Doppler sonography classification
Types/examples
Intraluminal structural venous abnormality: This is an echogenic structure extending from the endothelial lining of the vein wall with/without the presence of functional abnormality. These abnormalities include web, flap, septum, membrane, and malformed valve
Web: multiple septae and/or flaps located in a cluster
Flap: thin linear echogenic structure extending from endothelial lining of vein wall
Septum: thin linear echogenic structure extending from endothelial lining of vein wall and attached to it at both ends. Septum may extend across a vein to attach on opposing sides or attach on same side
Membrane: membranous structure almost occluding the entire diameter of the vein
Malformed valve: dysdynamic or fibrous valve
Extraluminal structural venous abnormality: This is a restriction of the venous wall or stenosis. These abnormalities include stenosis and annulus
Stenosis: cross-sectional area (CSA) measurement of ≤3 mm2
Annulus: circumferential thickened vein wall that is restricting the vein from fully expanding with respiratory or positional changes
Functional venous abnormality: This is an abnormal cerebral venous outflow in the presence of a structural venous anomaly. These abnormalities include reflux/bidirectional flow, paradox, and no flow
Reflux/bidirectional flow: present in the IJV for more than 0.88 s with the head at 90° and 0°
Paradox: vein wall not reacting to respiratory phase; noncompliant
No flow: no color flow noted in vein, despite deep breaths
Recently several CNS disorders such as transient global amnesia, transient monocular blindness, leukoaraiosis, dementia, normal-pressure hydrocephalus, cough headache, and primary exertional headache have been linked to the presence and severity of uni- or bilateral jugular venous reflux [2, 8–12]. Further, more recently the introduction of a composite criteria-based vascular condition named chronic cerebrospinal venous insufficiency (CCSVI) generated an intense interest in better understanding the role of the extracranial venous system in the pathophysiology of CNS disorders, especially in multiple sclerosis (MS) [13, 14]. This returned possible vascular etiology of MS lesions to the forefront of MS research.
CCSVI is characterized by impaired brain and spinal cord venous drainage due to outflow obstruction in the extracranial venous system, mostly related to anomalies in the internal jugular (IJV) and azygos veins [14–16]. It was hypothesized that these venous anomalies may cause alterations to blood flow that eventually result in iron deposition, degeneration of neurons, and characteristic brain injury patterns. The CCSVI diagnosis is based mainly on color Doppler sonography (DS) findings in the extracranial (neck) and intracranial veins by assessing five venous hemodynamic (VH) criteria (with cutoff of ≥2 positive criteria used for diagnosis of CCSVI) [17, 18]. From the time it was first mentioned, the CCSVI theory has not ceased to provoke controversy and attention in the scientific community and the media, mostly because the sensitivity and specificity results originally reported [14, 17] were not reproduced by other groups [19–21]. Also, the reliability of using DS in the diagnosis of CCSVI is questionable without proper training and has been the focal point of recent statements from various societies [19, 22, 23]. Further, the CCSVI concept did not take into account the physiological variations of the IJV in diameter [24].
One of the main criticisms of the CCSVI concept arose from the use of endovascular procedures to unblock potentially stenotic IJV and azygos veins in an open-label fashion without previously establishing: (a) diagnostic imaging modalities and protocols that will serve as a “gold standard” for the detection of extracranial venous anomalies and (b) safety as well as efficacy of the endovascular procedures in properly designed randomized, double-blinded, sham-controlled studies, as well as the potential usefulness of endovascular treatment of vein pathology related to CCSVI [5, 25–27]. So far there have been several case reports concerning patients who had serious side effects after angioplasty for CCSVI like IJV stent thrombosis requiring open thrombectomy, stent migration, aneurismal vein dilatation, cranial nerves neuropathy, as well as some reports of lethal cases [2, 28, 29].
Furthermore, classification, existence, and interpretation of venous abnormalities are questionable, given the fact that the same can be found among healthy populations and patients with other CNS disorders [11, 12, 30, 31, 32, 33]. At this time, it remains unclear whether extracranial venous abnormalities represent an acquired pathology or developmental variants.
It is pretty clear that there is an urgent need to define and validate the spectrum of extracranial venous abnormalities and their relationship to neurodegenerative diseases. Since there is still no established noninvasive or invasive diagnostic imaging modality that can serve as a “gold standard/benchmark” for the detection of extracranial venous anomalies, it is most likely that only a multimodal imaging approach will represent the most comprehensive means for the screening, diagnosis, as well as monitoring for these venous abnormalities ([2, 9, 23, 34]).
Anatomy and Function of the Cerebral Venous System
It is important to first appreciate the anatomy and function of the cerebral venous drainage system in order to understand the potential role of the extracranial venous system in diseases of the CNS and aging. The venous system contains approximately 70 % of the circulating blood volume, with approximately three-quarters of it within small veins and venules [35, 36]. Cerebral venous system drainage is composed of two systems: the superficial and the deep venous system (Fig. 1). The superficial system drains blood from the cortex and superficial white matter (WM) by cortical veins, collected by dural sinuses. There are two important dural sinuses: the superior sagittal sinus (SSS) draining dorsolaterally and the cavernous sinus draining antero-ventrally. The transverse sinus then drains the SSS equally on both sides in only 20 % of cases and asymmetrically in more than 50 % of cases, depending on the configuration of torcular Herophili. In 20 % of cases, one transverse sinus drains the SSS in total (most often on the right side), and the other one drains the straight sinus, which collects blood from the deep venous system [5, 37–39].
