighted and T2-weighted images to assess for the presence of posterior element fractures, to evaluate for spinal stenosis, to better define disc pathology, and to confirm the precise location of abnormalities detected on sagittal images • Sagittal T2-weighted gradient-echo images (in some institutions) to assess for the presence of acute spinal cord hemorrhage and disc herniation (high signal in the disc even with severe osseous degeneration, which enables the distinction between bone fragments and a disc herniation)
Specialized Pulse Sequences and Protocols
Traumatic Conditions
Anatomy | Evaluation |
Spinal column/vertebral bodies | Alignment Vertebral body fracture Posterior element fracture Edema Degenerative change |
Ligaments | Anterior longitudinal ligament Posterior longitudinal ligament Interspinous and supraspinous ligaments Ligamentum flavum Evaluation for edema/rupture |
Spinal cord | Edema Hemorrhage Compression Syrinx |
Epidural space | Hematoma Disc herniation Osseous fragment |
Vascular | Vertebral artery |
Source: Takhtani D, Melhelm ER. MR imaging in cervical spine trauma. Magn Reson Imaging Clin N Am 2001;8:615–634. Modified with permission.
Regardless of the specific institutional MRI protocol, a systematic approach (see Chapter 3) for the evaluation of cervical spine MRI should be used to avoid missing pathologic conditions (see Table 10.1 for important cervical spine structures to evaluate). In addition, it is essential that the interpretation of the MRI findings be performed in conjunction with that of the other available imaging modalities, including conventional radiographs (with flexion and extension views if clinically indicated) and CT (see Chapter 17).
Classification of Cervical Spine Trauma
Cervical spine injuries can be classified based on the mechanism of injury. Although six categories have been described (vertical compression, compressive flexion, distractive flexion, lateral flexion, compressive extension, and distractive extension12) (Fig. 10.1), the classification scheme is simplified here into three broad categories:
• Hyperflexion
• Hyperextension
• Axial loading
In many instances, the mechanism of injury can be difficult to determine from an analysis of the clinical situation (in the absence of imaging findings), and therefore clinicians may choose to broadly classify cervical spine injuries as follows:
• Secondary to blunt trauma
• Secondary to penetrating trauma
Fig. 10.1 An artist’s representation of the Allen-Ferguson mechanistic classification system for subaxial cervical spine fractures. (From Chapman JR, Anderson PA. Cervical spine trauma. In: Frymoyer J, Ducker TB, Hadler NM et al, eds. The Adult Spine: Principles and Practice. 2nd ed. Philadelphia: 1997:1245–1295. Reprinted with permission.)
• Occipitocervical junction
• Suboccipital cervical spine (C1-C2)
• Subaxial cervical spine (C3-C7)
More recently, the subaxial cervical spine injury classification system has been described as an approach that recognizes the importance of fracture morphology, neurologic injury, and integrity of the discoligamentous complex.13 A systematic evaluation of these three components can be used to guide the treatment of patients with cervical spine fractures.
Hyperflexion Injuries
Flexion-compression injuries range from the minor anterior compression of the anterosuperior end plate (Fig. 10.2) to a severe teardrop or quadrangular fracture. These injuries are associated with retrolisthesis, kyphosis, and circumferential soft-tissue disruption. The radiographic evaluation of flexion-compression injuries includes inspection for the following:
• Anterior and middle column compromise
• Vertebral body-height loss
• Translation
• Angulation
• Posterior element competence
Although conventional radiographs and CT scans can evaluate fracture pattern, alignment, angulation, and translation, MRI provides additional diagnostic value and can assist with the determination of treatment options for such patients because it facilitates the assessment of spinal cord compression and posterior element compromise.