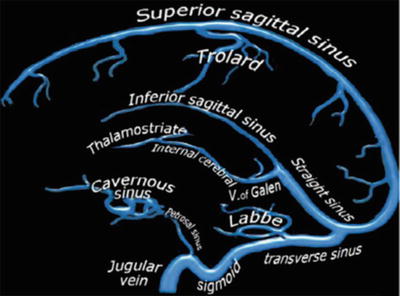
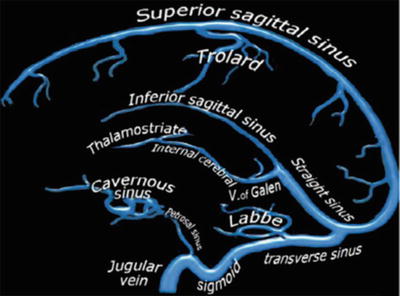
Fig. 1
Intracranial venous system anatomy of dural sinuses, cortical veins, deep intracerebral veins, and cavernous sinus (The figure was reproduced with permission from the Radiology Assistant website: (http://www.radiologyassistant.nl/en/p4befacb3e4691/cerebral-venousthrombosis.html))
The cavernous sinus extends from the superior orbital fissure to the petrous apex, which receives orbital venous and middle cranial fossa drainage. From the cavernous sinus, blood drains posterolaterally along the superior petrosal sinus into the transverse sinus and inferior-laterally along the inferior petrosal sinus into the sigmoid sinus [5, 40]. The deep cerebral venous system drains the deep WM and the regions surrounding the lateral and third ventricles or the basal cistern. Three veins unite just behind the interventricular foramen of the Monro to form the internal cerebral vein (s). These include the choroid vein, septal vein, and thalamostriate vein. The vein of Galen is a short (1–2 cm long), thick vein that passes posterosuperiorly behind the splenium of corpus callosum in the quadrigeminal cistern. The vein of Galen receives the internal cerebral vein, the basal veins of Rosenthal, and the posterior fossa veins and then drains to the anterior end of the straight sinus where this unites with the inferior sagittal sinus. The main collecting vein for the deep venous system is the straight sinus, which receives the venous blood from the vein of Galen and flows into the transverse sinus (most often into the left side). The basal vein of Rosenthal is an important collateral pathway for the internal cerebral veins and the vein of Galen. Venous blood flow can bypass the straight sinus by connecting with the superficial Sylvian vein via the deep Sylvian vein [38, 39, 41]. Venous drainage of the posterior fossa mainly depends on the galenic system and the petrosal system and, to a lesser extent, the tentorial veins and the transverse sinuses.
Extracranial Cerebral Venous Drainage Pathway: Neck Veins
The cerebral venous drainage pathway continues via neck veins, mainly the IJV, vertebral venous system, and deep cervical veins (veins in cervical soft tissue) (Fig. 2) [5, 35, 38].
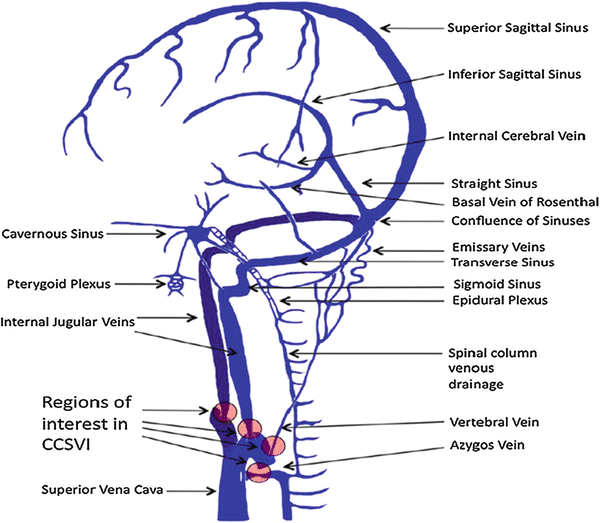
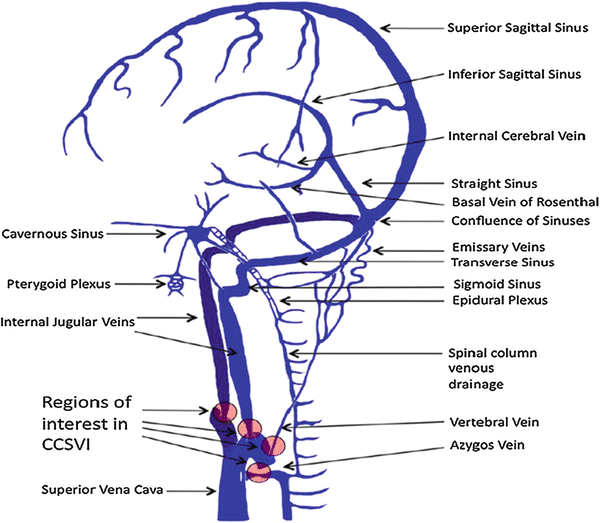
Fig. 2
Illustration depicting the predominant veins and sinuses involved in the craniocervical venous outflow. Venous narrowing is depicted at locations of interest in chronic cerebrospinal venous insufficiency. (The figure was reproduced with permission from Lazzaro MA, Zaidat OO, Mueller-Kronast N, Taqi MA, Woo D Endovascular therapy for chronic cerebrospinal venous insufficiency in multiple sclerosis. Front Neurol 2011, 2:44)
The IJVs are the largest veins in the neck and are generally considered to be the most important cerebral venous outflow pathways. Venous drainage of the superficial and deep cerebral venous system is via the transverse sinuses to the sigmoid sinuses, which then drain into the IJV [5, 32, 36]. The inferior petrosal sinus, a major drainage route collecting blood from the cavernous sinus, communicates with the basilar plexus, anterior and lateral condylar veins, anterior condylar confluence, and vertebral venous plexus before draining into the IJVs. The IJVs then join with the subclavian veins to form the brachiocephalic vein (BV). The confluence of the bilateral BV is the superior vena cava, which ultimately drains venous blood into the heart. Several tributaries in the neck also drain into the IJVs. These bilateral IJV branches will interconnect with each other at the midline to form anastomosing plexi that can serve as collateral channels to maintain adequate venous drainage when the principal pathways are obstructed [5].
The vertebral venous system consists of two components; one is the vertebral venous plexus and the other is the vertebral vein (VV). The vertebral venous plexus can be subdivided as internal (posterior and anterior internal vertebral plexus) and external (posterior and anterior external vertebral plexus). The IJVs can also exhibit anastomosis with the other extracranial venous drainage system within the craniocervical junction region, which includes the anterior condylar confluent (ACC) and its tributes. Numerous anastomoses of the ACC make it a crossroad between the cavernous sinus, dural sinuses of the posterior fossa, IJVs, and posterior cervical outflow tract (vertebral venous system and deep cervical veins) [5, 36, 42, 43].
IJV Valves
Although there are anatomical variations, the valves are generally located about 0.5 cm above the union of the subclavian vein and IJVs at the lower limit of the jugular bulb, which are shown in 96.8 % of the general population. They make IJV a buffer zone between large central veins and the cerebral venous system. The IJV valves are generally thought to prevent the backflow of venous blood and backward venous pressure into the cerebral venous system during conditions where the central venous pressure or intrathoracic pressure is increased, such as chest compression during external cardiopulmonary resuscitation and severe or repetitive cough and straining [5, 44–46]. The pressure gradient across competent IJV valves can be as high as 100 mmHg. Without competent IJV valves, a sustained or prolonged retrograde-transmitted venous pressure via IJVs might impair cerebral venous drainage and lead to neurological deficits. For example, IJV valve incompetence has been associated with encephalopathy after cardiopulmonary resuscitation [5, 47].
Other Neck Veins Serving as Collaterals for Cerebral Venous Drainage
When there is narrowing of the principal pathways of the extracranial venous system, collateral veins probably represent physiological variations of the venous system that may play a compensatory role [2, 13]. The extra-jugular venous drainage system for cerebral venous drainage mainly consists of the vertebral venous system and deep cervical veins [5, 36, 40, 48, 49]. The external jugular vein (EJV) and anterior jugular vein (AJV), compared with the IJV, are located superficially in the neck. They serve as collaterals and become prominent (enlarged lumen) when the main cerebral venous drainage pathways (IJV and VV) are compromised. EJV is formed by the confluence of the posterior branch of the posterior facial vein and the posterior auricular vein [5, 50]. It usually terminates into the confluence of the subclavian vein and IJV. The AJV receives blood from superficial veins, such as EJVs, facial veins, or IJVs. They usually end in the subclavian vein or EJV. Bilateral AJVs may communicate via the jugular venous arch (JVA), which is located just above the sternum. The JVA receives tributaries from the thyroid gland via inferior thyroid veins. In summary, venous collaterals in the neck include the anterior (jugular venous system) and the posterior (vertebral and other deep neck venous system), and different patterns of collateral establishment may reflect the location and severity of venous outflow obstruction [5, 51, 52].
Extracranial Cerebral Venous Drainage Pathway: Abdominal and Thoracic Veins
There is communication of vertebral venous plexus system with the deep thoracic and lumbar veins, intercostal veins, as well as the hemiazygos and azygos veins. Abnormalities in these abdominal and thoracic veins may impair venous drainage from the vertebral venous system, which serves as an important collateral for cerebral venous drainage. The hemiazygos arch is connected with the left renal vein that represents a major outflow route for shunting blood into the inferior vena cava. Ultimately, the azygos vein serves as the final venous blood collector and drains into the superior vena cava. The anatomy and developmental stages of the abdominal/thoracic blood vessels can be quite variable (Fig. 3). For example, in some rare variations, the azygos vein also drains thoracic veins, bronchial veins, and even gonadal veins. The vein is so named because it has no symmetrically equivalent vein on the left side of the body [5, 6].