Flexion-distraction forces can lead to facet subluxations, dislocations, or fracture-dislocations. These injuries represent a spectrum of osteoligamentous pathology, ranging from the purely ligamentous dislocation to fracture of the facet and lateral mass. MRI helps assess the compromise of posterior musculature, interspinous ligaments, ligamentum flavum, and facet capsules that is often seen with flex ion-distraction injuries.14 The role of MRI in the treatment algorithm of patients who present with bilateral cervical facet dislocations (Fig. 10.3) without neurologic compromise is the subject of substantial debate in the literature and among spine surgeons.14–16 The treatment options include MRI before attempting closed reduction or surgical intervention; closed reduction with traction while monitoring the patient’s neurologic examination; and surgical intervention via anterior, posterior, or combined approaches.14–16 One of the purposes of obtaining an MRI study before the reduction of bilateral facet dislocations is to rule out the possibility of an extruded disc fragment that may displace into the spinal canal during a closed reduction (Fig. 10.4).
Fig. 10.2 C7 vertebral compression fracture. Sagittal T2-weighted (A) and T1-weighted (B) images showing the fracture (arrow on each) with minimal loss of height.
Fig. 10.3 Artist’s sketches illustrating the pathology in bilateral facet dislocation. (A) A lateral view of osseous structures shows that the facets are perched and that additional translation will lead to complete dislocation. (B) A lateral view before reduction shows approximately 50% translation of the superior vertebral body relative to the inferior one and displacement of the intervertebral disc. (C) A lateral view after reduction shows that the intervertebral disc has displaced into the spinal canal and compressed the spinal cord during the reduction maneuver.
Most flexion injuries are well visualized on MRI, and MRI is particularly effective for the assessment of the following11:
• Alignment
• Fractures
• Ligamentous injury
• Cord abnormalities
• Acute disc herniations
• The cause of anterior subluxation, either chronic degenerative changes or hyperflexion sprain
Facet joint injuries may be seen on parasagittal or axial images, which show increased signal on T2-weighted images secondary to edema from facet capsule tears.11,17–19 Injury to posterior ligaments may be seen as areas of hyperintensity on T2-weighted images, especially fat-suppressed T2-weighted or STIR images (Fig. 10.5).
Hyperextension Injuries
Cervical spine extension injury results in the posterior translation or rotation of a vertebral body in the sagittal plane.6,11,20 Hyperextension injuries often are produced by rear-impact motor-vehicle collisions or direct facial trauma. In cervical spine hyperextension injuries, potential findings include the following6,11,17,19,20:
Fig. 10.4 Bilateral cervical facet dislocation. (A) A sagittal T2-weighted image showing anterior translation of C7 over T1 with an associated disc extrusion (arrow) and cord compression. Parasagittal T2-weighted (B) and gradient-echo (C) images showing the inferior articular process of C7 (arrow on each) displaced anterior to the superior articular process of T1 (arrowhead on each) .
Fig. 10.5 A sagittal STIR image shows edema in the supraspinous ligament region (arrowhead) and interspinous region at C6-C7 and C7-T1, with a small, focal region of increased T2-weighted signal in the ligamentum flavum at the C7-T1 level (arrow) compatible with a partial tear.
Fig. 10.6 A sagittal STIR image showing an intervertebral disc rupture at C4-C5 (arrow) in a patient who sustained a hyperextension injury to the cervical spine. Note the associated prevertebral hematoma and the severe multilevel degenerative stenosis with associated cord signal change.