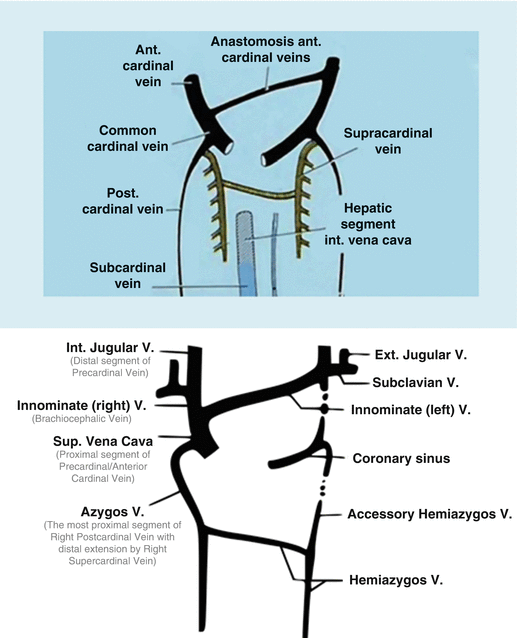
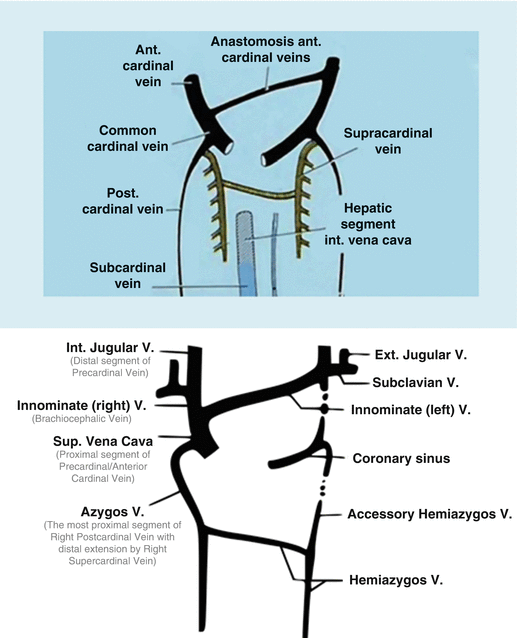
Fig. 3
Paired anterior cardinal veins form common cardinal veins with paired posterior cardinal veins, draining centrally into the sinus venosus (sinus horns) as depicted (top). Paired anterior cardinals soon form an anastomosis between them; the connection grows from the left to the right anterior cardinal vein to form the left brachiocephalic (innominate) vein (bottom). The left anterior cardinal vein distal (cranial) to the anastomosis becomes the “left internal jugular vein,” while the left anterior cardinal vein proximal to the brachiocephalic anastomosis regresses/atrophies to become the base of the “coronary sinus” of the heart as displayed. The right anterior cardinal (precardinal) vein proximal to the right brachiocephalic vein forms the superior vena cava (SVC) with the common cardinal and terminal/proximal segment of the posterior cardinal (postcardinal) vein (The figure was reproduced with permission from Lee BB: Venous embryology: the key to understanding anomalous venous conditions. Phlebolymphology 2012, 4:170–181)
Extracranial Venous Abnormalities Versus Developmental Variants
The venous system development through stages may be associated with a number of developmental variants that do not necessarily represent pathological findings. This is the reason why there is still disagreement about the physiological range of hemodynamic measurements in these veins including determination of normal or abnormal function. It has been hypothesized that these abnormalities may be related to embryologic developmental arrest, aging, or other comorbidities [2, 6, 32]. Probably, even more important is to establish what constitutes a significant narrowing of the extracranial venous system with hemodynamic consequences for the intracranial venous drainage.
So far, there have been reported a huge range of possible abnormalities in the extracranial veins draining cerebral venous blood flow. These can be classified as structural/morphological, hemodynamic/functional, and those determined only by the composite criteria and use of multimodal imaging (Table 1) [2, 9]. For example, structural/morphological venous abnormalities can be divided into those creating narrowing or occlusion and those causing abnormal distensibility. On the other hand, hemodynamic functional venous abnormalities represent an abnormal cerebral venous outflow in the presence or absence of a structural venous anomaly in the extracranial veins. Finally, because it is almost impossible to determine the prevalence of a single structural/morphologic or hemodynamic/functional venous abnormality, regardless of the imaging modality or methodology utilized, the need for use of composite criteria by uni- or multimodal imaging modalities of the extracranial venous system is emerging [23].
Diagnostic Imaging and the Diagnosis of Extracranial Venous Abnormalities
Unlike the carotid artery, the vascular wall of the IJVs is much more flexible with a variable lumen diameter which can be influenced by postural change, respiration, cardiac function, hypovolemia, and hydration status even by the pulsation of nearby arteries [2, 50, 53, 54]. The current definition (narrowing of >50 %) with respect to the proximal adjacent vein segment is mainly derived from observations in the arterial system [5, 55, 56]. Even less is known about the main drainage routes of the spine, namely, the azygos venous system and its pathophysiology. When performing imaging of the extracranial venous system, it is almost impossible to take all of the above factors into account, regardless of the imaging modality utilized. While there is common knowledge among radiologists about how to perform diagnostic imaging and intervention on the extracranial arteries, no established standardized guidelines for detection of extracranial venous abnormalities currently exist. This chapter summarizes current knowledge regarding the advantages and disadvantages of both noninvasive and invasive imaging modalities for the detection of those extracranial venous pathologies (Tables 2 and 3).
Table 2
Advantages and disadvantages of noninvasive diagnostic methods for diagnosis of extracranial venous anomalies (Adapted from Dolic et al. [2])
Noninvasive diagnostic methods | Advantages | Disadvantages |
---|---|---|
Doppler sonography | Noninvasive Without ionizating radiation Less expensive High resolution Real-time information Sensitive to detect flow changes, intra- and extraluminal abnormalities Ability to measure velocity Possible control of respiratory phases | No standardized guidelines Operator dependent Time-consuming (60–120 min) Blinding procedures are challenging Cannot perform global view of the veins (limited window) Misidentification of the veins Influenced by hydration status |
Magnetic resonance venography | Noninvasive Without ionizating radiation Well-established method Operator independent Less time-consuming than DS Provide global view of intra- and extracranial venous system Easy to blind Ability to measure flow and velocity with advanced technique (phase-contrast MRV) Global view of collateral veins Can be performed without contrast (pregnancy, allergy, etc.) | No real-time informations Cannot detect intraluminal abnormalities Low specificity of conventional MRV techniques Influenced by hydration status Azygos vein examination needs technical improvements due to important artifacts (breathing, heart movements, etc.) Underestimate the vascular caliber “Snapshot” nature |
Plethysmography | Noninvasive | Time-consuming method |
Provides valuable information regarding the impact of reflux and obstruction on overall venous function | Higher false-positive rate due to venous compression arising from incorrect patient positioning or the action of extrinsic masses | |
Can monitor the dynamics of venous disease over time and evaluation of treatment outcomes | Low resolution |
Table 3
Advantages and disadvantages of invasive diagnostic methods for diagnosis of extracranial venous abnormalities (Adapted from Dolic et al. [2])
Invasive diagnostic methods | Advantages | Disadvantages |
---|---|---|
Catheter venography | Considered the gold standard Real-time information can be obtained by using contrast Ability to measure pressure Provide “road map” for planning endovascular procedures Can be complemented by use of more sophisticated criteria (time to empty contrast from vein or wasting of the balloon) | Invasive method Ionizating radiation Cannot be performed without contrast (allergy, toxicity, etc.) Operator dependent Time-consuming (>45 min) Cannot detect intraluminal abnormalities No global view of veins and collaterals No standardized definition of significant Vein stenoses |
Intravascular ultrasound | Offers 360° view of the vessel’s wall from the inside Can detect intraluminal abnormalities Easily access all parts of IJVs in comparison with DS Provides more accurate assessment of vein stenosis and wall thickness than CV and DS | Invasive method Lack of experience – no standardize protocols Ring-down artifacts Geometric distortion – from imaging in an oblique plane Size of IVUS probe – limitation in the imaging of severe stenosis |
Noninvasive Imaging Modalities
Doppler Sonography
Description of Imaging Techniques
DS is clinically the most useful technique for detecting, localizing, and evaluating peripheral venous obstruction and venous valvular incompetence [57, 58]. The sensitivity and specificity of venous DS for symptomatic proximal deep vein thrombosis exceeds 90 % [59, 60]. Spectral analysis of the DS signal is used to confirm the presence or absence of flow and indicates its direction and the patterns. Spectral analysis of DS signal and color DS are used to confirm the presence of reflux. Several DS studies have shown a relationship between IJV drainage abnormalities, characterized by JVR and specific neurological diseases of undetermined etiology along with a higher prevalence of brain WM hyperintensities in elderly people ([8, 12, 32, 61]). An increased prevalence of JVR, dilated vessel lumen, and slowed flow velocity in the left IJV, as well as decreased time-averaged mean velocity of bilateral IJV was found in those over 70 years of age ([12, 32]).
Because of the advantages of the DS in detecting intraluminal venous pathology, it was initially promoted as a method of choice for screening of extracranial venous abnormalities and developmental variants, indicative of CCSVI [17, 62]. The diagnosis of CCSVI is both based on hemodynamic and imaging findings that utilize DS to study the deep cerebral veins, the IJVs, and the VVs in both erect and supine positions. Recent findings suggest that the majority of CCSVI pathology is confined to the intraluminal portion of extracranial veins which can be visualised with high resolution B-mode imaging while visible “stenoses” (Fig. 4) or extraluminal venous abnormalities most likely develop more frequently with the progression of the disease or age [7, 56, 63].