• Tear(s) of the anterior longitudinal ligament
• Avulsion of the intervertebral disc from an adjacent vertebral body
• Horizontal intervertebral disc rupture (Fig. 10.6)
More severe and potentially unstable hyperextension injuries may be associated with the following6:
• Prevertebral hematoma
• Widening of the disc space
• Posterior ligament complex edema
• Herniated disc
Elderly patients with spondylosis and kyphosis of the cervical spine may suffer spinal cord injury without fracture or ligamentous injury because of posterior infolding of the ligamentum flavum upon a spinal canal already narrowed by posterior vertebral osteophytes.6
Whiplash injuries often have no associated osseous injury on standard radiographs or CT images, and flexion-extension radiographs may be nondiagnostic because of poor excursion secondary to pain. However, MRI is of limited value for the assessment of whiplash; several studies have failed to show positive MRI findings in the absence of neurologic symptoms.18,21 In contrast, patients with a fused cervical spine secondary to ankylosing spondylitis or diffuse idiopathic skeletal hyperostosis may benefit from an MRI examination to assess for acute fracture, instability, or neurologic compromise. In such patients, the fused cervical spine acts like a long-bone fracture, and even minimally displaced fractures may be unstable (Fig. 10.7).22
Finally, MRI can assess intervertebral disc injury and subtle fractures caused by any of the above-mentioned mechanisms.11,17–19,23 Intervertebral disc injury may range from tear (s) of the outer annulus fibrosis (seen as increased T2-weighted signal in the outer annular fibers) to frank intervertebral disc herniation. The identification of an annular tear on MRI does not indicate acute traumatic injury and can be seen in asymptomatic individuals.24,25 Intervertebral disc separation from the adjacent vertebral body may be seen as a horizontal hyperintense T2-weighted signal.11,17,19 Subtle fractures, such as vertebral end-plate fractures, may be best visualized with MRI because it can detect osseous edema and hemorrhage not seen on conventional radiographs or CT images.11,17,19
Fig. 10.7 Ankylosing spondylitis. (A) A T2-weighted image shows multilevel ankylosis of the cervical spine and ossification of the posterior longitudinal ligament posterior to the body of C2 but no specific evidence of fracture. (Conventional radiographs and CT images also showed no evidence of fracture.) (B) A sagittal STIR image shows a nondisplaced “fracture” or injury through the anterior column at C6 (arrow) and posterior column injury; both injuries manifested as regions of increased signal intensity with the use of this fluid-sensitive pulse sequence.
Axial Load Injuries
Axial load injuries are caused by the axial transmission of force through the skull, through the occipital condyles, and into the spine. This force transmission can cause a Jefferson burst fracture or burst fractures of the subaxial cervical spine. MRI is useful for the assessment of C1 compression fractures and associated pathologies such as lateral mass displacement on coronal images, atlantodental interval increase on sagittal images, and transverse ligament disruption on axial images.11 For burst fractures, MRI is useful for diagnosing associated spinal cord injury caused by an acute herniated disc or retropulsion of osseous fragments (Fig. 10.8). Because a purely axial force subjects the posterior capsuloligamentous structures to compression only, these posterior structures should remain intact.11,20 However, there often is some degree of spine flexion during the traumatic event that may cause injury to the posterior spinal elements, which can be detected by MRI.20 It is important to carefully scrutinize the fat-suppressed T2-weighted and other images for evidence of injury to the posterior ligamentous and osseous structures because such injury will lead to consideration of posterior fusion in addition to the anterior decompression and fusion that is often performed for patients with cervical burst fractures.
Occipitocervical Junction Injuries
Although injury to the occipitocervical junction occurs in a small percentage of blunt trauma victims (0.8% in one study26), recognition of such injuries is crucial because of their devastating effects.27–30 A detailed discussion of occipitocervical craniotomy and the various measurement techniques for evaluation of occipitocervical pathology is beyond the scope of this chapter, but presented here is an overview of the major types of occipitocervical traumatic findings as seen on MRI. It is important to keep in mind that MRI studies of the occipitocervical junction should be reviewed in conjunction with conventional radiographic and CT imaging.
Atlantooccipital Dissociation
Atlantooccipital dissociation is any separation of the atlantooccipital articulation. The skull may displace anteriorly, posteriorly, or superiorly, and may be complete (dislocation) or partial (subluxation). Atlantooccipital dissociation can be a devastating injury.27–30 The primary injury is to the ligaments that provide structural support to the cervicocranial junction. In addition, even without frank dislocation, the occiput–C1 junction may be injured, as indicated by postmortem studies.27,28 Although this injury may be fatal, improvement in resuscitative and medical treatment has increased survival rates. CT imaging may be used to assess associated fractures or relationships among the basion, dens, occipital condyles, and atlas in conjunction with atlanto-occipital dissociation, whereas MRI is better at detecting injury to the cervicocranial ligaments (e.g., transverse, apical, cruciate, atlantooccipital membrane and capsular ligaments, tectorial membrane), brainstem, or spinal cord.11,19,31
Fig. 10.8 Cervical burst fracture. Sagittal fat suppressed T2-weighted (A) and T1-weighted (B) images showing a C5 burst fracture (arrow on each) with moderate loss of height, retropulsion, and spinal cord contusion (arrowhead on A). (C) Axial T2-weighted gradient-echo image shows the sagittal component of the fracture (arrow) .