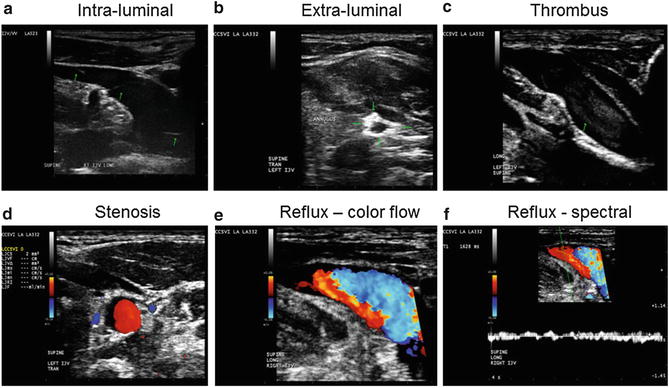
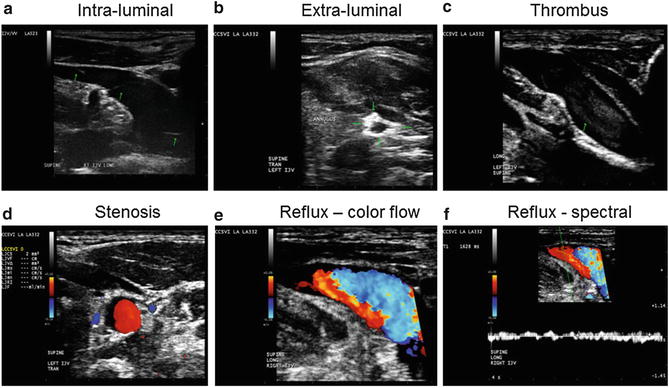
Fig. 4
Examples of chronic cerebrospinal venous insufficiency venous hemodynamic criteria on Doppler sonography. (a) Flap anomalies noted in internal jugular vein (IJV) lumen; (b) annulus in the left IJV: circumferential thickened vein wall that is restricting the vein from fully expanding with respiratory or positional changes; (c) thrombus noted in IJV; (d) severe stenosis of left IJV: CSA measurement of ≤3 mm2; (e, f) reflux/bidirectional flow directed toward the brain for a duration of >0.88 s in the right IJV in the supine position (E demonstrates reflux using color flow, while F demonstrates reflux using spectral analysis – waveform noted above baseline for greater than 0.88 s) (The figure was reproduced with permission from Dolic et al. [2])
The prevalence of CCSVI and JVR, as well as their relationship to clinical findings in CNS disorders, emphasizes the need for more quantitative and reproducible measures for the integration of morphological and functional anomalies. These include blood flow as well as velocity and blood volume measurements that could be potentially more reliable in assessing the degree of venous outflow obstruction in the IJVs and azygos vein (Fig. 5). Monti et al. performed quantitative evaluation of cerebral venous blood outflow in the supine and sitting positions and found significant alterations in cerebral venous outflow in MS patients in comparison with healthy controls and patients with other neurological diseases ([64, 65]).
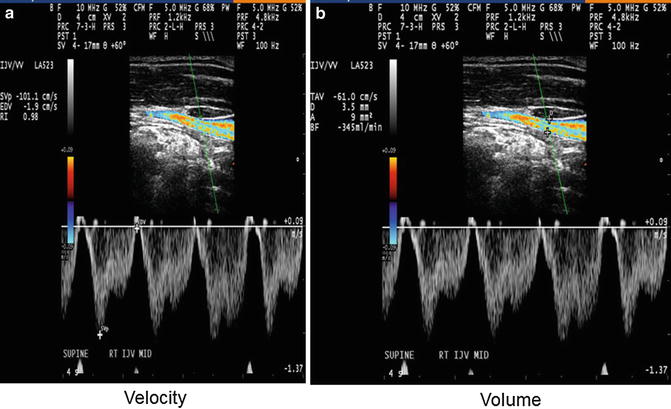
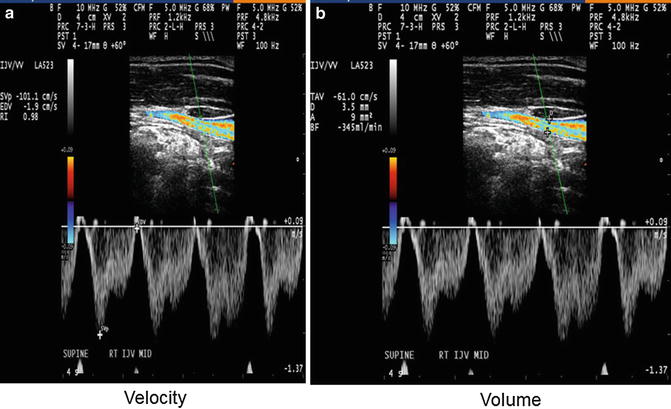
Fig. 5
Example of velocity (a) and volume (b) measurement over 4 s phase in internal jugular vein (IJV) (The figure was reproduced with permission from Dolic et al. [2])
While the value of these CCSVI VH criteria in detecting venous abnormalities or developmental variants is uncertain and operator dependent, no other validated criteria have been proposed at this time [23]. Recently, the International Society for Neurovascular Disease (ISNVD) developed a more comprehensive consensus document that included the participation of more than 60 international experts in DS imaging [23]. DS was proposed as a standardized screening tool for determining CCSVI status. The protocol proposes also the use of quantitative measures for the definition of functional anomalies such as blood flow velocity and volume (Fig. 5) that could be potentially more reliable in assessing the degree of venous outflow obstruction in the IJVs. It also refines originally proposed VH criteria. A novel technology called Quality Doppler Processing (QDP) [62, 66] designed to insonate the veins at the base of the skull may become useful in the near future, because it is difficult to visualize the superior petrosal sinus, the inferior petrosal sinus, and the contralateral inferior and superior petrosal sinuses. Because insonation of the petrosal sinuses requires a Doppler angle close to 90°, detection of reflux in the form of bidirectional flow can be made only using a multi-angle Doppler system. The QDP technology enables the operator to determine the direction of blood flow within the examined cerebral veins and make an adjustment of the pulse repetition frequency (PRF) to clearly visualize the direction without background Doppler noise. But because of its limited availability, QDP technology can only be considered as an additional criterion for diagnosis of CCSVI.
Also recently, the European Society of Neurosonology and Cerebral Hemodynamics (ESNCH) expressed considerable concerns regarding the accuracy of the proposed criteria for CCSVI in MS [19] and proposed the central blinded DS reading as part of a recent multicenter Italian CoSMo study investigating the prevalence of CCSVI in MS patients, healthy controls, and patients with other neurologic diseases (OND) [67].
Dynamic contrast-enhanced exams can potentially increase the value of DS by overcoming the operator-dependent analysis of the ultrasonic signal [68]. Unlike the diffusible agents commonly used in MR imaging and computed tomography (CT), DS contrast agents are true intravascular agents that remain entirely within the vascular space and possess intravascular characteristics similar to those of red blood cells. This technique allows registration of changes in ultrasonic signal intensity during and after intravenous injection of a contrast agent. Time dependence of enhancement can be shown in a time-intensity curve (TIC) where the average signal from a region of interest (ROI) is plotted as a function of time. Tissue and vascular uptake, transit times, and washout of the contrast agent can also be obtained from a TIC [69, 70]. Mancini et al. used contrast-enhanced ultrasonography of the internal jugular vein with TIC analysis and revealed significantly reduction of washout rate in IJVs in MS patients compared to healthy controls [68].
Advantages
DS has the advantage among other diagnostic techniques of being noninvasive, providing high-resolution images with real-time dynamic information such as flow and velocity, showing intraluminal as well as extraluminal abnormalities and developmental variants (Fig. 4), and being considerably less expensive than other noninvasive imaging techniques. DS imaging can also be readily applied in the follow-up period of subjects undergoing endovascular treatment because it can recognize the associated complications (residual stenosis, restenosis, or venous thrombosis) (Fig. 4) [2, 5, 13, 22, 71]. DS can also assess the hemodynamic consequences of outflow derangement, while B-mode ultrasound detects structural venous intraluminal anomalies (Fig. 4) [22, 71].
Disadvantages
The main criticism of the recommended DS protocol is that its reproducibility depends on the training level and skills of the operator and it is not easy to be blinded and standardized in either a research or clinical setting [7, 19, 72, 73]. Moreover, the value of the CCSVI VH criteria is controversial because they combine functional and structural intra- and extracranial venous abnormalities/developmental variants in a single binary composite. Further, the assessment of the second CCSVI criterion (reflux in deep cerebral veins) (Fig. 6) is particularly controversial because the direction of the blood flow in veins connecting cortical with deep veins may vary considerably as a consequence of the physiologic interindividual variation of the cerebral venous anatomy [72].
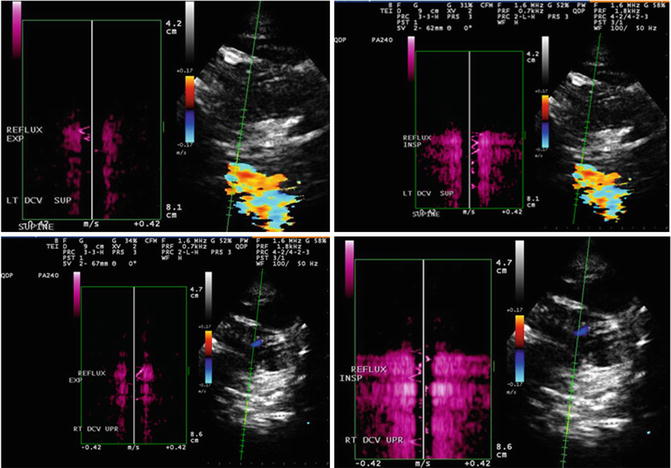
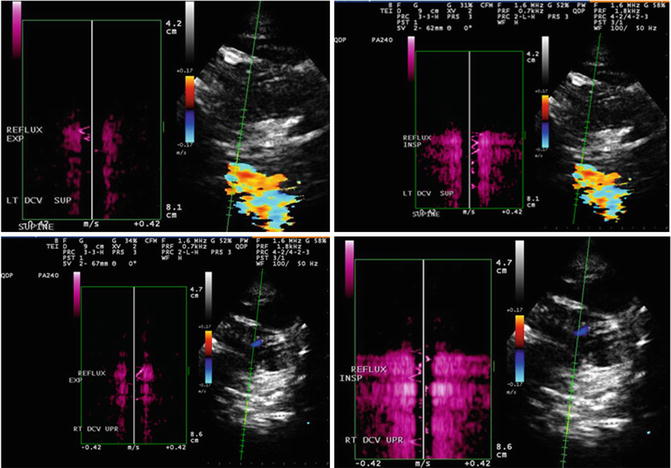
Fig. 6
Example of reflux in the deep cerebral veins using Quality Doppler Profile (QDP). Doppler profile on opposite sides of baseline (The figure was reproduced with permission from Dolic et al. [2])
DS also has limits regarding extracranial vein characterization, since findings can be influenced by hydration status. It is a very time-consuming method, and visualization of the central veins, particularly in the thorax and abdomen, is often limited and cannot give the global view of vein anatomy. Although it can detect extracranial collateral veins which are probably associated with CCSVI, it is not technically feasible to follow the complete course of the collateral veins which can be more easily visualized with the use of magnetic resonance venography (MRV), computed tomography venography (CTV), or CV. Other pitfalls in DS imaging include the misidentification of veins. Additionally, overlying bone and muscle may prevent continuous imaging (cannot visualize suitably the confluence of the IJVs and the subclavian vein because the clavicle commonly blocks direct visualization). Similarly, the cervical part of IJV and the jugular bulb cannot be visualized by DS because of the limited acoustic window resulting from the spine, mandible, and skull. So far, none of the recently published DS studies have reproduced the originally reported CCSVI prevalence, regardless of diagnostic DS method utilized [5, 48, 71, 74, 75].