Trauma to the Atlas
Axial load to the occipitocervical junction at the atlas may result in a burst fracture of the atlas. The injury is visualized on open-mouth odontoid radiographs or coronal CT images.32,33 As indicated by the cadaver study of Spence et al.,34 combined overhang of the lateral masses of C1 over C2 of ≥6.9 mm is associated with transverse ligament rupture and indicates a relatively unstable Jefferson burst fracture. The axial T2-weighted images can be critically evaluated to rule in or rule out injury to the transverse ligament. These images should be carefully scrutinized for increased T2-weighted signal in or along the course of the transverse ligament. The ligament should also be evaluated for regions of discontinuity.
Trauma to the Axis
C2 is the most commonly fractured cervical spine level.26 In the elderly, odontoid fractures tend to be posteriorly displaced. Fracture location, displacement, and angulation are important factors that assist with clinical decision-making. Lateral cervical radiographs and CT scans can be used to characterize such fractures and better evaluate the osseous detail. MRI can assist in the evaluation of these fractures by providing assessment of the degree of edema at the fracture site (Fig. 10.9). This information provides insight into the age of the fracture (acute versus subacute versus chronic), which can be used to guide treatment. The sagittal and axial T2-weighted images should also be carefully evaluated to determine the degree of neural compression, from either a displaced fracture or underlying degenerative changes.
Fig. 10.9 A sagittal T2-weighted image of a type II odontoid fracture showing edema at the fracture site (arrow), indicating an acute or subacute fracture. Note the prevertebral edema or hematoma (arrowhead) .
Atlantoaxial Dissociation
Atlantoaxial dissociation may be caused by distraction with superior migration of the atlas away from the axis or by odontoid fractures with anterior or posterior displacement of C1 relative to C2. MRI clearly depicts the associated pathology seen with atlantoaxial dissociation.11 As noted above, MRI is very useful for detecting ligamentous and spinal cord injury. The relationship of the atlas to axis is clearly visible with MRI. Specifically, the integrity of the transverse ligament should be evaluated along with the approximate size of the anterior atlantodens interval. This measurement is more frequently evaluated on flexion and extension lateral cervical spine radiographs (Fig. 10.10).
Vertebral Artery Injury
Vertebral artery injury is associated with blunt cervical trauma, with an incidence as high as 11%.35 This potentially devastating injury may occur with cervical spine fractures extending into the transverse foramen, but it is associated most often with unilateral or bilateral facet dislocations.19,36 MR angiography may be used to assess vertebral artery patency, especially with such fractures.19 MR angiography may show areas of vascular stenosis or occlusion. Flowing blood creates a signal void on axial SE images and is seen as a bright signal on gradient-echo images; merging the data from these two modalities can help determine the status of blood flow in the vertebral arteries19,37 (Fig. 10.11).
The types of vertebral artery injuries are thrombosis, dissection, and transection (rare). MR angiography shows vertebral artery thrombosis as the absence of flow-related enhancement on images in the expected course of the vertebral artery and as an acute thrombus in the foramen transversarium, dissections as a tapering of the vessel, and transections as a focal discontinuity of the vessel. Major clues to vascular injury include changes in vessel caliber, loss of the normal rounded shape, increase in caliber from proximal to distal (except at the carotid bulb), or the presence of an extraluminal thrombus or a slit or dark band through the lumen.
Penetrating Trauma
Penetrating injury to the cervical spine can be caused by projectiles (e.g., bullets) or puncture mechanisms. Missiles can cause spinal cord injury by direct penetration, displacement of bone fragments into the spinal canal compressing the spinal cord, or a blast effect. The osseous architecture of the spine often protects the spinal cord from direct injury from a stabbing mechanism because the lamina and spinous processes can deflect the penetrating object into the para-spinal soft tissues. MRI is useful for assessing the specific location, extent, and type of cord injury from penetrating trauma.
Fig. 10.10 Atlantoaxial instability. Lateral extension (A) and flexion (B) radiographs showing instability at the C1-C2 level with widening of the anterior atlantodens interval on the flexion radio-graph (arrow onB) relative to the extension radiograph. (C) A sagittal T2-weighted image showing prominence of the transverse ligament (arrow) .(D) An axial T2-weighted image also shows that prominence (arrowhead) and edema at the insertion site of the transverse ligament on the lateral masses of C1 (arrows) .
Fig. 10.11 Vertebral artery injury after unilateral facet dislocation at C5-C6 without spinal cord injury. (A) A sagittal T2-weighted image shows an injured disc at C5-C6 with increased signal intensity in the disc and probable avulsion of the anterior longitudinal ligament (arrow) . Prevertebral edema (small arrowheads) and edema in the posterior paraspinal musculature (large arrowhead) are present. (B) An MR angiogram (anterior view) from a 2D time-of-flight acquisition shows absence of signal intensity in the expected course of the right vertebral artery (arrowheads) . Note the normal course of the left vertebral artery (arrows) .(C) An axial image from a 3D gradient-echo acquisition shows an oval area of low signal intensity in the right foramen transversarium (arrow) corresponding to a thrombus in the right vertebral artery. Note the normal flow-related enhancement in the left foramen transversarium (arrowhead) . (D) An axial FSE image obtained at a similar level to that in C shows a high-signal-intensity thrombus (arrow) in the right foramen transversarium, indicative of a thrombosed vertebral artery. Note the normal flow void of the left vertebral artery in the left foramen transversarium (arrowhead) . (From Torina PJ, Flanders AE, Carrino JA, et al. Incidence of vertebral artery thrombosis in cervical spine trauma: correlation with severity of spinal cord injury. AJNR Am J Neuroradiol 2005;26:2645–2651. Reprinted with permission.)
Characterization of Spinal Cord Injury
The severity of spinal cord injury depends on the characteristics of the traumatic event (including the amount, duration, and location of the applied force) and the underlying health of the spinal cord. Spinal cord insult may range from a concussive injury (purely functional and reversible) to complete transection (irreversible). Spinal cord concussive injury often has no MRI evidence of edema (increased T2-weighted signal), or the edema is transient and resolves with time.11 Spinal cord contusion is a more severe injury and may be caused by transient compression or stretching of the spinal cord. Spinal cord compression may show injury characteristics similar to those of spinal contusion, but it can be associated with a specific compressive lesion such as disc herniation (Fig. 10.12) or osseous fragment.
An injured spinal cord segment may have an increase in cord diameter because of swelling, edema, or hemorrhage. MRI characteristics of an injured spinal cord segment are based on the degree of swelling, edema, or hemorrhage, each of which may have a different pattern of signal changes on various pulse sequences.7 On T2-weighted images, edema and acute hemorrhage are seen as bright signal, whereas chronic hemorrhage is seen as darker signal. Gradient-echo images show dark areas that are larger than the abnormality on the T2-weighted images. This enlargement, or “blooming,” is the result of the magnetic susceptibility artifact from met-hemoglobin.7 The anatomic location, morphology, and length of the spinal cord lesion are important factors in determining the degree of neurologic loss. Initial neurologic deficit and potential for recovery are related directly to the extent of spinal cord damage by hemorrhage or edema.7 Evidence of parenchymal hemorrhage on MRI may predict worse functional outcomes or neurologic recovery than is associated with a spinal cord injury with predominantly edematous changes.7
Highly T2-weighted images offer a myelographic effect for the assessment of spinal cord compression. These images should be carefully evaluated in the sagittal and axial planes for regions of effacement of the ventral or dorsal CSF spaces, which indicate spinal stenosis and cord compression. Trauma patients who have underlying degenerative or congenital stenosis are at increased risk for spinal cord injury because of the decrease in the cross-sectional area available for the spinal cord.
Clinical and experimental evidence has shown that surgical decompression of stenotic areas has a beneficial effect on neurologic recovery, which makes prompt identification of stenotic areas and distinguishing these areas from simple contusions important.11 In addition, MRI assessment of cervical spine fractures in obtunded or uncooperative patients may identify disc herniations that may cause spinal cord compression and iatrogenic or progressive neurologic injury during fracture reduction.20,38,39
Fig. 10.12 A sagittal T2-weighted image showing a large central disc extrusion at the C5-C6 level with associated increased cord signal intensity (arrow) compatible with myelomalacia. Note the elevation of the posterior longitudinal ligament (arrowhead) .