Magnetic Resonance Venography
Description of Imaging Techniques
During the past decade, catheter-based digital subtraction angiography, as the preferred method for imaging of the intracranial venous anatomy, has been increasingly supplanted by MRV, an often overlooked and underappreciated noninvasive and safe method for the evaluation of head and neck veins [76, 77]. In the absence of better noninvasive techniques for the imaging of the dural venous sinuses, well-known and documented pitfalls associated with flow-sensitive MR techniques have been tolerated [34, 56, 78–81]. Furthermore, simple protocols that incorporate two-dimensional time-of-flight (2D-TOF) pulse sequence have already improved their accuracy for the diagnosis of deep venous thrombosis involving the femoral, popliteal, or iliac veins [82]; however, experience with MRV techniques in the cervical veins remains still limited.
The CCSVI concept triggered several MRV studies that emphasized the value of MRV as a comprehensive, noninvasive, and relatively operator-independent technique which provided a 3D structural assessment of the intra- and extracranial vasculature for the potential identification of stenosis and quantification of blood flow through major veins [7, 48, 56, 78, 79, 81, 83–90]. But so far almost none of the MRV studies found significant differences in extracranial vein morphology between MS patients and controls. It could be hypothesized that those so-called abnormalities in venous outflow likely reflect normal developmental anatomic variants. Further, the findings of these studies suggest also that MRV morphologic information by itself may be insufficient to allow conclusions to be drawn about the presence of venous abnormalities and their relationship to CCSVI in MS.
Time of Flight
TOF venography has the advantage of simplicity because no special pulse sequences are required, and this technique is available on nearly every MRI system. TOF pulse sequences are spoiled gradient-echo or gradient-echo acquisitions performed sequentially, i.e., all “phase-encode” steps are played out in a single slice before moving on to the next slice, which results in much greater suppression of stationary tissue [34, 77, 82]. It also has the advantage of avoiding the need for the use of contrast agents, and it remains the technique of choice in the evaluation of the pregnant patient with suspected dural sinus thrombosis [34, 78]. Furthermore, the accompanying conventional MR study is more sensitive in terms of the detection of cortical venous infarction than a CT [91]. The axial orientation of the acquisition allows for high in-plane resolution, which is ideal for cross-sectional area (CSA) measurements of the veins (Fig. 7). However, the TOF sequence is easily affected by motion artifacts, especially from the patient’s breathing, swallowing, snoring, or head motion [87, 92]. Relative insensitivity to in-plane flow is another limitation of the TOF technique. Regarding the direction of flow, the optimal acquisition plane lies orthogonal, which is inefficient from the standpoint of acquisition time and not always achievable. Although it has a higher spatial resolution, 2D TOF may overestimate stenosis in the setting of turbulent or slow flow [78]. All in all, standard conventional MRV techniques are more prone to artifacts than phase-contrast MRV and multidirectional TOF angiography [34, 56]. These techniques can potentially alleviate some of the usual MRV artifacts and provide more detailed flow information. One obvious improvement is to image at higher field strength, such as 3 T, because this increases signal-to-noise ratio and better characterizes slow flow.
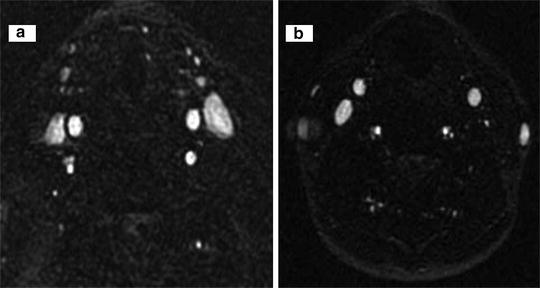
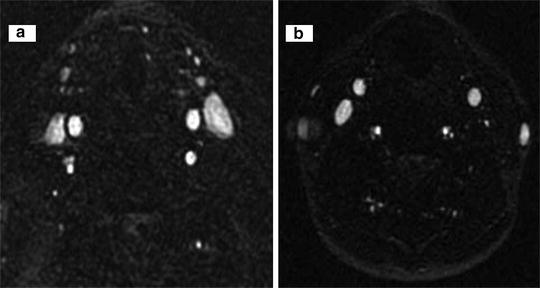
Fig. 7
Example of normal and abnormal flow in internal jugular vein on magnetic resonance venography. Normal flow in both internal jugular veins (a) and abnormal flow in left internal jugular vein on axial 2D time of flight (b). The figure was reproduced with permission from Dolic et al. [2]
Phase-Contrast Imaging
In contrast to TOF techniques, which rely mainly on flow-related enhancement for producing vascular images, phase-contrast MR angiography (PC-MRA) uses velocity-induced phase shifts imparted upon the moving spins to distinguish flowing blood from the surrounding stationary tissue, thus providing information regarding both anatomy and flow (Fig. 8). The major advantage of PC-MRI angiography is excellent background suppression as well as quantitative determination of blood velocities [93, 94]. However, it requires long imaging times and a prior estimate of blood flow velocity. Furthermore, it may also be more sensitive to signal loss due to turbulence or intravoxel dephasing. So far there are only a few studies that used PC-MRI to quantify venous flow in MS patients compared to healthy controls [92, 95, 96]. More studies are needed to validate the venous flow at the upper neck level on an adequate number of age- and gender-matched healthy controls with heterogeneous age groups.