Characterization of Cervical Spine Instability
White et al.40 defined cervical spine instability as the inability to maintain a normal association between vertebral segments while under a physiologic load. Cervical spine instability may be caused by damage to the osseous and/or ligamentous structures. Conventional radiographs and CT scans often provide the best assessment of osseous injuries. Ligamentous injury contributing to cervical spine instability may be assessed with flexion and extension lateral cervical spine radiographs and by physical examination. MRI can also be used to evaluate for ligamentous injury; the sensitivity for detection of such injuries is greatest within 24 to 72 hours postinjury.5,17,19,41 Important ligaments to assess include the following20:
• Anterior longitudinal ligament
• Posterior longitudinal ligament
• Posterior column ligament complex (supraspinous ligament, interspinous ligament, and ligamentum flavum), which has been recognized as an important restraint to spinal instability (especially kyphosis)
• Transverse ligament
MRI characteristics of ligamentous injury include increased T2-weighted signal (from edema) within the ligamentous and other posterior structures (Fig. 10.13) or loss of ligament continuity (normally a low intensity continuous signal). Ligamentous injury is best assessed on STIR or fat-suppressed T2-weighted images.20 A ligament strain, without complete disruption, may be seen as an elongated or redundant ligament on sagittal MR images. Despite the capability of MRI to detect ligamentous injury, not all MRI-detected ligamentous injuries result in spinal instability or warrant treatment.20,21 For example, minor motor-vehicle accidents that result in acute whiplash injury of the cervical spine without fracture do not need emergent MRI evaluation for ligamentous injury and may be treated symptomatically only.21
Fig. 10.13 A sagittal STIR image of a patient who sustained a hyperflexion injury to the cervical spine shows increased signal intensity within the region of the supraspinous and interspinous ligaments between C3 and C6 (arrow) . These findings are compatible with injury to the posterior ligamentous structures.
With the increasing availability of flexion–extension (kinematic) cervical spine MRI, a dynamic assessment of cervical spinal instability and associated stenosis can be obtained.42 Although such information provides insight into the degree of spinal instability, it tends to be most useful for the evaluation of patients with degenerative disorders of the cervical and lumbar spine (Fig. 10.14).43 Patients who have sustained severe trauma to the cervical spine are likely to be immobilized. After a period of immobilization and after frank instability of the cervical spine has been ruled out with patient-controlled flexion–extension cervical spine radio-graphs, a kinematic cervical spine MRI study can be considered. The information obtained from such kinematic studies can be used to guide surgical treatment and may allow the surgeon to decide among anterior, posterior, or combined surgical approaches.
Degenerative Conditions
Degenerative changes of the cervical spine are common after the fourth decade of life.44 Cervical spine degeneration may be asymptomatic or have acute or insidious onset of symptoms; it may result in pain, stiffness, radiculopathy, myelopathy, and even permanent disability. Degenerative pathology may affect multiple areas in the cervical spine, including the following:
• Intervertebral discs
• Facet joints
• Uncovertebral joints of Luschka
• Ligaments
• Paravertebral musculature
Because these elements are biomechanically linked, a single cervical spine level may have multiple degenerative pathologies and cause adjacent-level degenerative changes. Conventional radiographs often are the initial screening studies for evaluating cervical spine degeneration and may guide the selection of more advanced imaging techniques.45
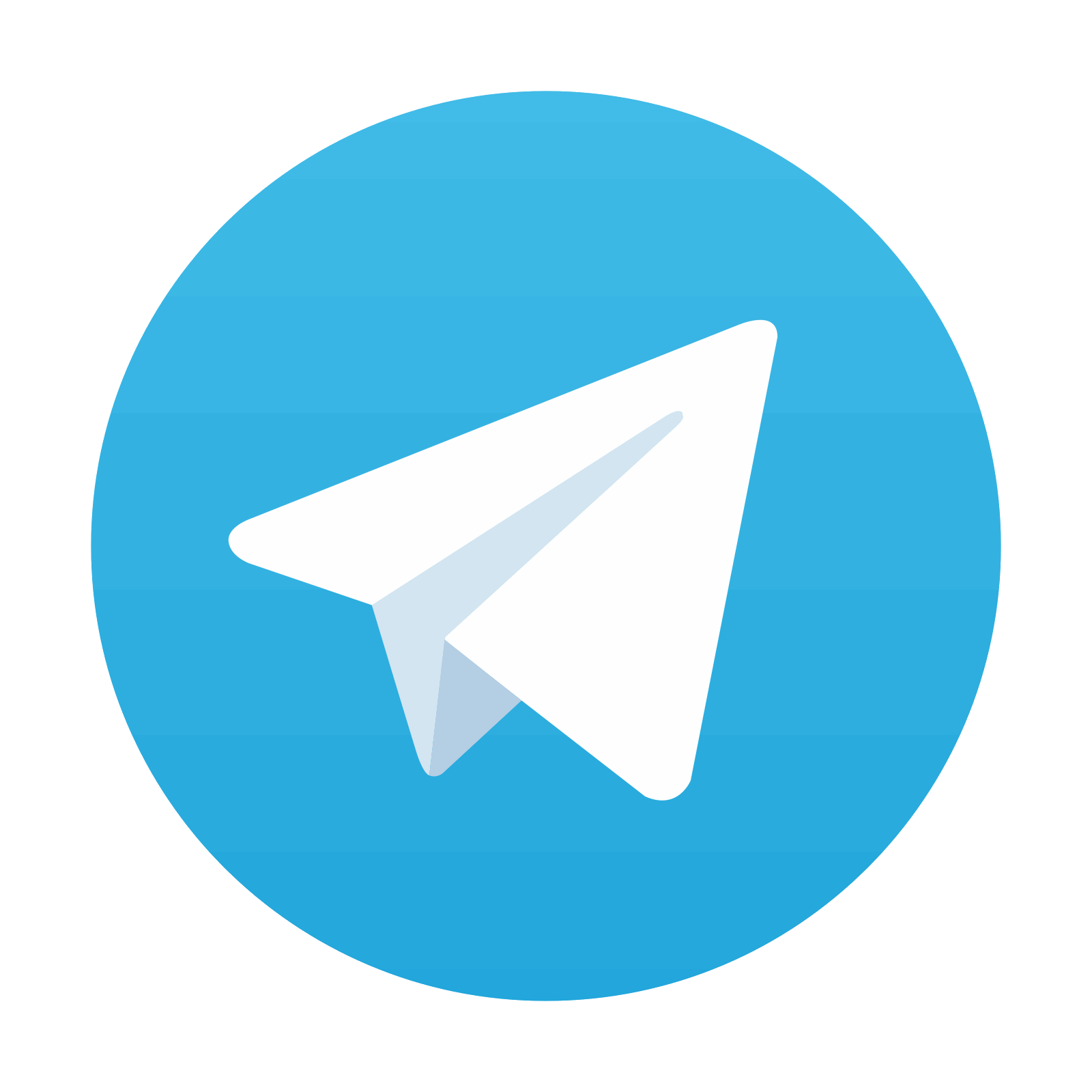
Stay updated, free articles. Join our Telegram channel

Full access? Get Clinical Tree
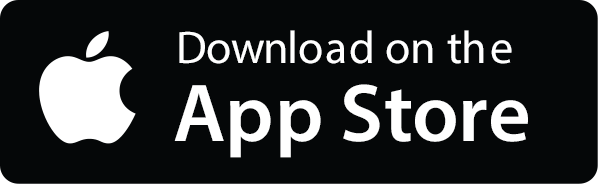
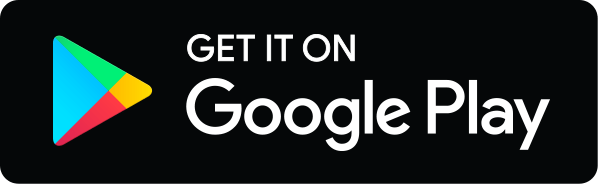